|
|
|
ABSTRACT |
Exercise adaptations to strength, anaerobic and aerobic training have been extensively studied in adults, however, young people appear to respond differently to such exercise stimulus in comparison to adults. In addition, because overtraining in young athletes has received little attention, this important area is also discussed. Resistance training in children can be safe and effective. It has the potential to improve sport performance, enhance body composition and reduce the rate of sport incurred injury. Furthermore, with the appropriate stimulus, prepubertal and adolescent athletes can show significant increments in muscle strength (13 - 30%). Children can improve anaerobic power (3%-10% Mean Power and 4%-20% in Peak Power), although the mechanisms responsible for the improvements in children remain unclear. Children show a ‘reduced’ trainability of peak VO2 in comparison to adults. Nevertheless, their aerobic power is trainable, with improvements reported at approximately 5%. Moreover, improvements in other variables like exercise economy or lactate threshold may occur without significant changes in peak VO2 The limited evidence available indicates that overtraining is occurring in young athletes (30% prevalence), highlighting the importance of further research in to all the possible contributing factors - physiological, psychological and emotional - when investigating overtraining. |
Key words:
Prepubertal and adolescent athletes, trainability, mechanisms, resistance training, anaerobic training, aerobic training, overtraining
|
Key
Points
- Children’s strength, anaerobic and aerobic power is trainable, although the improvements may be smaller than seen in adults.
- Children can demonstrate significant gains in muscle strength with resistance training (13 - 30%).
- Improvements in mean power (3 - 10%) and peak power (4 - 20%) are reported in children.
- Aerobic fitness can improve with training in children by approximately 5%.
- Limited available evidence indicates an occurrence of overtraining in young athletes of around 30%.
|
Strength gainsThere have been few studies that have looked at differences in training induced strength improvements between genders, but it appears that both sexes respond similarly. Kirsten (1963) (cited in Blimkie, 1992), studied the effects of 15 weeks of isometric training in groups of boys and girls (ages 11-12, 13-14 and 15-16 years), and found significant increases in strength in only the two older age groups for the girls, and only in the oldest age group for the boys - however the magnitude of change, when evident, was similar between the sexes. Other work showed that 12 weeks of dynamic strength training in prepubertal boys and girls, resulted in similar increases in strength in both sexes (McGovern, 1984; cited in Blimkie, 1992).
Muscle SizeThe gender differences in muscle size are small until mid-puberty, increase progressively with age during late puberty and adolescence, and reach peak difference during early adulthood (Malina and Bouchard, 1991). As discussed previously, strength training-induced muscle hypertrophy has not been consistently observed in either prepubertal or pubertal boys (Blimkie and Sale, 1998; Fukunaga et al., 1992; Mersh and Stoboy, 1989). Due to a lack of investigations on girl’s resistance training responses there are few available data to make confident gender comparisons, but training induced muscle hypertrophy has also been reported in girls. Fukunaga (1976) (cited in Blimkie and Bar-Or, 1996) reported an 8.3% increase in upper arm lean area in 13-year-old adolescent girls engaging on a 3- month isometric strength-training programme. Conversely, Blimkie and colleagues (1996), employing 26 weeks of heavy resistance training in adolescent girls (14-17 years) observed significant strength gains but found no increase in anthropometric measurements of the upper arm girth or quadriceps mCSA determined by computerized tomography. It appears that if muscle hypertrophy is seen after resistance training it is a sex independent response (Blimkie et al., 1996).
Can children be physically trained? Is training healthy for children? Can a child become overtrained? These are just some of the questions that exercise and sports scientists have debated over in the past; however conclusive answers to these issues remain elusive. As Armstrong and Welsman, 2002 state, “children are not mini-adults ”indicating that our understanding of the exercise physiology of an adult cannot just be scaled down and applied to children; Indeed the very process of growth and maturation complicates our understanding of the trainability of children as this process mimics the effects of training (Baxter-Jones et al., 1995; Naughton et al., 2000) and thus needs to be accounted for when determining the success or failure of an exercise training programme. Additionally with the majority of studies being cross-sectional in nature it is difficult to partial these aforementioned maturational effects on the training response. With these caveats understood the available literature suggests that children can show improvements in strength (Falk and Tenembaum, 1996; Payne et al., 1997; Tolfrey, 2007), aerobic fitness (Baquet et al., 2003; Baxter-Jones et al., 1993; Kobayashi et al., 1978), and anaerobic fitness (Rowland, 2005; Tolfrey, 2007) with exercise training.
1. Strength Trainability During ChildhoodWith the increasing participation in youth sports (American Academy of Pediatrics 2000; Baxter-Jones and Mundt, 2007), young athletes, like adults, strive to achieve higher performances through improving their health and fitness, with resistance training being used to enhance an athlete’s performance in sport (Kraemer et al., 1989)., The development of muscle strength through resistance training in children is still the subject of some debate and criticism. In the past, clinicians would consider resistance training contraindicated in children due to the risk of epiphyseal plate injury and because it was believed that children were incapable of increasing their strength or muscle mass through such exercise (Myer and Wall, 2006). Although early studies suggested that resistance training did not lead to significant improvements in strength (Vrigens, 1978), subsequent studies have suggested that children are indeed able to increase their strength (Blimkie, 1992; Blimkie and Bar-Or, 1996; Mahieu et al., 2006; Myer and Wall, 2006; Ozmun et al., 1994).
1.1. Increases in strengthMuscular strength can be defined as the maximal force or tension a muscle or a group of muscles can generate at a specified velocity (Knuttgen and Kraemer, 1987). The positive effects of resistance training on adult athletes have been well documented in the literature (Chilibeck et al., 1998; Sailors and Berg, 1987; Staron et al., 1994); isometric strength training in adult men can result in increases in strength of 92% and in muscle size of 23% (Ikai and Fukunaga, 1970); dynamic weight training can increase strength by 30% (Moritani and DeVries, 1980). Both types of training are also effective in increasing strength in pre-pubertal children and adolescents, with both groups also showing considerable improvements (Rowland, 2005; Tolfrey, 2007). The findings of two meta-analyses on resistance training in children support these claims. Falk and Tenenbaum (1996) analysed 28 studies involving girls and boys under the age of 12 and 13 years, respectively, with nine of these studies subsequently included to calculate effect size (ES). Findings indicated an overall mean ES of 0.57 (0.12), suggesting a significant improvement in strength is achievable in children. The typical gains in muscle strength were approximately 13 - 30%, greater than that which should be expected from growth and maturation. It was interesting to note that the largest absolute and relative gains in strength were seen in the youngest aged children (Falk and Tenembaum, 1996). Similar findings were observed in the other meta-analysis by Payne and colleagues (1997), which included 28 studies and reported a mean ES of 0.75 (0.57). Participants were categorized into “younger ”or “older ”groups based on the average age at peak height velocity (PHV). The authors concluded that, regardless of participant or study characteristics, children and youth can demonstrate considerable increases in muscular strength as a result of resistance training (Payne et al., 1997). Although the overall picture suggests that children can in fact improve muscular strength, the studies on which these conclusions are based are not without their problems; a lack of control for a learning effect that could account for early strength gains, not reporting adherence rates, non-randomization into the training and control groups, and a over-reliance on boys as study participants. Finally, apart from 2 studies that were longer than 20 weeks, all other studies were of relatively short duration, making it difficult to delineate whether a dose response factor operates with resistance training.
1.2. Why do children get stronger?The mechanisms underlying strength improvements and muscle hypertrophy in adults arise through an interaction between neural and hormonal mechanisms. However, in the pre-pubescent population, muscle hypertrophy is not believed to be the primary factor in strength improvement. When an adult undertakes a resistance training programme, the gains in strength that arise in the first three to five weeks occur primarily through neural mechanisms, after this period, increased muscle fibre size is the main contributor to strength improvements (Tolfrey, 2007).
1.3. Safety of resistance trainingIt appears that developing strength during childhood will lead to improvements in sport performance (Faigenbaum, 2000; Kraemer et al., 1989), and diminish susceptibilities to injuries (American Academy of Pediatrics, 2000). Furthermore, resistance training has also been recommended for girls as an osteoporosis preventive measure (Myer and Wall, 2006). Strength training can be a safe and effective method of conditioning for children and adolescents (Coutts et al., 2004; Faigenbaum, 2000; Myer and Wall, 2006). However, training programmes performed without proper supervision can greatly increase the risk of musculoskeletal injuries such as fractures of the epiphyseal plate, herniated intervertebral disks, and lower back injuries in both children and adolescents. However, beneficial adaptations in ligament tendon and bone strength may reduce injury risk in a young athlete’s chosen sport (Fleck and Falkel, 1986). Lenhard and colleagues (1996) reported that adding a resistance training programme to a male soccer team significantly reduced injury rates. Moreover, Hejna and Rosenberg, 1982 reported that young athletes (13-19 years) who included resistance training as part of their exercise regimen demonstrated decreased injuries and spent less time in rehabilitation when compared with their team mates (Hejna and Rosenberg, 1982). Thus, resistance training may be considered not only a safe activity but it also may reduce injuries in competitive athletes.
