|
|
|
ABSTRACT |
The present study intended to verify if the inclusion of intensities above lactate threshold (LT) in the VO2/running speed regression (RSR) affects the estimation error of accumulated oxygen deficit (AOD) during a treadmill running performed by endurance-trained subjects. Fourteen male endurance-trained runners performed a sub maximal treadmill running test followed by an exhaustive supra maximal test 48h later. The total energy demand (TED) and the AOD during the supra maximal test were calculated from the RSR established on first testing. For those purposes two regressions were used: a complete regression (CR) including all available sub maximal VO2 measurements and a sub threshold regression (STR) including solely the VO2 values measured during exercise intensities below LT. TED mean values obtained with CR and STR were not significantly different under the two conditions of analysis (177.71 ± 5.99 and 174.03 ± 6.53 ml·kg-1, respectively). Also the mean values of AOD obtained with CR and STR did not differ under the two conditions (49.75 ± 8.38 and 45.8 9 ± 9.79 ml·kg-1, respectively). Moreover, the precision of those estimations was also similar under the two procedures. The mean error for TED estimation was 3.27 ± 1.58 and 3.41 ± 1.85 ml·kg-1 (for CR and STR, respectively) and the mean error for AOD estimation was 5.03 ± 0.32 and 5.14 ± 0.35 ml·kg-1 (for CR and STR, respectively). The results indicated that the inclusion of exercise intensities above LT in the RSR does not improve the precision of the AOD estimation in endurance-trained runners. However, the use of STR may induce an underestimation of AOD comparatively to the use of CR. |
Key words:
Methodology, linear regression, estimation error, lactate threshold
|
Key
Points
- It has been suggested that the inclusion of exercise intensities above the lactate threshold in the VO/power regression can significantly affect the estimation of the energy cost and, thus, the estimation of the AOD.
- However data on the precision of those AOD measurements is rarely provided.
- We have evaluated the effects of the inclusion of those exercise intensities on the AOD precision.
- The results have indicated that the inclusion of exercise intensities above the lactate threshold in the VO/running speed regression does not improve the precision of AOD estimation in endurance-trained runners.
- However, the use of sub threshold regressions may induce an underestimation of AOD comparatively to the use of complete regressions.
|
An important methodological issue related to the estimation of the accumulated oxygen deficit (AOD) is the choice of intensities for sub maximal testing. In fact, for low intensity exercise, VO2 kinetics presents a mono-exponential behaviour with a minor time delay due to lung-to-muscle oxygen transient (Barstow et. al., 1991). During this brief period, VO2 reflects pulmonary blood flux (Whipp and Ozyener, 1998). However, at exercise intensities above the lactate threshold, a VO2 slow component (SC) arises and alters the aforementioned model. This secondary component induces an increasing VO2 that reflects muscle oxygen consumption (Barstow, 1994). Therefore, the estimation of the energy cost based on VO2 measurements depends on the exercise intensity and on the magnitude of the SC. It has been suggested that the SC is less prominent during treadmill running than cycle ergometer exercise (Billat et. al., 1997; Binsse et. al., 1998; Carter et. al., 2000; Hill et. al., 2003; Jones and McConnell, 1999; Sloniger et. al., 1996; Whipp and Ozyener, 1998). This phenomenon may be explained by the better mechanical efficiency of running compared to cycling (Billat et. al., 1997; Binsse et. al., 1998). Running mechanical efficiency relies partly on stretch shortening movements but cycling is more dependent on concentric work which demands more energy consumption. It has also been shown that the SC is considerably lower in endurance-trained subjects than in untrained subjects (Carter et. al., 2002; Phillips et. al., 1995; Russell et. al., 2002a; Sloniger et. al., 1996; Wormack et. al., 1995; Zoladz et. al., 1995). Therefore, the estimation of the supra maximal energy demand may be less influenced by the exercise intensities during the sub maximal testing when endurance-trained runners are assessed. Some authors have suggested that the VO2 values measured during sub maximal intensities above the lactate threshold are higher than the values estimated from sub-lactate threshold intensities, which would induce significant changes in the regression parameters (Bearden and Moffat, 2001; Green and Dawson, 1995, 1996; Olesen and Secher, 1995; Pederson et. al., 2002; Riley and Cooper, 2002; Yamamoto and Kanehisa, 1995; Zoladz et. al., 1998; Zoladz and Korniewski, 2001; Zoladz et. al., 1995). Additionally, those effects could significantly affect the estimation of the energy cost, therefore the estimation of the AOD (Olesen