|
|
|
ABSTRACT |
The accurate and reliable assessment of physical activity is necessary for any research study where physical activity is either an outcome measure or an intervention. The aim of this review is to examine the use of objective measurement techniques for the assessment and interpretation of children’s physical activity. Accurate measurement of children’s activity is challenging, as the activity is characteristically sporadic and intermittent, consisting of frequent, short bouts. Objective measures of physical activity include heart rate telemetry, pedometry and accelerometry, and each of these methods has strengths and limitations. Heart rate is suited to the measurement of sustained periods of moderate and vigorous activity, pedometry provides a valid measure of total activity, and accelerometry provides a valid measure of total activity as well as the pattern and intensity of activity. As the weaknesses of heart rate and accelerometry for the assessment of activity are not inter-correlated, a combination of the two methods may be more accurate than either method alone. Recent evidence suggests that the Actiheart, an integrated accelerometer and heart rate unit, provides a more accurate prediction of children’s energy expenditure than either heart rate or accelerometry alone. However, the cost of the Actiheart is prohibitive for large-scale studies. The pedometer is recommended when only the total amount of physical activity is of interest. When the intensity or the pattern of activity is of interest, accelerometry is the recommended measurement tool. |
Key words:
Activity pattern, heart rate, pedometry, accelerometry
|
Key
Points
- The use of objective measures to assess physical activity in children is recommended.
- Pedometers provide an inexpensive objective measure of total activity that is highly correlated with more sophisticated techniques, e.g. accelerometry, and has been used to identify relationships between health and activity in children.
- Accelerometry allows examination of the temporal pattern and intensity of children’s activity, including sporadic physical activity and bouts of physical activity.
|
The accurate and reliable assessment of physical activity is necessary for any research study where physical activity is either an outcome measure or an intervention. However, physical activity is notoriously difficult to measure and these difficulties are particularly exacerbated when assessing activity in children. Numerous methods exist for the measurement of physical activity. Broadly, the various techniques can be grouped as self-report, observation, heart rate telemetry and motion sensors. Pragmatic considerations often lead to self-report as the tool of choice, particularly in large-scale epidemiological studies (Freedson et al., 2005). However, the sporadic short-burst nature of children’s activity (Bailey at al., 1995; Baquet et al., 2007; Berman et al., 1998) makes it particularly difficult to capture data via self-report methods. The problem is compounded, as the concept of time and the ability to accurately recall are limited by the child’s level of cognition and the emotion associated with the activity (Gleitman, 1996). Developments in technology over the past twenty years have resulted in an increase in the use of objective methods to assess habitual physical activity. During the 1990’s, heart rate monitoring was extensively used by Armstrong and colleagues (e.g. Armstrong et al., 1990) and was the preferred method of choice, but over the last ten years pedometry and accelerometry (e. g. Rowlands et al., 1999; Trost et al., 2002) have increased in popularity. The purpose of this review is to examine the use of objective measurement techniques for the assessment and interpretation of children’s physical activity.
The pattern of activityBailey et al., 1995 highlighted the transitory nature of children’s physical activity using a very comprehensive observation protocol with fifteen American 6-10 year-old children. This study recorded the frequency, duration and varying intervals between activities of different intensity and duration (tempo). The protocol was very labour-intensive; observation periods were four hours in duration and observers were cued every three seconds to record activity by an audible bleep from a microcassette recorder earphone. The median duration of low and medium intensity activities was found to be a mere 6 s, and for high intensity activities it was only 3 s. Re-analysis of the same data set with spectral analysis showed 83 ± 11 bouts of activity per hour in boys and 89 ± 12 bouts per hour in girls and revealed the mean bout duration was 21 ± 5 s in boys and 20 ± 4 s in girls (Berman et al., 1998). More recently, researchers have used high-frequency accelerometry monitoring to assess the temporal pattern of children’s activity. Despite the different methodologies, results are very similar to those reported from the observation protocols. Baquet et al., 2007 reported a mean bout duration of 22.1 ± 3.5 s in French 8-10 year-olds and showed that 80, 93 and 96% of activity bouts of moderate, vigorous and very high intensity, respectively, were shorter than 10 s. Although the proportion of time spent in vigorous and very high intensity activity was low (<3%), it accounted for over a third of the total physical activity (Baquet et al., 2007). This highlights the importance of accurately quantifying these short bouts of intense activity. Many methods of activity measurement are fairly insensitive to these short bouts. The lack of appreciation of and quantification of the transitory nature of the activity patterns in children may have affected the ability of studies to provide an accurate reflection of children’s activity and this will have impacted on the determination of associations between activity and health.