1.4. Resistance training programme prescriptionThe component parts of the training programme will play a great influence on the extent to which a child improves strength; those are: the resistive load, the number of repetitions used, the number of sets, the rest in between repetitions and groups of repetitions (sets), session frequency and the total length of the programme. Furthermore, for the programme to be safe and healthy, children should not engage in high-intensity efforts (e.g. maximal or near maximal lifts with free weights or weight machines), should avoid isolated eccentric training, and exercise on a circuit-training programme in order to stimulate possible cardiorespiratory benefits (Kraemer et al., 1989). Young athletes should be allowed 2 to 4 weeks of adaptation to basic resistance training that should consist of a minimum of 2 sessions per week, with a limited number of sets (one or two), basic exercises, moderate loads (12-15 RM) and adequate recovery (approximately 48 hours). This is important whether it is the first time an individual has weight trained or a new programme is being started after weeks or months of no training (Kraemer et al., 1989; Tolfrey, 2007). Furthermore, if experienced, children may be able to exercise at higher intensities and with a higher frequency of training per week; indeed high intensity and volume training has been shown to be effective in children (Mersh and Stoboy, 1989; Nielson et al., 1980). Again, extremely high-intensities (very high loads) should be avoided, especially during prepubertal years, as should eccentric-type of training until the later stages of adolescence (Blimkie and Bar-Or, 1996).
1.5. Key point summaryThe data challenges the myth that resistance training in children is dangerous, may result in growth plate injuries and also that it is ineffective because young athletes are unable to increase their strength without the “necessary ”circulating hormones. Both prepubertal children and adolescents can demonstrate significant gains in muscle strength with resistance training (between 13 - 30%). Muscle hypertrophy is limited in prepubertal children, but is more frequently observed from puberty onwards, and may reflect changes in circulating concentrations of growth and sex hormones. Independent of changes in muscle hypertrophy, neuromuscular adaptations underpin increases in strength in young children. Resistance training can be a safe and effective type of exercise for young athletes if programmes are well designed and supervised by knowledgeable personnel. Strength training has the potential of improving sport performances, enhancing body composition and reducing the rate of sport injury and rehabilitation time following injury.
2. Anaerobic trainingThe trainability of the anaerobic system in young people has received less attention compared to strength and / or aerobic fitness. Nevertheless, anaerobic capacity and anaerobic power can impact sporting performance and therefore the trainability of these attributes is of interest to coaches, athletes and sports scientists. The anaerobic trainability of young people is difficult to study given the many facets of performance and capacity for short-term, maximal intensity activities. One reason for such a paucity of data is that the equipment and protocols for studying anaerobic performance are quite complex compared to those used for strength programmes; the latter only requires the measurement of force whereas the former calls for the simultaneous measurement of force production throughout time (Blimkie and Bar-Or, 1996). In addition, ethical factors have limited our ability to quantify changes in muscle glycolytic activity at the cellular level in children. Thus, these enhancements are inferred from changes seen from the product of their activity (i.e. peak power). Complicating this further is the fact that power production during tests such as the Wingate or the Force-Velocity test are a composite of not just glycolytic activity rate, but of other factors such as muscle size and neural activity. Also, when assessing speed trainability and other short-term activities like high jump or countermovement jump, the plasticity of neuromuscular coordination and motor skills must also be considered (Blimkie and Bar-Or, 1996; Rowland, 2005). Finally, different approaches have been taken during investigations of anaerobic power, but the most common has been the use of repetitions of short-term activities lasting from 5 to 30 seconds. These activities have mostly involved cycling and running at maximal intensities, with participants being tested on a cycle ergometer and treadmill, respectively (Blimkie and Bar-Or, 1996; Rotstein et al., 1986; Sargeant et al., 1985).
2.1. Can children improve anaerobic performance with training?Adult studies have demonstrated the trainability of anaerobic power - improvements after training of around 12% in peak power (PP), and 6-7% in mean power (MP) (Barnett et al., 2004; Nevill et al., 1998; Sharp et al., 1986). These training induced changes in power output arose through augmented intramuscular levels of PC, ATP, and glycogen, increased activity of anaerobic enzymes, hypertrophy of fast-twitch muscle fibres, augmented lactate production at submaximal and maximal intensities, and an improvement in performance in short-burst activities (McArdle et al., 2000).
2.2. Gender differences in anaerobic powerStudies looking specifically at gender differences in anaerobic trainability in children are lacking. Though some studies used both boys and girls as participants, subsequent analysis on gender was not performed (Baquet et al., 2002; Bencke et al., 2002; Hawley and Williams, 1991; Obert et al., 2001a). Naughton and co-workers (1998) found male adolescents display a greater magnitude of improvement in PP and MP after training, but no comparative data for prepubertal children are available.
2.3. What factors explain the changes in anaerobic power in children?Information relating anaerobic metabolic changes with training in young athletes is lacking, due in part to the practicalities of measuring metabolic responses directly (e.g. enzyme activity and muscle fibre histology), which are dependent on invasive muscle biopsies. However, the limited data available do however provide a window to attempt to explain the changes seen in children’s anaerobic power.
2.4. Anaerobic training programme prescriptionBased on the available literature is seems that an anaerobic training programme can be successful on inducing positive biochemical and performance adaptations in young children. Nevertheless, the problems described for the limitations of training studies also apply to the implementation of an anaerobic programme; methodological concerns on the evaluation of performance on anaerobic tests, and difficulties on sustaining the motivation and interest of young children and adolescents. Therefore, in order to keep the motivation levels high an anaerobic programme that includes both aerobic and short-burst activities may be beneficial. As such, an anaerobic programme should include a minimum of 3 sessions per week, of 30 minutes to hour duration. Children should engage in short high intensive activities (not less than 90% of maximal effort), like sprint running, jumping, throwing, plyometrics, sprint cycling, interspersed with submaximal aerobic activities. Finally, the duration of the anaerobic exercises should aim 20-30 seconds (Armstrong and Welsman, 1993).
2.5. Key-point summaryImprovements in MP ranging from 3% to 10% and in PP from 4% to 20% are reported in children, showing that the anaerobic fitness is to some degree trainable. There is a lack of knowledge about the mechanisms responsible for the improvements seen in anaerobic fitness in children - muscle size, fibre type, neurological and biochemical changes may underlie the response, but require future research.
3. Aerobic TrainingIn adults, training-induced adaptations to aerobic training have been extensively studied, and it is well documented that adults can improve their cardiorespiratory (aerobic) fitness levels if they are given the appropriate training stimulus. The focus of child-based studies has largely centered upon improving fitness levels for either athletic performance or for its relationship with health outcomes. Typically the training programme design used with children mirrors that used for adults (Rowland, 1985), whether this is appropriate or not remains to be clarified, but evidence indicates that higher intensities may have to be used to initiate a response in children (Baquet et al., 2003; Massicotte and Macnab, 1974). Unfortunately, despite the abundant research on aerobic training, the relationship between exercise training and adaptations in aerobic metabolism are still not well understood in prepubertal and early pubertal children (Baxter-Jones and Mundt, 2007; Tolfrey, 2007). Early investigations have generally assumed that young children would have minimal or no response in aerobic fitness (peak VO2) to endurance training, which was attributed to either a high inherent level of physical activity (American Academy of Paediatrics, 1976; Yoshida et al., 1980), or to some unexplained limitations in the biological responsive mechanisms related to maturation (Katch, 1983). This apparent “inferiority ”of children to develop aerobic fitness was thought to be a reflection of the maturational differences between pre- and postpubertal children. The ‘trigger hypothesis’ (Katch, 1983) implied that there would be a time (trigger point) in a child’s life - namely puberty - before which the effects of training would be negligible, or would not occur at all. The prepubertal hormonal environment of low GH and sex steroid concentrations were considered not suitable to bring about measurable improvements in physiological function and thus, enhanced aerobic fitness with training.
3.1. By how much can children improve aerobic fitness?According to the literature it is believed that children show a reduced magnitude of improvements in aerobic fitness after endurance type training than that seen in adults. Young adults typically show an increase in peak VO2 of around 15-20%, although a large intra- subject variation can be present (Bouchard et al., 1992), in children however this is reduced to <10%. The meta-analysis of Payne and Morrow (1997) analysed the data from 69 studies (of which 28 met the inclusion criteria) that were either a cross- sectional comparison between trained and untrained children or a pre-test/ post-test design. Greater differences between trained and untrained participants (Effect Size (ES) of 0.94 ± 1.00) were seen, but less than a 5% change in peak VO2 (approximately 2mL·kg-1·min-1) were noted in the pre-post studies (ES 0.35 ± 0.82). More recently, Baquet et al., 2003 argued that improvements in peak VO2 of around 5-6% are observed when the data are analysed independently by sex or pubertal status. These authors also stated that when only studies that reported significant changes were considered, peak VO2 improvements rose to 8-10% (Baquet et al., 2003). In line with the two previous studies, Rowland, 2005 compiled a series of what he considered to be well-designed investigations in children and stated that the improvements in aerobic power were indeed quite small, with the greatest improvement being 10%, but the average change being 5.8%.