and Secher, 1995; Wood et. al., 1997; Zoladz et. al., 1998; Zoladz et. al., 1995). Typically, an underestimation of the supra maximal energy demand and of the AOD was observed when sub maximal testing was limited to intensities below the lactate threshold (Olesen and Secher, 1995; Riley and Cooper, 2002; Wood et. al., 1997; Zoladz et. al., 1995; Zoladz et. al., 1998). Additionally, the majority of those studies have analysed cycling exercise. Indeed, only the study by Wood et al. (1997) assessed the response of endurance trained subjects during running exercise. However, none of those studies presented calculations about the precision of the AOD estimation. Medbø et al. (1988) were the first to present a detailed study on the precision of the AOD estimation for treadmill running. Others have subsequently assessed the robustness of the method by the precision of the supra maximal energy demand prediction error (Russell et. al., 2000; 2002a; 2002b) or by the precision of the AOD itself (Reis et. al., 2004). Russell et. al. (2002a) have shown that the inclusion of exercise intensities above the lactate threshold in the VO2/power regression line did not significantly changed the AOD estimations but did improved the precision of the estimated supra maximal energy demand. Taking into account the above considerations, the aims of the present study were twofold: i) to investigate if the inclusion of exercise intensities above the lactate threshold in the regression affects the estimations of the energy demand and of the AOD during treadmill running performed by endurance-trained subjects; ii) to assess if the referred procedure also affects the precision of the energy demand and of the AOD estimations.
Fourteen male subjects volunteered for this study after medical approval and gave their informed consent to participate on the experiments. All subjects were involved in systematic endurance training programs and their mean (± standard deviation) age, height and body weight were, respectively, 26.9 ± 4.7 years, 1.76 ± 0.06 m, 65.8 ± 5.4 kg. All the procedures were in accordance with the Helsinki Declaration of 1975. All exercise was conducted on a laboratory with a controlled temperature (20-25 degrees Celsius) and humidity (35-45%). Subjects completed a sub maximal running test followed by a supra maximal running test 48h later. The tests were performed on a Quasar-med Pulsar (HP Cosmos, Nussdorf, Germany) motorized treadmill with a 0% gradient. On the day between the two tests the subjects limited their training program to a single daily low intensity continuous running session. Through all testing expired gases were collected and analysed with a SensorMedics 2900 metabolic measurement cart (SensorMedics Corporation, Yorba Linda, USA) and VO2 was averaged as 20 s intervals. Before each test, a reference air calibration of the device was performed using a gas sample with a 16% O2 concentration and a 5% CO2 concentration. The flow meter was also calibrated before each testing with a 3000 ml syringe. To assess the blood lactate concentration ([La]), 32 micro-litres of capillary blood samples were collected and analysed with an Accusport Lactate Analyser (Boehringer, Mannheim, Germany). Before each subjects’ test a calibration of the Accusport was performed with several YSI 1530 Standard Lactate Solutions (2.5, 5, 10 and 15 mml.L-1).
Sub maximal testThe test included exercise intensities below and above the 4 mm·L-1 lactate threshold. Each subject performed several six min bouts at a constant speed. The starting running speed was 2.92 to 3.33 m·s-1. Each subsequent bout was performed with a speed increase of 0.37 to 0.46 m·s-1. The starting running speed for each subject as well as the increase between bouts were based on a laboratory pre-testing for each subject. The recovery time between bouts was also individual and based on VO2 measurement during the recovery. Subjects were allowed to start a new bout, when VO2 was less than 2 ml·kg-1·min-1 different from the value observed before the beginning of the first bout. Immediately after the conclusion of each bout [La] was measured as described before. The test was terminated when voluntary exhaustion occurred. The highest VO2 mean value averaged over 20 s was taken as the subject’s peak VO2.
Supra maximal testThe test comprised of a continuous, constant-intensity, exhaustive single bout. The test was finished by voluntary exhaustion. The running speed was previously calculated from the VO2-speed regression equation and corresponded to a relative intensity of 110% of the individual peak VO2. Warm-up for this test included: 5 min of constant intensity low-intensity (≈60% peak VO2); followed by a 5 min break; followed by 2 high-intensity (110% peak VO2) 20 s runs (with 1 min recovery between them); followed by a 3 min break. At the beginning of the test the treadmill was quickly accelerated until the required speed was attained (generally in ≈5 s).