Assessment methods Heart rateHeart rate is not a direct measure of physical activity. However, it does provide an indication of the relative stress placed upon the cardiopulmonary system by physical activity (Armstrong, 1998). Heart rate monitoring also allows the recording of values over time, which facilitates a visual assessment of the pattern and intensity of activity. It was the first widely used objective measure of physical activity in children. In the South West of England alone, over a ten year period Neil Armstrong and colleagues monitored the heart rates of over 1200 5-16 year-old children over three schooldays (Armstrong et al., 1990; 2000; Armstrong and Bray, 1990; 1991; Biddle et al., 1991; McManus and Armstrong, 1995; Welsman and Armstrong, 1997; 1998; 2000). However, there are a number of limitations to the use of heart rate monitoring for assessing physical activity (Armstrong and Welsman, 2006; Rowlands, 2001; Rowlands et al., 1997). Physical activity is not the only factor that causes changes in heart rate. Heart rate can also be influenced by other parameters, e.g. emotional stress, anxiety, level of fitness, type of muscular contraction, active muscle group, hydration and environment (Armstrong and Welsman, 2006; Rowlands et al., 1997). These factors can have the greatest influence at low activity intensity; hence Riddoch and Boreham, 1995 recommended that heart rate monitoring should be considered primarily as a tool for the assessment of moderate to vigorous activity and that heart rates below 120 bpm would not normally be considered to be valid estimates of physical activity. The methods of analysing heart rate activity data are numerous with over 24 different methods of analysing heart rate data identified by Harro and Riddoch, 2000, This diversity in methods of analysis limits the comparability between studies. However, most studies predict energy expenditure from individual or group regression equations, report the time spent above pre-determined heart rate thresholds or report net heart rate (heart rate minus resting heart rate). To account for the problems associated with prediction of energy expenditure from low heart rates, investigators have used a ‘flex’ heart rate approach, whereby heart rates below a pre-determined threshold are considered to equate to resting energy expenditure and an individual heart rate: energy expenditure regression equation is used to predict energy expenditure above this threshold (Livingstone et al., 1992). However, the labour intensive nature of individual calibration limits the use of this technique (Armstrong and Welsman, 2006) and the majority of studies report time spent above heart rate thresholds equivalent to moderate and vigorous activity. The method chosen for the analysis of heart rate data needs careful consideration as it can affect the interpretation of the data and hence conclusions regarding habitual activity status and associations with health variables. For example, Janz et al., 1992 reported a negative relationship between children’s activity and fatness when expressing heart rate as net heart rate, whereas when expressing the same heart rate data as time spent above intensity thresholds the relationship was weaker and non-significant. A meta-analysis of fifty studies investigating the relationship between activity and body fat in children and youth showed that the average effect size elicited from studies using heart rate to assess activity levels, most of which used time spent above moderate and vigorous thresholds, was significantly lower than the average effect size elicited from studies using a different method of activity assessment (questionnaire, observation, motion counter) (Rowlands et al., 2000). It is possible that increased body fatness increases the cardiovascular stress, and hence heart rate, during normal activities (Rowlands et al., 1999). Heart rate monitors are generally set to store heart rate values every minute. However, as suggested by Armstrong and Welsman, 2006, in order to capture the rapid short bursts of activity characteristic of children’s activity, a smaller sampling interval would be optimal. As the heart rate response tends to lag behind changes in movement (Rowlands et al., 1997) and there is a rapid transition between activities associated with children’s behaviour (Bailey et al., 1995), it is unlikely the heart rate response would be able to provide a comprehensive picture of the temporal pattern of activity typical of children. Despite the above limitations, heart rate monitoring has provided a valid and reliable objective estimate of physical activity, particularly sustained periods of moderate and vigorous activity, and the vast quantity of data collected has given researchers valuable, objective insights into the nature of children’s activity over the past twenty years.
PedometryLeonardo da Vinci designed the pedometer approximately 500 years ago (Gibbs-Smith, 1978); it is a simple mechanical motion sensor that records the acceleration and deceleration of movement in one direction. Generally, the pedometer gives a measure of total activity, or movements, over the time period assessed, although more sophisticated models are available. The well-documented disadvantages of this method include the pedometer’s inability to measure intensity, record counts during cycling and record increases in energy expenditure due to carrying objects or walking/running uphill (Rowlands, 2001; Rowlands et al., 1997). Early studies using mechanical pedometers concluded that they were inaccurate at counting steps or measuring distance walked (Gayle et al., 1977; Kemper and Verschuur, 1977; Saris and Binkhorst, 1977; Washburn et al., 1980). However, during the last ten to fifteen years, studies have provided evidence for the reliability and validity of electronic pedometers for the quantification of distance walked, number of steps taken (Bassett et al., 1996), assessment of total daily activity (Sequeira et al., 1995) and estimation of activity intensity and duration (Tudor-Locke et al., 2005; Rowlands and Eston, 2005). Reliability and validity do differ by brand, hence it is important to consult some of the comparative studies (e.g. Schneider et al., 2004; Tudor-Locke et al., 2006) and test the accuracy of the pedometers with the population of interest before commencing a study. Kilanowski et al., 1999 investigated the validity of pedometry as a measure of daily activity of 10-12 year-old children using contemporaneous measures of pedometry (Yamax Digi-walker SW-200, Yamasa, Tokyo, Japan), triaxial accelerometry (Tritrac Professional Products, Reining International, Madison, WI, USA) and observation. Pedometer counts correlated significantly with both observation and triaxial accelerometry counts during high intensity and low intensity recreational activities. In the same year, a study