3.2. Can pre-pubertal children improve aerobic fitness?To return to the hypothesis of Katch, 1983 discussed previously, it appears that prepubertal children are indeed able to show increased aerobic fitness levels with training (Baquet et al., 2002; Kobayashi et al., 1978; McManus et al., 1997; Obert et al., 2003; Tolfrey, 2007). Indeed, work by Danis et al. (2003) with sets of prepubertal and circumpubertal monozygotic twins - one acting as the control, the other undergoing endurance training for 6 months, 3 times per week, with the intensity set at 85-120% of their lactate threshold (LT) - saw improvements only in the prepubertal children and not the circumpubertal twins, clearly contradicting the postulations of Katch. Furthermore, one investigation looking at the effects of two types of training (cycling and sprint) during 8 weeks (3 times per week), on aerobic power of prepubertal girls showed significant increases in peak VO2 (McManus et al., 1997). The literature suggests that prepubertal and adolescent athletes are aerobically trainable, nevertheless, one should not disregard Katch’s hypothesis since prepubertal children seem able to improve aerobic fitness to a lesser magnitude compared to adults.
3.3. Physiological mechanisms affecting aerobic fitnessWhat potential reasons have therefore been given to explain the reduced magnitude of the training response seen in children compared to adults?
3.4. Aerobic fitness: A blunted response or too narrow view of the concept?From the presented data it is clear that children can experience improvements in cardiorespiratory function after exercise training, although not to the same extent as adolescents or adults. The majority of the studies report increments in peak VO2 typically around 6%, with exceptional cases when studies demonstrate improvements greater than 15%. However methodological flaws related with the intensity used during the training study, initial level of fitness of the subjects, lack of controls, a mismatch of training type with testing mode (e.g. swim training with cycle testing), insufficient training intensities, all possibly contribute to the reduced aerobic fitness gains. Another issue related with studying aerobic fitness may be related with to the over-reliance on using peak VO2 as a ‘gold standard’. What if the concept of aerobic fitness has not been interpreted fully in children? Does focusing on changing peak VO2 provide too narrow a focus for what is meant by the term ‘aerobic trainability’? After all peak VO2 is only one component of the factors that contribute to endurance exercise performance. According to Bosh, 2006, misunderstandings surrounding scientific dogma may have influenced sport scientists’ interpretation of the evidence that is used to extrapolate and generalize results. As such, because studies mainly used peak VO2 as a parameter of evaluation it is hard to ascertain if other changes in aerobic fitness were also present. Indeed adult data indicates that peak VO2 is of limited value to athletes, because exercise scientists and coaches cannot use it as a predictor for future performance, or for the prescription of potentially “optimal ”training (Bosh, 2006; Jones, 2006). Although peak VO2 might show limited scope for change, other physiologic parameters known to be related to aerobic exercise performance such as lactate and ventilatory thresholds, exercise economy, and VO2 kinetic response might have been altered favourably (Jones, 2006; Jones and Carter, 2000). The longitudinal studies on Paula Radcliff (Jones, 2006) and Lance Armstrong (Coyle, 2005) epitomize this; both these world class athletes showed a relatively stable peak VO2 during long periods of their careers, yet economy and performance enhanced significantly in the same time. In children the data indicate similar adaptations are evident. Welsman et al. (1997) found no changes in peak VO2 in prepubertal girls after endurance training; however a significant reduction in submaximal exercise lactate levels were noted in these same children. Haffor et al., 1990 noted an improvement in lactate threshold in a group of 11-year-old boys after interval training yet peak VO2 was unchanged (Haffor et al., 1990). Studies on young children have also seen improvements in running economy and performance set against no changes in peak VO2 (Daniels et al., 1978; Krahenbuhl et al., 1989).
3.5. Key point summaryPeak VO2 can significantly improve with training in children by approximately 5%. Why children show a ‘reduced’ trainability of peak VO2 in comparison to adults is still not clear. Improvements in exercise economy, lactate threshold and performance may occur without any change in peak VO2.
4. Overtraining in Young AthletesSport development practices and structures direct children to specialize in one or two sports from an early age, especially if they seek to aspire to perform at the very highest levels. The “catch them young philosophy ”that matched the beliefs of many coaches who think that in order to achieve success at senior level it is necessary to start intensive training well before puberty (Baxter-Jones, 2007; Baxter-Jones and Mundt, 2007), has meant many of our youngsters are training intensively and for considerable hours by the time they become adolescents. This is of great importance as they are often asked to train at levels that could be considered exhaustive even for adults, but also they are assumed to be capable of withstanding the physical and psychological pressures that participation in elite sport can entail (Borms, 1986; Kentta et al., 2001). It is this success driven environment, that potentially causes the athlete to engage in very hard training without being aware if it really is of benefit for his / her own performance (Kentta et al., 2001). In addition to the long hours of intensive, repetitive training and strict dietary regimens, it is also important to consider the impact on the young athlete’s socio-cultural life; separations from the family to train or compete away from home, the impact on academic performance and the reduced opportunities to make school friends etc (Coakley, 1992; Hollander, 1995; Kentta et al., 2001; Kentta, 2001; Morgan, 1987). Consequently, the combination of heavy training, inadequate recovery and limited social support networks for the young athlete can result in overtraining in even young and aspiring elite athletes (Coakley, 1992; Kentta et al., 2001). It is therefore important that sport scientists, coaches, medics and parents start to become aware of the potential negative health implications (physical, physiological and psychological) of such training practices in young athletes (American Academy of Pediatrics, 2000).
4.1. Overtraining conceptAccording to Kreider et al. ‘s (1998) definition of overtraining, this state results from an accumulation of training and non-training stressors that has a detrimental long-term effect on performance, with a recovery period that may take several weeks or months. Overreaching, which is considered to be a normal process of training, is defined as an accumulation of training and non-training stressors that lead to a short-term decrease in performance, which can be overcome with recovery lasting days or a few weeks (Kreider et al., 1998). However overreaching may develop into overtraining if sufficient recovery time is not given, indicating that overreaching and overtraining are just two ends of the same continuum. Overtraining is fundamentally an imbalance between training fatigue and non-training stressors, and recovery. Furthermore, it is associated with a variety of symptoms that often vary considerably across individuals (Kentta et al., 2001). The latter has led to disagreements as to which signs and symptoms need to be present for an athlete to be defined as being overtrained or overreached (Meesuen et al., 2006; Uusitalo, 2006). A recent position statement by the European College of Sports Sciences tried to provide clarity to the definition by recommending that overreaching was divided into two different stages, functional overreaching and non-functional overreaching, with the latter eventually leading to the overtraining syndrome (Meesuen et al., 2006). However, the statement did not provide guidance over the duration of each stage (Uusitalo, 2006), making it hard to distinguish which state is the athlete in and also, when does functional overreaching turn into non-functional overreaching and ultimately overtraining? With disagreement over not only the presenting symptoms of but also the definition of overreaching and overtraining, the simplicity of the categorizations provided by Kreider et al., 1998 seems more useful to employ.
4.2. Overtraining in adults and young athletesOvertraining syndrome has been extensively studied in adult athletes, and therefore data on its prevalence are known. Morgan and colleagues (1988a) reported that approximately 10% of male collegiate swimmers indicated being overtrained, although rates of up to 21% have been noted in other swimmers (Hooper et al., 1995; O'Connor et al., 1989). The signs and symptoms of overtraining appeared in more than 50% of professional soccer players during a 5-month competitive season (Lehmann et al., 1992), and 33% in basketball players during a training camp of 4 weeks (Verma et al., 1992). In two separate research studies in elite US distance runners, Morgan et al. found that 60% of women and 64% of men reported being overtrained at some point in their career (Morgan et al., 1988b; Morgan et al., 1987b). Importantly, the rate of overtraining occurrence dropped to 33% when non-elite women runners were considered (Morgan et al., 1987b), which is indicative of the close relationship between performance level and the prevalence of overtraining. While overtraining is acknowledged to be of concern for athletes both in endurance and non-endurance sports (Kentta et al., 2001; Kreider et al., 1998), less data are known regarding incidence rates in individual sports compared to team sports. The few studies available report incidence ranging from 10-64% in individual sports and between 33-50% in team sports (Hooper et al., 1995; Morgan et al., 1988a; O'Connor et al., 1989).
4.3. Overtraining or depression?It has been recently recognised that the overtraining signs and symptoms are actually very similar to the ones encountered in the condition traditionally known as depression (Armstrong and VanHeest, 2002). According to the American Psychiatric Association, there are two sub-types of this condition, but concerningly the one that most closely resembles overtraining syndrome is known as major depression (Yudofsky and Hales, 1992). Major depression affects an individual’s thoughts, feelings, physical health, behaviour and ability to function on daily activities. Furthermore, it involves at least two weeks of depressed mood or lack of interest on nearly all life activities, with individuals reporting similar signs and symptoms to the ones seen in overtraining - problems with sleep, lack of appetite, irritable mood, loss of body weight, loss of motivation (Yudofsky and Hales, 1992). Finally, overtraining syndrome shares similar brain structures, endocrine pathways and immune responses to the ones reported for major depression (Morgan et al., 1987a; O'Connor et al., 1991). In fact, Morgan and colleagues (1987a) reported that approximately 80% of athletes with overtraining had a psychopathology similar to people with psychological depression. Moreover, even highly motivated athletes will have difficulties coping with the way they perceive poor performance, because of their desire to perform well and win (Coakley, 1992; Hanna, 1979). One of the reasons for the frustrations seen in these athletes who are performing badly, has to do with the pressure they put on themselves to achieve their goals (Hanna, 1979). The frustration due to the lack of performance typically drives the athlete to further increase the amount of training that he / she is doing, which just exacerbates the situation by increasing physical and emotional fatigue, with a consequent worsening in performance (Kentta et al., 2001). This cycle (process) manifests itself by changes in mood, sleep disturbances, losses in appetite and body weight, and an increase in depressive symptoms, eventually leading to depression (Armstrong and VanHeest, 2002).