CalculationsThe sub maximal test was performed in order to obtain VO2/running speed relation points that enabled the calculation of a valid regression equation. For each speed, mean VO2 over the last minute of the bout was used for this purpose. Bouts lasting less than 6 min were not included in the regressions. Additionally, bouts that failed to comply with the VO2 steady-state attainment (given by a difference less than 2 ml·kg-1.min-1 between two consecutive minutes) were also not included in the regressions. All the subjects completed five full bouts and ten of them completed a full sixth bout. All data was used to establish the regression lines. A zero speed VO2 (mean 20 s value recorded before the start of the sub maximal test) was included in the regression lines by a non- forced procedure. Two types of regressions were calculated. The complete regressions included all available VO2-speed points and the sub threshold regressions included solely the points observed at exercise intensities below the lactate threshold. Blood lactate accumulation was traced by linear interpolation for determination of the speed corresponding to a 4 mmol·L-1 concentration (V4). The O2 on-kinetics slow component was calculated for each bout as the difference between the mean VO2 during the sixth and the third minutes of exercise (Carter et. al., 2000; Phillips et. al., 1995). The VO2 measured during the supra maximal test was integrated over time to exhaustion to obtain the Accumulated VO2 (VO2Ac). The energy demand (ED), total energy demand (TED) and the accumulated oxygen deficit (AOD) during the supra maximal test were estimated as described elsewhere (Medbø et. al., 1988). The precision of the AOD estimation (AODerror) was calculated as follows (Reis et. al., 2004):
The error for ED is given by the standard error of the predicted value for the energy demand. The error for VO2 was assumed to be 3% (Robergs and Burnett, 2003).
StatisticsData was analysed with SPSS 10.0 (SPSS Science, Chicago, USA) software and the graphics were designed with Sigma Plot 8.0 (SPSS Science, Chicago, USA) software. The results are presented as means ± standard deviations (SD). Linear regression was used an all appropriate data. The scatter around the regression line and the correlation coefficient, were used as measures of the fitness of the regression lines. Mean differences were tested by paired samples t-tests. The statistical significance was set to p ≤ 0.05.
The VO2 on-kinetics during the sub maximal exercise bouts is presented in Figure 1. The O2 on- kinetics slow component mean values varied between -43.3 ± 82.3 and 74.3 ± 118.4 ml·min-1 on the first five sub maximal bouts and present a larger mean value on the sixth bout (181.9 ± 240.2 ml·min-1). The mean intensity of the exercise bouts varied between ≈56% (on the first bout) and ≈94% (on the sixth bout). There were no significant differences between complete and sub threshold regressions in the slope and in the y-intercept mean values (see Figure 2). The sub threshold regressions presented a better robustness than the complete regressions as the standard error of the regression line (Šœy.x) was lower (p ≤ 0.05) and the correlation coefficient (R) was higher (p ≤ 0.05). Table 1 presents the estimations for the supra maximal running test. There were no significant differences between the use of complete or sub threshold regression lines to estimate the total energy demand (TED) or the accumulated oxygen deficit (AOD). The differences in the measures of the precision for the estimation of TED (TEDerror) and of the AOD (AODerror) were also non-significant.