from our laboratory showed that activity measured by pedometry or the Tritrac triaxial accelerometer, correlated positively with fitness (Tritrac r = 0.66; Pedometer = 0.59, p < 0.01) and negatively with fatness (Tritrac r = -0.42; Pedometer = -0.42 p < 0.05) in 34 boys and girls, aged 8-10 years (Rowlands et al., 1999). It is notable that the simple pedometer identified the same relationships with fitness and fatness as the relatively sophisticated Tritrac. In contrast, contemporaneous measures of time spent above moderate and vigorous heart rate thresholds were not related to body fatness. Over the last ten years, there has been a growth in the number of studies that have used pedometry to assess physical activity in children. The method is objective, cheap, unobtrusive and ideal for large population surveys, or any situation where only a measure of total activity and not activity pattern is required. Recent studies have shown positive relationships between children’s daily step counts and aerobic fitness (Le Masurier and Corbin, 2006), bone density (Rowlands et al., 2002), psychological well-being (Parfitt and Eston, 2005) and negative relationships with body fatness (Duncan et al., 2006). There is a possibility that the act of wearing any activity monitor will cause a child to engage in reactive behaviour. This is defined as “a change in normal activity levels because of the participants’ knowledge that their activity levels are being monitored ”(Welk et al., 2000, p.59). The likelihood of reactive behaviour is potentially greater when activity is assessed using pedometers as children may be aware of their pedometer scores and/or able to check their score throughout the course of the day. This has led many researchers to ‘blind’ children to their scores by sealing the pedometers. The output can then be collated in a number of different ways. At the most controlled level, researchers may visit school each day and take pedometer scores from each child, re-sealing the pedometer after it has been read. However, this is a problem at weekends, so some researchers have provided one pedometer for each day of the measurement (well marked) and the child simply wears the appropriate pedometer each day and hands all the pedometers in at the end of the study. Alternatively, protocols may require parents/guardians to read the pedometer scores and re-seal the pedometer after the child has gone to bed. Other protocols make no attempt to blind the child to the pedometer output. Research has indicated little evidence for reactive behaviours whether the child is blinded to the pedometer output (Vincent and Pangrazi, 2002) or not (Ozdoba et al., 2004). We have assessed the difference between sealed and unsealed pedometers worn simultaneously by 9-11 year-old children and found no consistent discrepancy between the pedometers (unpublished data). It appears that valid measures of daily habitual activity can be obtained from sealed and unsealed pedometers, however researchers may wish to seal pedometers during the day to minimise the risk of the pedometer accidentally being re-set and the loss of the days’ data. The pedometer also holds promise as a motivational tool to self-regulate physical activity levels. Pedometer-based intervention studies have demonstrated increased steps/day using set or individualised goals in adults (e.g. Chan et al., 2004; Tudor-Locke et al., 2004). Studies with children show that rewards based on access to television viewing combined with pedometer-based goals are effective in increasing children’s activity levels (Goldfield et al., 2000; Roemmich et al., 2004), but that pedometer-based goals alone, without the rewards, are not as effective (Goldfield et al., 2006). We have shown that a peer-modelling, rewards (small customised toys, e.g. balls and frisbees) and pedometer-feedback intervention was successful in increasing physical activity in 9-11 year-old children (unpublished data). Therefore, evidence exists for the use of a pedometer not only as a measurement tool, but also as an intervention tool for behaviour change.
AccelerometrySince 2001, there has been a dramatic increase in the number of studies using accelerometers to assess physical activity in children (Rowlands, 2007). Like pedometry, accelerometry is objective and measures movement directly, which is an important factor when assessing the relationship between health and activity. Critically, accelerometers also have a time-sampling capability allowing the assessment of the temporal pattern and intensity of activity as well as total accumulated activity. However, there is a lack of standardisation regarding how accelerometers are used, which outcome measures are used and how the output is interpreted. This limits comparability between studies and the accumulation of knowledge relating to children’s activity. At the end of 2004, experts in accelerometry presented at the conference on ‘Objective Monitoring of Physical Activity: Closing the Gaps in the Science of Accelerometry’ held at the University of North Carolina, USA. Medicine and Science in Sports and Exercise subsequently published a special issue (November, 2005) containing the papers from this conference. This collection of papers provides an excellent, thorough analysis of the accelerometer literature and the areas where there is no clear consensus and where further research is required. Some of the current issues regarding the use of accelerometry are discussed below: choice of accelerometer, frequency of data collection (time sampling interval or epoch) and translating accelerometer output into meaningful units. Accelerometers measure acceleration in one to three orthogonal planes (vertical, mediolateral and anteroposterior). Uniaxial accelerometers are normally worn so the sensitive axis is oriented in the vertical plane. Omnidirectional accelerometers are most sensitive in the vertical plane, but are also sensitive to movement in other directions with the output being a composite of the signals (Chen and Bassett, 2005). In contrast, triaxial accelerometers consist of three orthogonal accelerometer units and provide an output for each plane as well as a composite measure. The commercially available accelerometers most frequently referred to in the literature are the uniaxial ActiGraph (ActiGraph, Fort Walton Beach, FL, which has also been referred to as the CSA, the MTI and the WAM), the omnidirectional Actical (Mini Mitter Co., Inc., Bend OR) and Actiwatch (Mini Mitter Co., Inc., Bend, OR), and the triaxial RT3 (Stayhealthy, Inc., Monrovia, CA), which superceded the Tritrac. Evidence suggests that triaxial accelerometers may provide a more valid estimate of children’s physical activity than uniaxial accelerometers (Eston et al., 1998; Louie et al., 1999; Ott et al., 2000; Welk, 2005). However, the difference appears to be small and correlations