4.4. Prevention of overtrainingThe limited data available suggests overtraining is prevalent in young athletes, therefore finding ways to identify those children with the condition or at risk of becoming overtrained, supported by constructive ways to treat, or better still avoid, overtraining would be invaluable. Accordingly, it seems appropriate to address this topic by taking a multidisciplinary view (Birrer and Levine, 1987; Borms, 1986; Kentta et al., 2001); we believe that psychobiologic parameterization of training can offer an effective monitoring strategy for child athletes in the detection and prevention of overtraining. Future overtraining research should try to incorporate the collection of emotional parameters questionnaires such as POMS, Training-Induced Distress scale (Raglin and Morgan, 1994), or overtraining questionnaires (Maso et al., 2004) and, at the same time collect objective physiological markers such as immunoglobulins (IgA), hormonal parameters (testosterone and cortisol), heart rate variability etc. By so doing, this will allow us to understand how variations in measures of mood correlate with physiological markers (Kentta and Hassmen, 1998), but also view the problem holistically. Treatment strategies can therefore be focused not just on physiological recuperation but also address the personal identity and emotional issues in parallel.
4.5. Key-point summaryThe limited available evidence seems to point to an occurrence of overtraining in young athletes around 30% and therefore should be investigated more extensively in the future. Overtraining and clinical major depression share many similarities, therefore overtraining should be studied by acknowledging this perspective. Collection of physiological, psychological and emotional parameters may be useful to improve our understanding of overtraining and to provide new perspectives on how to look at this important issue in child athletes.
Children’s strength, anaerobic and aerobic power is trainable, although the improvements may be smaller than seen in adults. The underlying mechanisms responsible remain to be conclusively determined, but improvements in non-invasive technologies will aid our ability to investigate these issues for children in the future. Overtraining seems to be present in some young athletes, yet we need more research to allow us to recognize the key signs / symptoms along with identifying the central determining factors to allow us to help prevent this condition arising in the first place.
1.2.1. MuscleIt is still unclear whether children’s improvements in muscle strength are accompanied by increases in muscle size. Vrijens (1978) compared strength development in prepubescent and post-pubescent boys who trained isotonically 3 times per week for eight weeks. Using roentgenography to measure muscle cross-sectional areas (mCSA), no significant changes were observed in either arm or thigh mCSA in the pre-pubertal boys (1.2% and 0.6% increments for thigh and arm muscles, respectively), whereas muscle area increased significantly for both limbs in the adolescent group (8.5% and 6.2% increments for thigh and arm muscles, respectively). The lack of change in mCSA in prepubertal children is supported by Sailors and Berg, 1987, who determined muscle size using anthropometric and somatotype techniques, but failed to show any training-induced muscle hypertrophy even though a significant increase in strength was reported. Comparable results have also been reported in adolescent females (Blimkie et al., 1993). Clearly the hormonal environment plays a key role in determining the magnitude of increase in muscle size. Although testosterone, Growth Hormone (GH), Insulin-like Growth Factor I (IGF-I) and insulin all influence muscle hypertrophy during growth and maturation, their interaction with strength training in children is not clear. It is thought that until the concentration of testosterone in particular, starts increasing at puberty, significant muscle hypertrophy is unlikely to occur with resistance training in prepubertal subjects (Vrigens, 1978). However, Mersh and Stoboy, 1989 reported significant strength-induced increases in muscle size after 10 weeks of isometric training in prepubertal boys. The trained leg increased in strength by 35% to 40% and in mCSA by 4% to 9%. Likewise, Fukunaga et al., 1992 studied 50 boys and girls aged 6.9 to 10.9 years on a 12-week resistance-training programme, which resulted in significant improvements in mCSA. This was particularly true in the older children, in whom the average increase in mCSA was 15.1% for the boys and 12.8% for the girls. Such observations suggest that although muscle hypertrophy can occur, other factors, beyond changes in muscle size, predominantly account for increases in strength during pre and early puberty (Tolfrey, 2007).
1.2.2. Neurological adaptationsLimited information is available on neural adaptations to resistance training in young athletes, but the data suggests that these play a significant role (Ozmun et al., 1994; Ramsay et al., 1990). While the hormonal influence on muscle tissue is important in developing the potential for muscular strength, neuromuscular interactions are essential for the functional development of muscle tissue (Kraemer et al., 1989; Sale, 1988). Based solely on the lack of evidence for muscle hypertrophy seen in some studies, the strength gains in preadolescent boys after resistance training have often been attributed to undefined neurological and neuromotor adaptations (Blimkie, 1992; Blimkie and Bar-Or, 1996; Sailors and Berg, 1987). Such conclusions are supported by the work of Ozmun et al., 1994, who used electromyography (EMG) to measure strength training-induced changes in neuromuscular activation. They tested 8 prepubertal boys who followed an 8-week-resistance-training programme and observed improvements in isometric and isokinetic arm strength of 22.6% and 27.8%, respectively. At the same time, EMG amplitude rose by 16.8%, supporting the possibility of improved neural activity within the muscle being trained. Another technique to assess neuromuscular changes is the twitch interpolation technique, which determines the contribution of changes in motor unit activation (MUA) to muscle force development; that is, a brief electrical stimulus is applied to the muscle in order to evoke contraction. Ramsay et al., 1990 tried to identify specifically whether changes in muscular strength were due to hypertrophy and / or neurological activity. After undertaking a resistance-training programme with 13 boys for 20 weeks the experimental group increased their strength compared to the control group; training resulted in 13.2% and 17.4% increase in MUA for elbow flexors and knee extensors, respectively. Furthermore, the same authors also assessed twitch torque (TT), a measure of intrinsic muscle strength, using the same technique; they observed improvements of approximately 30% in evoked TT on the elbow flexors and knee extensors. Since there were no corresponding increases in muscle size, the resulting data suggested an improvement in twitch-specific tension (strength per muscle cross sectional area) (Ramsay et al., 1990). In addition biomechanical factors such as changes in pennation angle of the muscle, the level of skill and motor coordination, the balance between synergist and antagonist muscle activity, enhanced stores of ATP / CP (adenosine triphosphate / phosphocreatine), improved glycolytic capacity and elevated psychological drive, all may contribute to improving strength during a training programme (Blimkie, 1992; Rowland, 2005), and therefore require further study.
1.2.3. Gender differencesMuscle hypertrophy appears to be a consistent outcome of strength training throughout adolescence in males, although in adolescent females a more variable response and smaller increases in muscle size are found. Also, the relative gains in lean mass that can accompany resistance training during adolescence and preadolescence are smaller than the relative gains in strength (Blimkie and Sale, 1998).
1.2.4. Persistence of strength gainsHow persistent are resistance training- induced strength gains in children after they stop training? Only few studies have investigated this topic on adults and even fewer in children. In adults, strength training-induced increases in muscle size and neural drive appear to decay during detraining about the same rate as they increase during training. Furthermore, detraining in adults is characterized by a relatively rapid reduction in neuromuscular activation and a more gradual reduction in muscle size (Narici et al., 1989). To date, only one study by Blimkie and colleagues (1989) looked at the effects of 8 weeks of detraining in prepubertal boys following 20 weeks of resistance training. The training-induced strength gains regressed toward the growth-adjusted control level during the detraining period, suggesting that alike with adults, training adaptations are reversible (Blimkie et al., 1989).
2.1.1. Short-term power activitiesEnhanced power output in children does occur with training Grodjinovsky et al. (1980) (cited in Tolfrey, 2007) found that mean power (MP) and peak power (PP) tested on the Wingate test, increased by 3.4% and 3.9% respectively, after sprint cycling or sprint running training. The small magnitude of gains may be attributable to the low duration of the training (15 minutes per session over 6 weeks), however other studies have also demonstrated that children can improve their anaerobic power through sprint training; MP (10%) and PP (14.2%) (Rotstein et al., 1986; Sargeant et al., 1985).
2.1.2. Changes in anaerobic power with mixed-type trainingTwelve-weeks of complex-training (using dynamic constant external resistance and plyometrics) with early pubertal boys resulted in improved anaerobic power, jumping, throwing and sprint performance, and marked improvements in dynamic strength (Ingle et al., 2006). Similarly, Sargeant et al., 1985 studied 13-year-old boys during an 8-week mixed training programme composed of short-burst activities and aerobic exercises. They observed an improvement in PP of 4.5% compared with an average 1.2% increase in the control group.