Based on the sub maximal VO2 measurements, we have determined linear regressions using only the VO2/running speed points observed during exercise intensities below the lactate threshold (sub threshold regressions), as well as the regression lines including all available steady-state VO2 measurements (complete regressions). With this analysis, we intended to verify the effects of the inclusion of exercise intensities above the lactate threshold (LT) upon the regression equations. Some authors have suggested that when the regression lines are drawn with the use of high sub maximal intensities (above the LT) rather than lower intensities, significant changes do occur in the regression parameters (Bearden and Moffat, 2001; Green and Dawson, 1995, 1996; Olesen and Secher 1995; Pederson et. al., 2002; Riley and Cooper, 2002; Robergs and Burnett, 2003; Yamamoto and Kanehisa, 1995; Zoladz et. al., 1998; Zoladz and Korniewski, 2001; Zoladz et. al., 1995). Our results do not support the aforementioned studies (see Figure 2). In fact, we did not find significant differences in the slope and in the y-intercept between the two types of regression lines. Others have also failed to observe significant changes in the regression line by the adding of exercise intensities above the LT (Belli et. al., 1995; Medbø, 1992; Medbø and Burgers, 1990; Medbø et. al., 1988). It was suggested that such effects are more likely to occur for cycle ergometer than for treadmill exercise (Medbø, 1996) which may explain our observations. The fitness of the regression lines was better for the sub threshold when compared with the complete regressions; since the mean standard error of the regression line (Šœy.x) was lower (p ≤ 0.05) and the mean correlation coefficient (R) was higher (p ≤ 0.05) for the former. These differences may be explained by the fact that the complete regressions have included VO2 measurements above the LT. These measurements could have suffered the influence of the VO2 on-kinetics slow component (SC), thus resulting in increased VO2 values and a deviation from the linearity of the complete regression lines (see Figure 2). Nonetheless, those deviations may be considered negligible, since they have not induced significant changes on the regression parameters. In fact, the VO2 attained a steady-state during all sub maximal exercise bouts (see figure 1) and the SC mean values (less than 100 ml·min-1 except for the highest sub maximal exercise intensity) are lower than the data present on the literature (Carter et. al., 2000; 2002; Pringle et. al., 2002). The low SC mean values that we have observed may be explained by the fact that the subjects were endurance-trained runners. Indeed, it has been shown that the SC is lower for endurance-trained subjects when compared to other populations (Belli et. al., 1995; Chilibeck et. al., 1997; Phillips et. al., 1995). Moreover, the subjects in the present study were tested on the treadmill and it has also been shown that the SC is lower during treadmill exercise compared to other modes of exercise, such as cycling (Billat et. al., 1997; Binsse et. al., 1998, Carter et. al., 2000; Hill et. al., 2003; Jones and McConnell, 1999; Sloniger et. al., 1996; Whipp and Ozyener, 1998). The present study also intended to assess if the inclusion in the regression of exercise intensities above the lactate threshold modify the precision of the total energy demand (TED) and of the accumulated oxygen deficit (AOD). Therefore, we have compared the TED and AOD estimations when using complete or sub threshold regression lines (see Table 1). No significant differences were found, although the use of the sub threshold regressions tended to induce lower values for TED and AOD. The estimation errors for TED and AOD were smaller when the complete regressions were used, although the differences were not significant. The relative imprecision of the TED was less than 2% in both procedures. This can be considered as a low imprecision when compared to data on the literature (Medbø et. al., 1988; Russell et. al., 2000; Russell et. al., 2002a; 2002b). The smaller error of the TED when estimated from the complete regressions can be explained by the mathematics of the regression model itself. In fact, the larger the difference between the highest exercise bout intensity included in the regression and the mean exercise intensity in the supra maximal test, the larger will be the standard error of the predicted value (which is the indicator of the TED imprecision). The relative imprecision of the AOD estimation was ≈10.1% and ≈11.2%, respectively for the complete and for the sub threshold procedures. These relative errors seem to be large compared to Medbø’s et al. (1988) validation study concerning the method. However, our calculations for the AOD imprecision took a VO2 measurement relative error of 3% (Robergs and Burnett, 2003), while the aforementioned authors have included in their calculations a 0.35 ml·kg-1·min-1 imprecision for the VO2 measurement (which represents a ≈0.6% error for a 60 ml·kg-1·min-1 VO2). If we had included that same value for VO2 measurement error in our calculations, the total imprecision of the AOD estimation would be ≈7% and ≈8%, respectively for the complete and sub threshold regressions. Nevertheless, we have previously observed a smaller AOD imprecision (≈3%) even with the inclusion of a 3% relative error for the VO2 measurement on the calculations (Reis et. al., 2004). It was interesting to observe that the better robustness of the sub threshold regression lines (either assessed by the Šœy.x or by the R), was not matched by a lower prediction error for the TED (and thus for the AOD estimation). Others have also concluded that the fitness of the regression line is not necessarily the best indicator of the validity of the method (Russell et. al., 2002a). Our results suggest that the use of complete regressions is better compared to sub threshold regressions for endurance-trained runners, as the prediction error is lower and a possible underestimation of the TED and the AOD is avoided.