between uniaxial and triaxial output are high indicating that they are providing similar information (Trost et al., 2005). More recent evidence in adults and children has indicated that uniaxial accelerometry plateaus or even begins to decline at running speeds greater than 10 km.h-1 (Brage et al., 2003a; 2003b; Rowlands et al., 2007). This is largely due to the dominance of horizontal acceleration at moderate to high running speed, rather than vertical acceleration. The incorporation of three vectors in triaxial accelerometry accounts for the variance in the relative dominance of the vectors across the different speeds. The relevance of this to the assessment of children’s habitual activity, where short bursts of high intensity activity are common (Bailey et al., 1995), is yet to be investigated. The signal from an accelerometer is integrated over a given time interval, or epoch, then summed and stored. Depending on the accelerometer model, the epoch can be set as low as 1 s or as high as several minutes. In the past, the vast majority of studies have set the epoch at 1 min, although this is known to underestimate vigorous and high intensity activity (Nilsson et al., 2002; Rowlands et al., 2006). As appreciation of the sporadic nature of children’s activity has increased, studies have begun to use 10 s epochs (e.g. Hasselstrom et al., 2007). The arbitrary selection of a 1 min epoch has most likely been due to the memory size of accelerometers. For example, the ActiGraph (model 7164) and triaxial RT3 are capable of collecting data at 1 s epochs for a maximum of only nine hours. If output from each of the three vectors of the RT3 is required, as well as the composite vector magnitude, the recording time is reduced to three hours. However, the latest version of the ActiGraph (GT1M) has a memory size of 1 Mb and can collect data at 1 s epochs for nearly six days. This makes it feasible to use epochs ranging from 1 to 15 s to objectively assess the temporal pattern of children’s activity over days at a time with the uniaxial ActiGraph. It should be noted that, at present, the RT3 can only be used in the 1 s mode for nine hours at a time. Further research should address whether short bursts of high intensity activity are underestimated by uniaxial accelerometry, as is the case for fast running. Baquet et al., 2007 and Chu et al., 2005 utilised high-frequency accelerometry monitoring with the ActiGraph and the RT3, respectively, to assess the pattern of children’s activity. Physical activity patterns were very similar to those from the earlier observation study (Bailey et al., 1995). Furthermore, Chu et al. demonstrated that the intensity of activity bouts was positively related to fitness (r > 0.4, p < 0.05) and the interval between bouts was positively related to fatness (r > 0.6, p < 0.01) in 24 nine year-old Hong Kong Chinese children. However, the duration of the bouts was not related to fitness or fatness. This novel study highlights the potential importance of activity pattern. Further studies should investigate whether temporal aspects of the activity pattern explain variance in health and fitness in addition to that explained by composite variables (e.g. total activity, total time spent in moderate to vigorous activity). For example, research has shown that total time accumulated in vigorous physical activity is related to fatness in children, aged 4 - 6 years (Janz et al., 2002), 5-11 years (Abbott and Davies, 2004), 8-11 years (Ekelund et al., 2004; Rowlands et al., 1999; Rowlands et al., 2006) and adolescents (Gutin et al., 2005). To what extent does the combination of frequency, intensity and duration of activity bouts matter, if the overall activity is the same? The output from accelerometers is a dimensionless unit commonly referred to as ‘accelerometer counts’. These counts are arbitrary, depending on the specifications of the accelerometer, and therefore cannot be compared between different types of accelerometer (Chen and Bassett, 2005). In order to give biological meaning to the output, these counts have been calibrated with energy expenditure (Freedson et al., 2005). As a result, count thresholds relating to various categories of energy expenditure (including sedentary behaviour) have been published that allow researchers to calculate the amount of time spent at differing intensities of activity for the ActiGraph (e.g. Freedson et al., 1997, Puyau et al., 2002, Treuth et al., 2004, Trost et al., 2002), the Actical (Heil, 2006, Puyau et al., 2004), the Actiwatch (Puyau et al., 2004), the Tritrac (McMurray et al., 2004, Rowlands et al., 1999) and the RT3 (Rowlands et al., 2004). Freedson et al., 2005 has provided a thorough discussion of the development of these thresholds. The number of thresholds available highlights the lack of agreement regarding interpretation of accelerometer output and highlights an ongoing problem with accelerometer research and comparability between studies. Calibration studies tend to take place in the laboratory environment due to the difficulty of using a criterion measure of energy expenditure in the field. Some studies focus on walking/running activities (Freedson et al., 1997; Trost et al., 1998), while other studies incorporate ‘free play’ activities (Eston et al., 1998; Pfeiffer et al., 2006, Puyau et al., 2002; 2004; Rowlands et al., 2004) into the calibration. Knowledge of the activities used to develop cut-points is important as the activities used to develop the accelerometer threshold counts have a major impact on the thresholds developed. For example, Eisenmann et al. (2004) demonstrated that use of a treadmill-based prediction equation (Trost et al., 1998) to estimate energy expenditure from the ActiGraph underestimated the energy cost of self-paced sweeping, bowling and basketball in 11 year-old boys and girls. However, activities were correctly classified as light or moderate at a group level according to thresholds based on structured activities (Puyau et al., 2002). Despite the errors apparent when predicting energy expenditure from accelerometer counts, accelerometer counts are generally reported to be moderately to highly correlated with energy expenditure, assessed via a criterion method, across a range of activities. Additionally, accuracy is fair to excellent for the classification of the intensity of an activity as light, moderate or vigorous. This may be sufficient for some research questions. Currently, research is addressing methods of analysing accelerometer data that will allow the mode of activity to be identified and the intensity to be classified once the mode is known (e.g Crouter et al., 2006; Pober et al., 2006). This would not only improve the accuracy of the estimation of intensity, but also add some degree of qualitative information regarding activity patterns that accelerometers have always lacked.