2.1.3. Changes in anaerobic power with aerobic trainingStudies dealing with the effect of aerobic training on peak power in children have generally provided concordant results. McManus and colleagues (1997) engaged 12 young girls either in an aerobic cycling programme of 8 weeks duration. They observed a 10% rise in peak VO2, yet they also saw a 20% increase in Wingate PP alongside. Although the differences in the magnitudes of the changes are reflective of the specificity of the training type, this suggests that a cross-training effect may occur in children (McManus et al., 1997). Likewise, Obert and his co-workers (2001b) also reported changes in anaerobic parameters measured on the force-velocity test after a 13-week aerobic (interval and continuous) running programme on prepubertal boys and girls. Participants improved peak power by 23%, which was mainly attributed to the increase in optimal force (17%). Finally, a 7-week aerobic running programme with 20 girls and 13 boys (training intensities of their maximal heart rate (HRmax) of 78% to 95% HRmax), was able to significantly increase maximal shuttle-running velocity (Baquet et al., 2002). Children seem therefore to be able to improve their anaerobic power whether following a dedicated anaerobic training programme or a mixed-type programme. These data help to support the inference of children being metabolic non-specialists, but care should be taken when interpreting these data since the studies used a variety of training loads (duration, intensity and frequency), employed differing methods to study anaerobic system’s responses to exercise (Wingate test, force-velocity test, field tests, non-motorised treadmill, and isokinetic dynamometer), conflicted training mode with testing mode, failed to account for changes in anthropometric variables, and mixed children of different maturities.
2.3.1. Rate-limiting enzymes and lactateFournier and colleagues (1982) studied the alterations in muscle PFK (phosphofrutokinase) and fibre area in 6 adolescent boys (16-17-years-old) after having undergone a sprint-training programme for 3 months. After obtaining pre- and post- biopsies form the vastus lateralis, no changes in muscle fibre size or percentage were reported (Fournier et al., 1982). However, PFK concentration increased 21% over and above that which could not be attributed to growth. Despite possible glycolytic enzymatic changes-with training, children do not normally show increments in maximal blood lactate levels after anaerobic exercise training. Prado, 1997 studied 12 healthy boys (age 10) and 12 adult males (age 24) in a 6-week swimming training programme (3 times per week). The training consisted exclusively in anaerobic series of 25m, 100m and a 45 second maximal swim distance. Adults improved their performance in the 25m test and the 45s test after the 6 weeks, whereas no effect of training was observed on the boys. Maximal lactate concentrations were significantly lower in children than adults, but no changes were observed in maximal lactate with training within either group. Such findings do however cast doubt on the appropriateness of using maximal lactate as a surrogate measure for glycolytic activity.
2.3.2. Muscle massSargeant et al., 1985 observed significantly greater improvements in lean body mass (4.8% increase), and upper leg muscle (9.7% increase) in the experimental group compared to the controls after 8-weeks of mixed training; indicating the importance of muscle mass in explaining training induced improvements in power output. Thigh muscle volume has been shown to be the strongest significant explanatory variable of PP in 12 to 14-year-old boys and girls (Santos et al., 2003), but qualitative muscular and neurological factors are also implicated (Obert et al., 2001a).
2.3.3. Fibre typeA study involving 11-13-year old Swedish boys who participated in a 4-year sprint-training programme investigated the link between anaerobic power and muscle morphological / histochemical adaptations. Improved muscular endurance (50 all-out repetitions of isokinetic knee extension) were associated with an increase in mCSA, even though no changes in muscle fibre-type composition occurred (Jacobs et al., 1982). In another study addressing muscle fibre characteristics and physical performance in athletic boys (sprinters, weight lifters and tennis players) concluded that the size and morphology of muscle fibre type are related to and influenced by the characteristics of the sports being performed (Mero et al., 1991).
2.3.4. Imaging techniquesThe use of phosphorus nuclear magnetic resonance spectroscopy (31P-NMRS) as a non-invasive technique to measure intracellular inorganic phosphate (Pi), PC, ATP, allows us a possible insight into muscular glycolytic activity. Kuno and colleagues (1995) reported a similar PC/(PC+Pi) ratio between untrained and trained 12 to 17-year-old adolescents after performing exhaustive exercise. However, the same ratio was higher when compared to adults, reflecting a ‘poorer’ anaerobic response in young populations (Kuno et al., 1995). However this conclusion has been challenged by recent findings in which a group of prepubertal and pubertal female synchronized-swimmers had their glycolytic responses to short-term high-intensity exercise assessed in their gastrocnemius and soleus muscles using 31P-NMRS. The authors found no significant differences for pH values or Pi/PC ratio and stated that the glycolytic system of physically active children appeared to be independent of maturity (Peterson et al., 1999).
3.1.1. Training intensityIs seems that children need to train at a higher exercise intensity to elicit increases in aerobic fitness than for adults. Heart rate has normally been used as a marker of training intensity, with recommended intensities between 60-90% of HRmax, or 60% of HR reserve (difference between resting and maximal HR) for adults. However, the same target heart rates do not seem to be effective in children as a sufficient exercise intensity is a necessary stimulus in order to see improvements in peak VO2 (Rowland, 2005). A study by Massicot and MacNab (1974) involving three separate groups of nine boys (11-13 years) set different training intensities for each group. Participants trained at heart rates of 130-140, 170-180, and 150-160 beats·min-1, and were compared to a matched control group. After having been matched for baseline peak VO2 and then randomly assigned to the four experimental groups, the boys undertook six weeks of aerobic training. The results showed that only the boys who exercised at the highest intensities (above 170 bts·min-1 - at least 88% HRmax) showed an 11% increase in peak VO2. Indeed as long as the training intensity is high enough, improvements have been seen in both prepubertal and adolescent children., Baquet and colleagues (2002) looked at the effects of a 7-week (twice per week) high intensity intermittent training programme on peak VO2 of prepubertal boys and girls. Peak VO2 increased by 8.2% compared with a 1.9% reduction in the 20 maturity-matched control participants. The results demonstrated that prepubertal children can increase peak VO2 with high intensity aerobic exercise. Also, the fact that children only exercised two times during the week comes to reinforce the importance of intensity as a major stimulus for peak VO2 improvements.
3.1.2. Sex differencesAt present, there are few studies, which have addressed sex differences in the trainability of aerobic fitness in children, with the majority of studies recruiting boys as participants. Single sex studies using girls however have reported that significant improvements in peak VO2 are possible (McManus et al., 1997), akin to that seen in boys. The data from those studies that have directly compared the sexes suggest that boys and girls demonstrate similar responses in aerobic trainability (Baquet et al., 2003; Baxter-Jones et al., 1993), even though boys’ peak VO2 appears to be slightly higher than girls (Naughton et al., 1998; Obert et al., 2003). Obert et al., 2003 had 10 girls and 9 boys participate in a 13-week endurance training programme (3 X 1 hour per week, intensity: 80%HRmax) and found that significant improvements in peak VO2 were observed for both groups; boys increased peak VO2 by 15% and girls by 8%. Similar findings were reported by Baquet et al., 2002; 9.5% increase in peak VO2 for the prepubertal boys being not significantly different from the 7.2% increment observed in girls.
3.3.1. Circulating testosteroneAdult data indicates that changes in the concentrations of hormones such as GH, IGF-I, testosterone, oestrogen are responsible for the anabolic effects seen with exercise training. It is not clear however how these hormones are affected by endurance training in children with much of the information being extrapolated from studies with adults and animals (Boisseau and Delamarche, 2000). It appears however that testosterone concentration is markedly up regulated with training in children; sex-related increases in VO2, muscle size and strength, and maximal arterio-venous oxygen (A-VO2) difference, seem to be related with pubertal increases in testosterone. The time when testosterone starts increasing during puberty matches the age when aerobic trainability improves significantly (Rowland, 2005). Mero and colleagues (1990) measured resting serum testosterone, before, during and after a year of training in 11-12-year-old boys. The boys engaged in different activities like long distance running, sprint running, tennis and weight lifting. After the training period testosterone was almost 3 times higher in the training group compared to the untrained boys, and the mean testosterone level had approximately doubled after a year of training. It was concluded that the increases in physical performance and fitness were a direct result of the training induced enhanced testosterone concentration even in early puberty (Mero et al., 1990). Furthermore, there is evidence showing that testosterone levels can rise during acute bouts of exercise in adults, although not in prepubertal subjects (Fahey et al., 1979).