ConclusionsIn summary we conclude that the inclusion of exercise intensities above the lactate threshold in the VO2/running speed regression does not improve the precision of the TED and of the AOD in endurance-trained runners. However, there might be a tendency for an underestimation of the AOD and for a larger imprecision when exclusively sub threshold intensities are used. Therefore, our results suggest that the use of complete regressions is more suitable to estimate the TED and the AOD of endurance-trained subjects.
|
AUTHOR BIOGRAPHY |
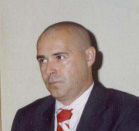 |
Victor M. Reis |
Employment: Ass. Prof. Depart. of Sport Sci, Univ. of Trás-os-Montes and Alto Douro. |
Degree: PhD |
Research interests: Physiological and biomechanical indicators of energy cost during physical activities. |
E-mail: vreis@utad.pt |
|
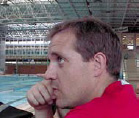 |
António J. Silva |
Employment: Assoc. Prof. Depart. of Sport Sci, Univ. of Trás-os-Montes and Alto Douro. |
Degree: PhD |
Research interests: Physiological and biomec-hanical indicators of energy cost during physical activities. |
E-mail: ajsilva@utad.pt |
|
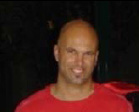 |
António Ascensão |
Employment: Ass. Prof. Faculty of Sport Sciences and PE, Oporto University. |
Degree: PhD |
Research interests: The role of exercise on the redox modulation of cardiac and skeletal muscles, with particular focus on mitochondrial function. |
E-mail: aascensão@fcdef.up.pt |
|
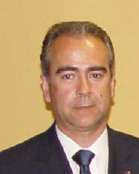 |
José A. Duarte |
Employment: Prof., Faculty of Sport Sciences and PE, University of Porto. |
Degree: PhD |
Research interests: Acute and chronic reactions of skeletal muscle induced by exercise and other types of aggressive stimulus. |
E-mail: jarduarte@fcdef.up.pt |
|
|
|
REFERENCES |
 Barstow T. (1994) Characterization of VO2 kinetics during heavy exercise. Medicine and Science in Sports Exercise 26, 1327-1334.
|
 Barstow T., Molé P. (1991) Linear and nonlinear characteristics of oxygen uptake kinetics during heavy exercise. Journal of Applied Physiology 71, 2099-2106.
|
 Bearden S., Moffat R. (2001) Leg electromyography and the VO2-power relationship during bicycle ergometry. Medicine and Science in Sports Exercise 33, 1241-1245.
|
 Belli A., Lacour J., Komi P., Candau R. (1995) Mechanical step variability during treadmill running. European Journal of Applied Physiology 70, 510-517.
|
 Billat V., Binsse V., Haouzi P., Koralsztein J. (1997) VO2 slow component for a supra lactate threshold exercise and fatigue: effect of type of exercise (running vs. cycling) and stride or pedalling rates (Abstract). Archives International Physiology Biochemistry and Biophysics 105, 8-12.
|
 Binsse V., Haouzi P., Koralsztein J., Billat V. (1998) VO2 slow component for a supra lactate threshold exercise depends on type of exercise but is not influenced by the stride and pedalling rates. Science et Motricité 35-36, 85-86.
|
 Carter H., Jones A., Barstow T., Burnley M., Williams C., Doust J. (2000) Oxygen uptake kinetics in treadmill running and cycle ergometry: a comparison. Journal of Applied Physiology 89, 899-907.
|
 Carter H., Pringle J., Jones A., Doust J. (2002) Oxygen uptake kinetics during treadmill running across exercise intensity domains. European Journal of Applied Physiology 86, 347-354.
|
 Chilibeck P., Paterson D., Cunningham D., Taylor A., Noble E. (1997) Muscle capillarization, O2 difusion distance and VO2 kinetics in old and young individuals. Journal of Applied Physiology 82, 63-69.
|
 Green S., Dawson B. (1995) The oxygen uptake-power regression in cyclists and untrained men: implications for the accumulated oxygen deficit. European Journal of Applied Physiology 70, 351-359.
|
 Green S., Dawson B. (1996) Methodological effects on the VO2 - power regression and the accumulated O2 deficit. Medicine and Science in Sports Exercise 28, 392-397.
|
 Hill D., Halcomb J., Stevens E. (2003) Oxygen uptake kinetics during severe intensity running and cycling. European Journal of Applied Physiology 89, 612-618.
|
 Jones A., McConnell A. (1999) Effect of exercise modality on oxygen uptake kinetics during heavy exercise. European Journal of Applied Physiology 80, 213-219.
|
 Medbø J. (1992) Is the O2 deficit an accurate quantitative measure of the anaerobic energy production during intense exercise? Reply to the letter to the editor. Journal of Applied Physiology 73, 1208-1209.