Multiple methodsPhysical activity is a complex behaviour and there are limitations associated with all the measurement methods described above. The limitations associated with heart rate monitoring are mainly due to biological variance, whereas the limitations associated with accelerometry are largely biomechanical (Brage et al., 2004). As the errors associated with the two techniques are independent, a combination of the two methods may provide a more accurate estimate of physical activity than either method alone. The Actiheart (Cambridge Neurotechnology, Papworth, UK) is a small (10 g) heart rate recorder with an integrated omnidirectional accelerometer. It is clipped onto two ECG electrodes worn on the chest. Corder et al., 2005 have reported a greater accuracy in the prediction of children’s energy expenditure during treadmill walking and running than either accelerometry or heart rate alone. At present, the cost of the Actiheart prohibits its use in all but small-scale studies. However, the Actiheart could provide a valid criterion measure of physical activity for use in the field.
In summary, children’s physical activity is characterised by frequent, short bursts of activity. This pattern has been identified using observation and high-frequency accelerometry monitoring techniques. Due to the nature of children’s activity and children’s limited ability for recall, objective techniques are recommended for the assessment of their physical activity. Heart rate monitoring was the first widely used objective measure of children’s activity. However, whilst it accurately captures sustained bouts of moderate and vigorous activity, it is not suited to the capture of low intensity activity or rapidly changing activity. Pedometers provide an inexpensive valid and reliable assessment of total activity, but do not provide any information regarding the pattern or intensity of activity. Total daily steps undertaken by children have been shown to relate with various aspects of health. Additionally, the pedometer shows promise as a motivational tool for increasing activity as well as a measurement tool. Accelerometry provides a valid and reliable assessment of the pattern of activity, as well as total physical activity, and its use has increased disproportionately since 2001. No single measure is without limitations and the Actiheart, which combines accelerometry and heart rate in one unit, is reported to provide a more accurate estimate of children’s energy expenditure than either measure alone. While the cost of this unit is prohibitive for large- scale studies, it could provide the ideal criterion measure for validation of other measures in the field.
|
AUTHOR BIOGRAPHY |
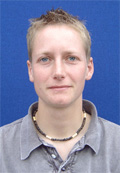 |
Ann V. Rowlands |
Employment: Research Fellow, School of Sport and Health Sciences, University of Exeter. |
Degree: BSc (HONS), PhD |
Research interests: Measurement of physical activity, relationship between physical activity and health, biological basis for physical activity. |
E-mail: a.v.rowlands@ex.ac.uk |
|
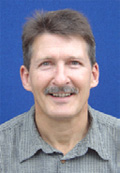 |
Roger G. Eston |
Employment: Professor and Head of School, School of Sport and Health Sciences, University of Exeter. |
Degree: PhD |
Research interests: Perceived exertion, physical activity and health, body composition, exercise-induced muscle damage. |
E-mail: r.g.eston@ex.ac.uk |
|
|
|
REFERENCES |
 Abbott R.A., Davies P.S. (2004) Habitual physical activity and physical activity intensity: their relation to body composition in 5.0-10.5-y-old children. European Journal of Clinical Nutrition 58, 285-291.
|
 Armstrong N. (1998) Young people’s physical activity patterns as assessed by heart rate. Journal of Sports Sciences 16, S9-S16.
|
 Armstrong N., Balding J., Gentle P, Kirby B. (1990) Patterns of physical activity among 11-16 year old British children. British Medical Journa 301, 203-205.
|
 Armstrong N., Bray S. (1990) Primary schoolchildren’s physical activity patterns during autumn and summer. Bulletin of Physical Education 26, 23-26.
|
 Armstrong N., Bray S. (1991) Physical activity patterns defined by continuous heart rate monitoring. Archives of Disease in Childhood 66, 245-247.
|
 Armstrong N., Welsman J.R. (2006) The physical activity patterns of European youth with reference to methods of assessment. Sports Medicine 36, 1067-1086.
|
 Armstrong N., Welsman J.R., Kirby B.J.. (2000) Longitudinal changes in 11-13-year-olds’ physical activity. Acta Paediatrica 89, 775-780.
|
 Bailey R.C., Olson J., Pepper S.L., Porszasz J., Barstow T.J., Cooper D.M. (1995) The level and tempo of children’s physical activities: an observational study. Medicine and Science in Sports and Exercise 27, 1033-1041.
|
 Baquet G., Stratton G., Van Praagh E., Berthoin S. (2007) Improving physical activity assessment in children with high-frequency accelerometry monitoring: a methodological issue. Preventive Medicine 44, 143-147.
|
 Bassett D.R., Ainsworth B.E., Leggett S.R., Matian C.A., Main J.A., Hunter D.C., Dunacan G.E. (1996) Accuracy of five electronic pedometers for measuring distance walked. Medicine and Science in Sports and Exercise 28, 1071-1077.