3.3.2. Cardiac changesThe cardiovascular characteristics of young adult endurance athletes have been well described. These athletes typically show a peak VO2, which is approximately twice as large compared to the sedentary population, a reflection of a superior cardiac output (CO) resulting from a greater left ventricular size and consequent larger SV (Faria et al., 1989; Wolfe et al., 1986). Intervention studies confirm that SV and ventricular size are eminently trainable in adults, with SV increasing by 10-30% with exercise training (DeMaria et al., 1979; Stratton et al., 1994; Wilmore et al., 2001). An increase in peak VO2 in adults is related to both central (increased maximal CO) and peripheral adaptations in A-VO2 difference (Wilmore et al., 2001). In children, however, less information is available on this topic. Cross-sectional studies of trained and untrained children suggest that cardiac size and function, thus ultimately SV, are greater in trained children. Nottin and colleagues (2002) found higher SV values in the trained cyclists compared to controls and stated that these increases were influenced by factors such as cardiac hypertrophy, augmented cardiac relaxation and possibly expanded blood volume. Furthermore, similar findings have been reported in trained versus untrained distance runners (Rowland et al., 1998). Intervention studies also indicate that cardiac size and function are modifiable with training in children. Early work by Erickson and Koch (1973), using dye dilution technique, measured the cardiovascular responses of prepubertal boys (11-13-years-old) to 16 weeks of training. The authors observed decreases in resting HR, increases in heart size and peak VO2 at the end of the programme; the latter related to the increase in SV rather than through an increased A-VO2 difference (Eriksson and Koch, 1973). In agreement with earlier work, a recent study looking at cardiac adaptations after a 13-week endurance training programme in prepubertal boys and girls (10.5 ± 0.3 years), saw that peak VO2 increased by 15% and 8% in the boys and girls respectively. The authors reported significant increments in SV (boys: +15%; girls: +11%) in both sexes, with this being the strongest explanatory variable for the increases in peak VO2 (Obert et al., 2003). Testosterone affects aerobic fitness by affecting changes in heart size (Janz et al., 2000). It has been shown that during puberty, the size of the left ventricle in males increases at a faster rate than it does in females (Hayward et al., 2001), which could work as an advantage for males during exercise by enabling increases in SV (stoke volume) and end-diastolic volume, and consequently CO (cardiac output). With no difference in maximal A-VO2 difference being reported in either cross-sectional comparisons or intervention studies in children, this indicates that central factors rather than peripheral usage may determine training induced adaptations in aerobic fitness. However it appears that the magnitude of change seen in cardiac factors is slightly lower than seen in adults and may contribute to the reduced aerobic trainability of children.
3.3.3. Aerobic enzymesIn adults, improvements in performance, lower submaximal exercise lactate production, and greater reliance on fat metabolism due to endurance training seem to be associated with an increase in mitochondrial numbers within the skeletal muscle (Holloszy and Coyle, 1984). In one of the few studies in children to assess the changes in oxidative enzymes with aerobic training, Eriksson et al., 1973 looked at the effects 6 weeks of endurance training on muscle metabolic profile in 5 prepubertal boys (11-13 years old). Via a muscle biopsy, the authors observed an increase in the concentration of the oxidative enzyme, succinicate dehydrogenase (SDH) of 30% post training. Work by the same authors in adults, reported that endurance athletes show greater levels of SDH (50% higher) when compared to sedentary people (Eriksson et al., 1973). Moreover, it has been reported that the concentration of another aerobic enzyme, isocitric dehydrogenase (ICDH), is higher in children than typically found in adults and also that there is a lower ratio of ICDH to PFK (Haralambie, 1982), suggesting that children might be preferentially adapted to aerobic metabolism. Arguably this may leave less scope to improve the aerobic enzymatic profile of the muscle with training potentially explaining the blunted training effect in children, but with such little data derived from limited numbers of children this has to remain speculation.
4.2.1. Is overreaching and overtraining prevalent in young athletes?Very few investigations have been directed to young athletes and therefore, it is not known whether similar responses to chronic over-exercising in adults are evident in children. Recently a study involving adolescent swimmers (13-18 year old) looked at the prevalence of overtraining across different countries (Japan, USA, Sweden and Greece) and found that 35% had been overtrained at least once (Raglin et al., 2000). Kentta and colleagues (2001) observed higher incidence rates for individual sports (48%) compared with team sports (30%) and less physically demanding sports (18%). In addition, in 1992, Coakley identified 15 adolescents who had been overtrained during their careers (ages ranged from 15 to 19 years); the author concluded overtraining was grounded in the social organization of sport and recommended changes in the organization of high performance sports; Also psychodoping was acknowledged as a social problem that restricts athletes from creating a “healthy ”identity due to their lack of control over their own lives (Coakley, 1992).
4.2.2. Signs and symptoms in adult athletesA considerable number of studies have investigated the common signs and symptoms reported in athletes from a range of different sports. Fry et al.’s (1991) review listed more than 90 different symptoms that are reported by overtrained athletes. Unfortunately, such a long list makes it difficult for a coach or an athlete to know which signs or symptoms to be concerned about, but further complicating the picture is the large interindividual variability found within athletes practicing the same sport (Verma et al., 1992). One of the main complaints from athletes is a decrease in performance (Budget, 1998), coupled with chronic fatigue and apathy (Armstrong and VanHeest, 2002), which will often be ignored. Nevertheless, the responses of athletes to overtraining have essentially been measured from 2 categories of symptoms: physiological and psychological. Several physiological markers have been proposed as important and potential parameters for the diagnose of overtraining such as: decreased or suppressed levels of immunoglobulin A (IgA) (Gleeson et al., 1999; Gleeson and Pyne, 2000; Ring et al., 2005), decreased salivary testosterone to cortisol ratio (Passelergue and Lac, 1999), increased salivary cortisol (O'Connor et al., 1989), decreased heart rate variability (Hedelin et al., 2000; Pichot et al., 2002), increased catecholamines (Hooper et al., 1995). However, some of the parameters, like hormonal markers, should not be used as a single measure, since they show a very high variability (Urhausen and Kindermann, 2002). As example, it has been proposed that a decrease in cortisol levels occurs in the more chronic state of overtraining (Lehmann et al., 1998), whereas an increase would represent an acute higher physiological strain (Kirwan et al., 1988). There is unfortunately no single practical, valid and reliable physiological marker that can be used to enable a clear and quick diagnoses of athletes who are entering this state (Kentta et al., 2001). Nevertheless, some symptoms appear to be more frequently reported than others; such as a higher incidence in infectious disorders (upper respiratory tract infections), loss of appetite, unexpected weight loss, sleep disturbances and emotional disturbances (Armstrong and VanHeest, 2002; Fry et al., 1991; Morgan et al., 1987a; Raglin et al., 2000).
4.2.3. Signs and symptoms in young athletesAlthough few investigations have collected data on athletes in regards to overtraining, there is some evidence suggesting that the signs and symptoms in young athletes are typically the same or similar to the ones found in the adult population. The mentioned cross-cultural study performed on swimmers found that the highest complaint from swimmers was primarily an increased perception of effort, followed by feelings of heaviness (Raglin et al., 2000), which resembles the results from another study on young elite athletes (Kentta et al., 2001). Also, swimmers who reported to be unmotivated had higher levels of mood disturbances at all assessment points compared with overtrained swimmers who retained their incentive to train (Raglin et al., 2000). The study by Raglin and colleagues (2000) further reported more symptoms associated with overtraining like muscle soreness, sleep disturbances and loss of appetite. Furthermore, athletes were also asked to report additional symptoms during the overtrained periods; they found these to be essentially of psychosocial nature, i.e. social problems (family, boyfriend / girlfriend, coach or friends), negative feelings like decreased interest in training and competition and frustration to continue training. Finally, many other psychological problems have also been described like athletes’ decreased self-confidence and ability to focus, short temper, heightened levels of irritability, depression, sadness, and elevated levels of perceived stress (Kentta et al., 2001). Since 20 years of research on overtraining indicates that objective physiological markers have generally not proven useful to monitor training and performance, therefore the need to increase athletes’ self-awareness, i.e. making them more aware of physical or psychological stress has been highlighted (Kentta and Hassmen, 1998; Kentta et al., 2001). This latter issue is important as clinical depression has been reported in overtrained athletes (Hooper et al., 1995; Kentta et al., 2006; Morgan et al., 1987a; 1988a. In regard to the psychological factors associated with overtraining in youth sports, the use of the POMS (profile of mood states) scale that assesses relevant emotional shifts in tension, depression, anger, vigour, fatigue and confusion has proven useful. As overtraining progresses the deterioration of positive moods and the increase in negative moods can clearly be tracked by POMS. Furthermore, the necessity to increase the awareness of youth sport coaches about this issue has been stressed elsewhere (Hollander et al., 1995). Likewise, parents can unduly pressurize their child by overemphasizing winning, holding unrealistic expectations, criticizing them and pushing them to play. All of these factors reiterate the need for parents to be educated about their critical role in their child’s sporting development (Gould and Eklund, 1991).
|
AUTHOR BIOGRAPHY |
 |
Nuno Matos |
Employment: PhD student at the Children’s Health and Exercise Research Centre. |
Degree: BSc, MSc |
Research interests: Overtraining in young athletes. |
E-mail: N.F.Matos@ex.ac.uk |
|
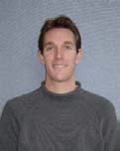 |
Richard J. Winsley |
Employment: Senior Lecturer and an Associate Director of the Children’s Health and Exercise Research Centre. |
Degree: BA(Ed), PhD |
Research interests: Overtraining in young athletes and children’s cardiac function. |
E-mail: R.J.Winsley@exeter.ac.uk |
|
|
|
REFERENCES |
 American Academy of Paediatrics. (1976) Fitness in the preschool child. Pediatrics 1, 88-89.
|
 American Academy of Pediatrics (2000) Intensive training and sports specialization in young athletes. Pediatrics 106, 154-157.
|
 Armstrong L., VanHeest L. (2002) The unknown mechanism of the overtraining syndrome - clues from depression and psychoneuroimmunology. Sports Medicine 32, 185-209.