|
 Medbø J. (1996) Medbø responds to Bangsbo´s paper. Canadian Journal of Applied Physiology 21, 364-369.
|
 Medbø J., Burgers S. (1990) Effect of training on the anaerobic capacity. Medicine and Science in Sports Exercise 22, 501-507.
|
 Medbø J., Mohn A., Tabata I., Bahr R., Vaage O., Sejersted O. (1988) Anaerobic Capacity determined by Maximal Accumulated O2 Deficit. Journal of Applied Physiology 64, 50-60.
|
 Olesen H., Secher N. (1995) Letter to the editor: Oxygen deficit of sprint and middle distance runners (reply). European Journal of Applied Physiology 70, 194-.
|
 Pedersen P., Sorensen J., Jensen K., Johansen L., Levin K. (2002) Muscle fiber type distribution and nonlinear VO2-power relationship in cycling. Medicine and Science in Sports Exercise 34, 655-661.
|
 Phillips S., Green H., MacDonald M., Hughson R. (1995) Progressive effect of endurance training on VO2 kinetics at the onset of sub maximal exercise. Journal of Applied Physiology 79, 1914-1920.
|
 Pringle J., Carter H., Doust J., Jones A. (2002) Oxygen uptake kinetics during horizontal and uphill treadmill running in humans. European Journal of Applied Physiology 88, 163-169.
|
 Reis VM., Duarte JA., Espírito-Santo J., Russell AP. (2004) Determination of accumulated oxygen deficit during a 400m run. Journal of Exercise Physiology 7, 77-83.
|
 Riley M., Cooper C. (2002) Ventilatory and gas exchange responses during heavy constant work-rate exercise. Medicine and Science in Sports Exercise 34, 98-104.
|
 Robergs R.A., Burnett A.F. (2003) Methods used to process data from indirect calorimetry and their application to VO2max. Journal of Exercise Physiology 6, 44-57.
|
 Russell AP., Rossignol P., Lo S. (2000) The precision of estimating the total energy demand: implications for the determination of the accumulated oxygen deficit. Journal of Exercise Physiology 3, 55-63.
|
 Russell AP., Rossignol P., Snow R., Lo S. (2002a) Improving the precision of the accumulated oxygen deficit using VO2-power regression points from below and above the lactate threshold. Journal of Exercise Physiology 5, 23-31.
|
 Russell AP., Rossignol P., Snow R., Lo S. (2002b) Cycling at 120 when compared to 80 rev/min increases the accumulated oxygen deficit but does not affect the precision of its calculation. Journal of Exercise Physiology 5, 32-38.
|
 Sloniger M., Cureton K., Carrasco D., Prior B., Rowe D., Thompson R. (1996) Effect of the slow component rise in oxygen uptake on VO2max. Medicine and Science in Sports Exercise 28, 72-78.
|
 Whipp B., Ozyener F. (1998) Oxygen uptake kinetics and domains of exercise intensity. Science et Motricité 35-36, 73-74.
|
 Womack C., Davis S., Blumer J., Barrett E., Weltman A., Gaesser G. (1995) Slow component of O2 uptake during heavy exercise: adaptation to endurance training. Journal of Applied Physiology 79, 838-845.
|
 Wood D., Myers S., Fallowfield J. (1997) Second Annual Congress of the European College of Sports Science, August 20-23, Copenhagen-Denmark. Non-linear VO2-running speed relationship in well trained runners: implications for the assessment of running speed at VO2max.
|
 Yamamoto M., Kanehisa H. (1995) Dynamics of anaerobic and aerobic energy supplies during sustained high intensity exercise on cycle ergometer. European Journal of Applied Physiology 71, 320-325.
|
 Zoladz J., Duda K., Majerczak J. (1998) Oxygen uptake does not increase linearly at high power outputs during incremental exercise test in humans. European Journal of Applied Physiology 77, 445-451.
|
 Zoladz J., Korzeniewski B. (2001) Physiological background of the change point in VO2 and the slow component of oxygen uptake kinetics. Journal of Physiology and Pharmacology 52, 167-184.
|
 Zoladz J., Rademaker A., Sargeant A. (1995) Non-linear relationship between O2 uptake and power output at high intensities of exercise in humans. Journal of Physiology 488, 211-217.
|
|
|
|
|
|
|