|
 Berman N., Bailey R., Barstow T.J., Cooper D.M. (1998) Spectral and bout detection analysis of physical activity patterns in healthy, prepubertal boys and girls. American Journal of Human Biology 10, 289-297.
|
 Biddle S., Mitchell J., Armstrong N. (1991) The assessment of physical activity in children: a comparison of continuous heart rate monitoring, self-report and interview techniques. British Journal of Physical Education Research 10, 4-8.
|
 Brage S. Brage, N. Franks P.W., Ekelund U. Wong, M. Anderson L.B., Froberg K., Wareham N.J. (2004) Branched equation modelling of simultaneous accelerometry and heart rate monitoring improves estimate of directly measured physical activity energy expenditure. Journal of Applied Physiology 96, 343-351.
|
 Brage S., Wedderkopp N., Anderson L.B., Froberg K. (2003a) Influence of step frequency on movement intensity predictions with the CSA accelerometer: A field validation study in children. Pediatric Exercise Science 15, 277-287.
|
 Brage S., Wedderkopp N., Franks P.W., Anderson L.B., Froberg K. (2003b) Reexamination of validity and reliability of the CSA monitor in walking and running. Medicine and Science in Sports and Exercise 35, 1447-1454.
|
 Chan C.B., Ryan D.A.J., Tudor-Locke C. (2004) Health benefits of a pedometer-based physical activity intervention in sedentary workers. Preventive Medicine 39, 1215-1222.
|
 Chen K.Y., Bassett D.R. (2005) The technology of accelerometry-based activity monitors: Current and future. Medicine and Science in Sports and Exercise 37, S490-S500.
|
 Chu E.Y.W., Hu Y., Tsang A.M.C., McManus A.M. (2005) The influence of the distinguished pattern of locomotion to fitness and fatness in prepubertal children. Children and Exercise XXII , -.
|
 Corder K, Brage S., Wareham N.J., Ekelund U.. (2005) Comparison of PAEE from combined and separate heart rate and movement models in children. Medicine and Science in Sports and Exercise 37, 1761-1767.
|
 Crouter S.E., Clowers K.G., Bassett Jr D.R. (2006) A novel method for using accelerometer data to predict energy expenditure. Journal of Applied Physiology 100, 1324-1331.
|
 Duncan J.S., Schofield G., Duncan E.K. (2006) Pedometer-determined physical activity and body composition in New Zealand children. Medicine and Science in Sports and Exercise 38, 1402-1409.
|
 Eisennman J.C., Strath S.J., Shadrick D., Rigsby P., Hirsch N., Jacobson L. (2004) Validity of uniaxial accelerometry during activities of daily living in children. European Journal of Applied Physiology 91, 259-263.
|
 Ekelund U., Sardinha L.B., Anderssen S.A., Harro M., Franks P.W., Brage S., Cooper A.R., Anderson L.B., Riddoch C., Froberg K. (2004) Associations between objectively assessed physical activity and indicators of body fatness in 9- to 10-y-old European children: a population-based study from 4 distinct regions in Europe (the European Youth Heart Study). American Journal of Clinical Nutrition 80, 584-590.
|
 Eston R.G., Rowlands A.V., Ingledew D.K. (1998) Validity of heart rate, pedometry and accelerometry for predicting the energy cost of children’s activities. Journal of Applied Physiology 84, 362-371.
|
 Freedson P.S., Melanson E., Sirad J. (1997) Calibration of the Computer Science and Applications, Inc. accelerometer. Medicine and Science in Sports and Exercise 29, S45-.
|
 Freedson P.S., Pober D., Janz K.F. (2005) Calibration of accelerometer output for children. Medicine and Science in Sports and Exercise 37, S523-S530.
|
 Gayle R., Montoye H.J., Philpot J. (1977) Accuracy of pedometers for measuring distance walked. Research Quarterly for Exercise and Sport 48, 632-636.
|
 Gibbs-Smith C.. (1978) The Inventions of Leonardo da Vinci. London. Phaidon Press Ltd. 31-43.
|
 Gleitman H.. (1996) Basic Psychology. New York. Norton and Company.
|
 Goldfield G.S., Kalakanis L.E., Ernst M.M., Epstein L.H. (2000) Open-loop feedback to increase physical activity in obese children. International Journal of Obesity and Related Metabolic Disorders 24, 888-892.
|
 Goldfield G.S., Mallory R., Parker T., Cunningham T., Legg C., Lumb A., Parker K., Prud'homme D., Gaboury I, Adamo K.B. (2006) Effects of open-loop feedback on physical activity and television viewing in overweight and obese children: a randomized, controlled trial. Pediatrics 118, e157-166.
|
 Gutin B., Yin Z., Humphries M.C., Barbeau P. (2005) Relations of moderate and vigorous physical activity to fitness and fatness in adolescents. American Journal of Clinical Nutrition 81, 746-750.
|
 Harro M., Riddoch C., Armstrong N. (2000) Paediatric Exercise science and Medicine. Physical activity. and van Mechelen, W. Oxford. Oxford University Press.