|
 Armstrong N., Welsman J. (2002) Young people and physical activity. Oxford. Oxford University Press.
|
 Armstrong N., Welsman J.R., Lee M. (1993) Coaching children in sport. Training young athletes. London. Spon.
|
 Astrand P., Rodahl K., Dahl H., Stromme S. (2003) Textbook of work physiology: Physiological bases of exercise. Champaign, IL. Human Kinetics.
|
 Baquet G., Berthoin S., Dupont G., Blondel N., Fabre C., Van Praagh E (2002) Effects of high intensity intermittent training on peak VO2 in prepubertal children. International Journal of Sports Medicine 23, 439-444.
|
 Baquet G., Van-Praagh E., Berthoin S. (2003) Endurance training and aerobic fitness in young people. Sports Medicine 33, 1127-1143.
|
 Barnett C., Carey M., Proietto J., Cerin E., Febbraio M., Jenkins D. (2004) Muscle metabolism during sprint exercise in man: Influence of sprint running. Journal of Science and Medicine in Sport 7, 314-322.
|
 Baxter-Jones A., Helms P., Maffulli N., Baines-Preece J., Preece M. (1995) Growth and development of male gymnasts, swimmers, soccer and tennis Players: A longitudinal study. Annals of Human Biology 22, 381-394.
|
 Baxter-Jones A., Mundt C., Armstrong N. (2007) Paediatric exercise physiology - Advances in sport and exercise science series. The young athlete. Philadelphia. Churchill Livingston Elsevier.
|
 Baxter-Jones A.G., Helms P. (1993) The development of aerobic power in young athletes. Journal of Applied Physiology 75, 1160-1167.
|
 Bencke J., Damsgaard R., Saekmose A., Jorgensen P., Jorgensen K., Klausen K. (2002) Anaerobic power and muscle strength characteristics of 11 year old elite and non-elite boys and girls from gymnastics, team handball, tennis and swimming. Scandinavian Journal of Medicine and Science in Sports 12, 171-178.
|
 Birrer R.B., Levine R. (1987) Performance parameters in children and adolescent athletes. Sports Medicine 4, 211-27.
|
 Blimkie C. (1992) Resistance training during pre- and early puberty: efficacy, trainability, mechanisms and persistence. Canadian Journal of Sports Science 17, 264-279.
|
 Blimkie C., Bar-Or O., Bar-Or O. (1996) The child and adolescent athlete. Trainability of muscle strength, power and endurance during childhood. Oxford. Blackwell Science.
|
 Blimkie C., Martin J., Ramsay J., Sale D., Macdougall D. (1989) The Eefects of detraining and maintenance weight training on strength development in prepubertal boys. Canadian Journal of Sports Science 14, 102-.
|
 Blimkie C., Rice S., Webber C. (1996) Effects of resistance training on bone mineral content and density in adolescent females. Canadian Journal of Physiology and Pharmacology 74, 1025-1033.
|
 Blimkie C., Rice S., Webber C., Martin J., Levy D., Gordon C. (1993) Effects of resistance training on bone mass and density in adolescent females. Medicine and Science in Sports and Exercise 25, 183-197.
|
 Blimkie C., Sale D., Van Praagh E. (1998) Pediatric anaerobic performance. Strength development and trainability during childhood. Champaign. Human Kinetics.
|
 Boisseau N., Delamarche P. (2000) Metabolic and hormonal responses to exercise in children and adolescents. Sports Medicine 30, 405-422.
|
 Borms J. (1986) The child and exercise: an overview. Journal of Sports Science 4, 3-20.
|
 Bosh A. (2006) Exercise science and coaching: Correcting common misunderstandings about endurance exercise. International Journal of Sports Science and Coaching 1, 77-87.
|
 Bouchard C., Dionne F., Simoneau J., Boulay M. (1992) Genetics of aerobic and anaerobic performances. Exercise and Sport Science Reviews 20, 27-58.
|
 Budget R. (1998) Fatigue and underperformance in athletes: The overtraining syndrome. British Journal of Sports Medicine 32, 107-110.
|
 Chilibeck P., Calder A., Sale D. (1998) A comparison of strength and muscle mass increases during resistance training in young women. European Journal of Applied Physiology and Occupational Physiology 77, 170-175.
|
 Coakley J. (1992) Burnout among adolescent athletes: A personal failure or social problem?. Sociology of Sport Journal 9, 271-285.
|
 Coutts A., Murphy A., Dascombe B. (2004) Effect of direct supervision of a strength coach on measures of muscular strength and power in young rugby league players. Journal of Strength and Conditioning Research 18, 316-323.
|
 Coyle E. (2005) Improved muscular efficiency displayed as Tour de France champion matures. Journal of Applied Physiology 98, 2191-2196.
|
 Daniels J., Oldridge N., Nagle F., White B. (1978) Differences and changes in VO2 among young runners 10 to 18 years of age. Medicine and Science in Sports and Exercise 10, 200-203.
|
 Danis A., Kyriazis Y., Klissouras V. (2003) The effect of training in male prepubertal and pubertal monozygotic twins. European Journal of Applied Physiology 89, 309-318.
|
 DeMaria A.N., Neumann A., Schubart P., Lee G., Mason D.T. (1979) Systematic correlation of cardiac chamber size and ventricular performance determined with echocardiography and alterations in heart rate in normal persons. American Journal of Cardiology 43, 1-9.
|
 Eriksson B., Gollnick P., Saltin B. (1973) Muscle metabolism and enzyme activities after training in boys 11-13 years old. Acta Physiologica Scandinavia 87, 485-497.
|
 Eriksson B., Koch G. (1973) Effect of physical training on hemodynamic response during submaximal and maximal exercise in 11-13 Year Old Boys. Acta Physiologica Scandinavia 87, 27-39.
|
 Fahey T.D., Del Valle-Zuris A., Oehlsen G., Trieb M., Seymour J. (1979) Pubertal stage differences in hormonal and hematological responses to maximal exercise in males. Journal of Applied Physiology 46, 823-827.
|
 Faigenbaum A (2000) Strenth training for children and adolescents. Clinics in Sports Medicine 19, 593-619.
|
 Falk B., Tenembaum G. (1996) The effectiveness of resistance training in children. Sports Medicine 22, 176-186.
|
 Faria I.E., Faria E.W., Roberts S., Yoshimura D. (1989) Comparison of physical and physiological characteristics in elite young and mature cyclists. Research Quaterly for Exercise and Sport 60, 388-95.
|
 Faust M. (1977) Somatic development of adolescent girls. Monographs of the Society for Research in Child Development 12, 3-7.
|
 Fleck S., Falkel J. (1986) Value of resistance training for the reduction of sports injuries. Sports Medicine 3, 61-68.
|
 Fournier M., Ricci J., Taylor A., Ferguson R., Montpetit R., Chaitman B. (1982) Skeletal muscle adaptation in adolescent boys: Sprint and endurance training and detraining. Medicine and Science in Sports and Exercise 14, 453-456.
|
 Fry R., Morton A., Keast D. (1991) Overtraining in athletes - An update. Sports Medicine 12, 32-65.
|
 Fukunaga T., Funato K, Ikegawa S. (1992) The effects of resistance training on muscle area and strength in prepubertal age. Annals of Physiology and Anthropology 11, 357-364.
|
 Gleeson M., McDonald W., Pyne D., Cripps A., Francis L., Fricker P., Clancy R. (1999) Salivary IgA levels and infection risk in elite swimmers. Medicine and Science in Sports 31, 67-73.
|
 Gleeson M., Pyne D. (2000) Exercise effects on mucosal immunity. Immunology and Cell Biology 78, 536-544.
|
 Gould D., Eklund R. (1991) The application of sport psychology for performance of optimization. Thai Journal of Sports Science 1, 10-21.
|
 Haffor A., Harrison A., Kirk P. (1990) Anaerobic threshold alterations caused by interval training in 11-year-olds. Journal of Sports Medicine and Physical Fitness 30, 53-56.
|
 Hanna E.A. (1979) Potential sources of anxiety and depression associated with athletic competition. Canadian Journal of Applied Physiology 4, 199-204.
|
 Haralambie G. (1982) Enzyme activities in skeletal muscle of 13-15 years old adolescents. Bulletin Européen Pyhsiopathologie Respiratoire 18, 65-74.
|
 Hawley J., Williams M. (1991) Relationship between upper body anaerobic power and freestyle swimming performance. International Journal of Sports Medicine 12, 1-5.
|
 Hayward C., Webb C., Collins P. (2001) Effects of sex hormones on cardiac Mass. Lancet 357, 1354-1356.
|
 Hedelin R., Kentta G., Wiklund U., Bjerle P., Henriksson-Larsen K. (2000) Short-term overtraining: effects on performance, circulatory responses, and heart rate variability. Medicine and Science in Sports and Exercise 32, 1480-1484.
|
 Hejna W., Rosenberg A. (1982) The Prevention of sports injuries in high school students through strength training. National Strength Coaches Association Journal 4, 28-31.
|
 Hoffman J. (2002) Physiological aspects of sport training and performance. Champaign. Human Kinetics.
|
 Hollander D., Meyers M., LeUnes A. (1995) Psychological factors associated with overtraining: implications for youth sport coaches. Journal of Sport Behaviour 18, 3-17.