|
 Hasselstrøm H., Karlsson K.M., Hansen S.E., Grønfeldt V., Froberg K., Andersen L.B. (2007) Peripheral Bone Mineral Density and Different Intensities of Physical Activity in Children 6-8 Years Old: The Copenhagen School Child Intervention Study. Calcified Tissue International 80, 31-38.
|
 Heil D. (2006) Predicting activity energy expenditure using the Actical activity monitor. Research Quarterly for Exercise and Sport 77, 64-80.
|
 Janz K.F., Golden J.C., Hansen J.R., Mahoney L.T. (1992) Heart rate monitoring of physical activity in children and adolescents: The Muscatine study. Pediatrics 89, 256-261.
|
 Janz K.F., Levy S.M., Burns T.L., Torner J.C., Willing M.C., Warren J.J. (2002) Fatness, physical activity and television viewing in children during the adiposity rebound period: The Iowa bone development study. Preventive Medicine 35, 563-571.
|
 Kemper H.C.G., Verschuur R. (1977) Validity and reliability of pedometers in habitual activity research. European Journal of Applied Physiology 37, 71-82.
|
 Kilanowski C.K., Consalvi A.R., Epstein L.H. (1999) Validation of an electronic pedometer for measurement of physical activity in children. Pediatric Exercise Science 11, 63-68.
|
 Le Masurier G.C., Corbin C.B. (2006) Step counts among middle school students vary with aerobic fitness level. Research Quarterly for Exercise and Sport 77, 14-22.
|
 Livingstone M.B.E., Coward A.W., Prentice A.M., Davis P.S., Strain J.J., McKenna P.G., Mahoney C.A., White J.A., Stewart C.M., Kerr M.J. (1992) Daily energy expenditure in free-living children: comparison of heart rate monitoring with the doubly labelled water method. American Journal of Clinical Nutrition 56, 343-352.
|
 Louie L.R.G., Eston A.V., Rowlands K.K., Tong D.K., Ingledew H.F. Fu.. (1999) Validity of heart rate, pedometry, and accelerometry for estimating the energy cost of activity in Chinese boys. Pediatric Exercise Science 11, 229-239.
|
 McManus A., Armstrong N., Ring FJ.. (1995) Children in Sport. Patterns of physical activity among primary schoolchildren. Bath. University Press.
|
 McMurray R.G., Baggett C.D., Harrell J.S., Pennell M.L., Bangdiwala S.I. (2004) Feasibility of the Tritrac R3D accelerometer to estimate energy expenditure in youth. Pediatric Exercise Science 16, 219-230.
|
 Nilsson A., Ekelund U., Yngve A., Sjostrom M. (2002) Assessing physical activity among children with accelerometers using different time sampling intervals and placements. Pediatric Exercise Science 14, 87-96.
|
 Ott A.E., Pate R.R., Trost S.G., Ward D.S., Saunders R (2000) The use of uniaxial and triaxial accelerometers to measure children’s free play physical activity. Pediatric Exercise Science 12, 360-370.
|
 Ozdoba R., Corbin C., Le Masurier G. (2004) Does reactivity exist in children when measuring activity levels with unsealed pedometers. Pediatric Exercise Science 16, 158-166.
|
 Parfitt G., Eston R.G. (2005) The relationship between children’s habitual activity level and psychological well-being. Acta Paediatrica 94, 1791-1797.
|
 Pfeiffer K.A., McIver K.L., Dowda M., Almeida M.J., Pate R.R. (2006) Validation and calibration of the Actical accelerometer in preschool children. Medicine and Science in Sports and Exercise 38, 152-157.
|
 Pober D.M., Staudenmayer J., Raphael C., Freedson P.S. (2006) Development of novel techniques to classify physical activity mode using accelerometers. Medicine and Science in Sports and Exercise 38, 1626-1634.
|
 Puyau M.R., Adolph A.L., Vohra F.A., Butte N.F. (2002) Validation and calibration of physical activity monitors in children. Obesity Research 10, 150-157.
|
 Puyau M.R., Adolph A.L., Vohra F.A., Zakeri I., Butte N.F. (2004) Prediction of activity energy expenditure using accelerometers in children. Medicine and Science in Sports and Exercise 36, 1625-1631.
|
 Riddoch C.J., Boreham C.A.G. (1995) The health-related physical activity of children. Sports Medicine 19, 86-102.
|
 Roemmich J.N., Gurgol C.M., Epstein L.H. (2004) Open-loop feedback increases physical activity of youth. Medicine Science in Sports and Exercise 36, 668-673.
|
 Rowlands A.V. (2007) Accelerometer assessment of physical activity in children: an update. Pediatric Exercise Science 19, 252-266.
|
 Rowlands A.V., Eston R.G. (2005) Comparison of accelerometer and pedometer measuresof physical activity in boys and girls, aged 8-10 yrs. Research Quarterly for Exercise and Sport 76, 251-257.
|
 Rowlands A.V., Eston R.G., Ingledew D.K. (1997) Measurement of physical activity in children with particular reference to the use of heart rate and pedometry. Sports Medicine 24, 258-272.
|
 Rowlands A.V., Eston R.G., Ingledew D.K. (1999) The relationship between activity levels, aerobic fitness, and body fat in 8- to 10-yr-old children. Journal of Applied Physiology 86, 1428-1435.
|
 Rowlands A.V., Eston R.G., Powell S.M. (2006) Total physical activity, activity intensity and body fat in 8 to 11 year old boys and girls. Journal of Exercise Science and Fitness 4, 97-103.
|
 Rowlands A.V., Eston R.G., Reilly T. (2001) Field measures of assessing physical activity and energy balance. Exercise Physiology and Kinanthropometry Laboratory Manual: Tests, Procedures and Data 1, 151-170.
|
 Rowlands A.V., Ingledew D.K., Eston R.G. (2000) The effect of type of activity measure on the relationship between body fatness and habitual physical activity in children: A meta-analysis. Annals of Human Biology 27, 479-497.
|
 Rowlands A.V., Powell S.M., Eston R.G., Ingledew D.K. (2002) Relationship between bone mass, objectively measured physical activity and calcium intake in 8-11 year old children. Pediatric Exercise Science 14, 358-368.
|
 Rowlands A.V., Powell S.M., Humphries R., Eston R.G. (2006) The effect of accelerometer epoch on physical activity output measures. Journal of Exercise Science and Fitness 4, 51-57.
|
 Rowlands A.V., Stone M.R., Eston R.G. (2007) Influence of speed & step frequency during walking & running on motion sensor output. Medicine and Science in Sports and Exercise 39, 716-727.
|
 Rowlands A.V., Thomas P.W.M., Eston R.G., Topping R. (2004) Validation of the RT3 triaxial accelerometer for the assessment of physical activity. Medicine and Science in Sports and Exercise 36, 518-524.
|
 Saris W.H.M., Binkhorst R.A. (1977) The use of pedometer and actometer in studying daily physical activity in man. Part I. Reliability of pedometer and actometer. European Journal of Applied Physiology 37, 219-228.
|
 Schneider P.L., Crouter S.E., Bassett D.R. (2004) Pedometer measures of free-living physical activity: comparison of 13 models. Medicine and Science in Sports and Exercise 36, 331-335.
|
 Sequeira M.M., Rickenbach M., Wietlisbach V., Tullen B., Schutz Y. (1995) Physical activity assessment using a pedometer and its comparison with a questionnaire in a large population survey. American Journal of Epidemiology 142, 989-999.
|
 Treuth M.S., Schmitz K., Catellier D.J., McMurray R.G., McMurray D.M., Almeida M.J., Going S., Norman J.E., Pate R. (2004) Defining accelerometer thresholds for activity intensities in adolescent girls. Medicine and Science in Sports and Exercise 36, 1259-1266.
|
 Trost S.G., McIver K.L., Pate R.R. (2005) Conducting accelerometer-based activity assessments in field-based research. Medicine and Science in Sports and Exercise 37, S531-.
|
 Trost S.G., Pate R.R., Sallis J.F., Freedson P.S., Taylor W.C., Dowda M., Sirad J. (2002) Age and gender differences in objectively measured physical activity in youth. Medicine and Science in Sports and Exercise 34, 350-355.
|
 Trost S.G., Ward S.S., Moorehead S.M., Watson P.D., Riner W., Burke J.R. (1998) Validity of the computer science and applications (CSA) activity monitor in children. Medicine and Science in Sports and Exercise 30, 629-633.
|
 Tudor-Locke C., Bell R.C., Myers A.M., Harris S.B., Ecclestone S.A., Lauson N., Rodger N.W. (2004) Controlled outcome evaluation of the First Step Program: a daily physical activity intervention for individuals with type II diabetes. International Journal of Obesity and Related Metabolic Disorders 28, 113-119.
|
 Tudor-Locke C., Sisson S.B., Collova T., Lee S.M., Swan P.D. (2005) Pedometer-determined step guidelines for classifying walking intensity in a young ostensibly healthy population. Canadian Journal of Applied Physiology 30, 666-676.
|
 Tudor-Locke C., Sisson S.B., Lee S.M., Craig C.L., Plotnikoff R.C., Bauman A. (2006) Evaluation of quality of commercial pedometers. Canadian Journal of Public Health 97, S10-S15.
|
 Vincent S., Pangrazi R.P. (2002) Does reactivity exist in children when measuring activity level with pedometers. Pediatric Exercise Science 14, 56-63.
|
 Washburn R., Chin M.K., Montoye H.J. (1980) Accuracy of pedometer in walking and running. Research Quarterly for Exercise and Sport 51, 695-702.
|
 Welk G.J. (2005) Principles of design and analyses for the calibration of accelerometry-based activity monitors. Medicine and Science in Sports and Exercise 37, S501-511.
|
 Welk G.J., Corbin C.B., Dale D. (2000) Measurement issues in the assessment of -physical activity in children. Research Quarterly for Exercise and Sport 71, 59-73.
|
 Welsman J.R., Armstrong N. (1998) Physical activity patterns of 5-to-7-year-old children and their mothers. European Journal of Physical Education 3, 145-155.
|
 Welsman J.R., Armstrong N. (2000) Physical activity patterns in secondary schoolchildren. European Journal of Physical Education 5, 147-157.
|
 Welsman J.R., Armstrong N., Armstrong N., Kirby B.J., Welsman J.R. (1997) Children and Exercise XIX: promoting health and well-being. Physical activity patterns of 5 to 11-year-old children. London. E & FN Spon.
|
|
|
|
|
|
|