|
 Holloszy J., Coyle E. (1984) Adaptations of skeletal muscle to endurance exercise and their metabolic consequences. Journal of Applied Physiology Respiratory Environmental & Exercise Physiology 56, 831-838.
|
 Hooper S., Mackinnon L., Howard A., Gordon R., Bachman A. (1995) Markers for monitoring overtraining and recovery. Medicine and Science in Sports and Exercise 27, 106-112.
|
 Ikai M., Fukunaga T. (1970) A study on training effect on strength per unit cross-sectional area of muscle by means of Ultrasonic measurement. Intern Z Angew Physiologica 28, 173-80.
|
 Ingle L., Sleap M., Tolfrey K. (2006) The effect of complex training and detraining programme on selected strength and power variables in early pubertal boys. Journal of Sports Science 24, 987-997.
|
 Jacobs I., Sjodin B., Svanne B. (1982) Muscle fibre type, cross-sectional area and strength in boys after 4 years’ endurance training. Medicine and Science in Sports 14, 123-.
|
 Janz K., Dawson J., Mahoney L. (2000) Predicting heart growth during puberty :The Muscatine study. Pediatrics 105, E63-.
|
 Jones A. (2006) The physiology of the world record holder for the Women’s marathon. International Journal of Sports Science and Coaching 1, 101-116.
|
 Jones A., Carter H. (2000) The effect of endurance training on parameters of aerobic fitness. Sports Medicine 29, 373-86.
|
 Katch V. (1983) Physical conditioning of children. Journal of Adolescence Health Care 3, 241-246.
|
 Kentta G., Hassmen P. (1998) Overtraining and recovery - A conceptual model. Sports Medicine 26, 1-16.
|
 Kentta G., Hassmen P., Raglin J. (2001) Training practices and overtraining syndrome in Swedish age-group athletes. International Journal of Sports Medicine 22, 460-465.
|
 Kentta G., Hassmen P., Raglin J. (2006) Mood state monitoring of training and recovery in elite Kayakers. European Journal of Sports Science 6, 245-253.
|
 Kirwan J.P., Costill D.L., Flynn M.G., Mitchell J.B., Fink W.J., Neufer P.D., Houmard J.A. (1988) Physiological responses to successive days of intense training in competitive swimmers. Medicine and Science in Sports and Exercise 20, 255-259.
|
 Knuttgen H., Kraemer W. (1987) Terminology and measurements in exercise performance. Journal of Applied Sports Science Research 1, 1-10.
|
 Kobayashi K., Kitamura K., Miura M., Sodeyama H., Murase Y., Miyashita M., Matsui H. (1978) Aerobic power as related to body growth and training in Japanese boys: A longitudinal study. Journal of Applied Physiology 44, 666-672.
|
 Kraemer W., Fry A., Frykman P., Conroy B., Hoffman J. (1989) Resistance training in youth. Pediatric Exercise Science 1, 336-350.
|
 Krahenbuhl G., Morgan D., Pangranzi R. (1989) Longitudinal changes in distance-running performance of young males. International Journal of Sports Medicine 10, 92-96.
|
 Kreider R., Fry A., O'Toole M. (1998) Overtraining in Sport. Champaign. Human Kinetics.
|
 Kuno S., Takahashi H., Fujimoto K. (1995) Muscle metabolism during exercise using phosphorus31 Nuclear Magnetic Resonance Spectroscopy in adolescents. European Journal of Applied Physiology 70, 301-304.
|
 Lehmann M., Foster C., Dickhuth H.H., Gastmann U. (1998) Autonomic imbalance hypothesis and overtraining syndrome. Medicine and Science in Sports and Exercise 30, 1140-1145.
|
 Lehmann M., Schnee W., Scheu R., Stockhausen W., Bachl N. (1992) Decreased nocturnal catecholamine excretion: Parameter for an overtraining syndrome in athletes?. International Journal of Sports Medicine 13, 236-242.
|
 Lehnhard R., Lehnhard H., Young R. (1996) Monitoring injuries on a College coccer team: The effect of strength training. Journal of Strength and Conditioning Research 10, 115-119.
|
 Magel J., Guido F., McArdle W., Gutin B., Pechar G., Katch F. (1974) Specificity of swim training on maximum oxygen uptake. Journal of Applied Physiology 38, 151-155.
|
 Mahieu N., Witvrouw E., Van de Voorde D., Michildsens D., Arbyn V., Vvan den Broecke W. (2006) Improving strength and postural control in young skiers: Whole-body vibration versus equivalent resistance training. Journal of Athletic Training 41, 286-293.
|
 Malina R., Bouchard C. (1991) Growth, maturation and physical Activity. Champaign. Human Kinetics.
|
 Maso F., Lac G., Filaire E., Michaux O., Robert A. (2004) Salivary testosterone and cortisol in rugby players: correlation with psychological overtraining items. British Journal of Sports Medicine 38, 260-263.
|
 Massicotte D.R., Macnab R.B. (1974) Cardiorespiratory adaptations to training at specified intensities in children. Medicine and Science in Sports and Exercise 6, 242-246.
|
 McArdle W., Katch F., Katch V., McArdle W, Katch F.V. (2000) Essentials of Exercise Physiology. Training the anaerobic and aerobic energy systems. Philadelphia. K. Lippincott Williams & Wilkins.
|
 McManus A., Armstrong N., Williams C. (1997) Effect of training on the aerobic power and anaerobic performance of prepubertal girls. Acta Paediatrica 86, 456-459.
|
 Meesuen R., Duclos M., Gleeson M., Rietjens G., Steinacker J., Urhausen A. (2006) Prevention, diagnosis and treatment of the overtraining syndrome. European Journal of Sports Science 6, 1-14.
|
 Mero A., Jaakkola L., Komi P. (1990) Serum hormones and physical performance capacity in young boy athletes during a 1 year training period. European Journal of Applied Physiology 60, 32-37.
|
 Mero A., Jaakkola L., Komi P. (1991) Relationships between muscle fibre characteristics and physical performance capacity in trained athletics boys. Journal of Sports Science 9, 161-171.
|
 Mersh F., Stoboy H., Oseid S, Carlsen K. (1989) Children and Exercise XIII. Strength training and muscle hypertrophy in children. Champaign. Human Kinetics.
|
 Morgan W., Brown D., Raglin J., O'Connor P., Ellickson K. (1987a) psychological monitoring of overtraining and staleness. British Journal of Sports Medicine 21, 107-114.
|
 Morgan W., Costill D., Flynn M., Raglin J., O'Connor P. (1988a) Mood disturbance followed increased training in swimmers. Medicine and Science in Sports and Exercise 20, 408-414.
|
 Morgan W., O'Connor P., Ellickson K., Bradley P. (1988b) Personality structure, mood states, and performance in elite male distance runners. International Journal of Sports Medicine 19, 247-263.
|
 Morgan W.P., O'Connor P.J., Sparling P.B., Pate R.R. (1987b) Psychological characterization of the elite female distance runner. International Journal of Sports Medicine 8, 124-131.
|
 Moritani T., DeVries H. (1980) Potential for gross hypertrophy in older man. Journal of Gerontology 35, 672-682.
|
 Myer G., Wall E. (2006) Resistance training in the young athlete. Operative Techniques in Sports Medicine 14, 218-230.
|
 Narici M., Roi G., Landoni L., Minetti A., Cerreteli P. (1989) Changes in force, cross-sectional area and neural activation during strength training and detraining of the human quadriceps. European Journal of Applied Physiology 59, 310-319.
|
 Naughton G, Carlson J, Iuliano S (1998) Cardiorespiratory responses and circulating metabolite concentrations in male and female adolescents during a simulated duathlon. International Journal of Sports Medicine 19, 303-309.
|
 Naughton G., Farpour L., Carlson J., Bradney M., Van Praagh E. (2000) Physiological issues surrounding the performance of adolescent athletes. Sports Medicine 30, 309-325.
|
 Nevill A., Holder R., Baxter-Jones A., Round J., Jones D. (1998) Modeling developmental changes in strength and aerobic power in children. Journal of Applied Physiology 84, 963-970.
|
 Nielson B., Nielson K., Behrendt-Hansen M., Asmussen E., Berg K, Eriksson B. (1980) Children and Exercise IX. Training of functional muscular strength in girls 7-19 years old. Champaign. Human Kinetics.
|
 Nottin S., Vinet A., Stecken F., N'Guyen L.D., Ounissi F., Lecoq A.M., Obert P. (2002) Central and peripheral cardiovascular adaptations to exercise in endurance-trained children. Acta Physiologica Scandinavia 175, 85-92.
|
 O'Connor P., Morgan W., Raglin J., Barksdale C., Kalin N. (1989) Mood state and salivary cortisol levels following overtraining in female swimmers. Psychoneuroendocrinology 14, 303-310.
|
 O'Connor P.J., Morgan W.P., Raglin J.S. (1991) Psychobiologic effects of 3 d of increased training in female and male Swimmers. Medicine and Science in Sports and Exercise 23, 1055-61.
|
 Obert P., Mandigout S., Nottin S., Vinet A., N'Guyen D., Lecoq A. (2003) Cardiovascular responses to endurance training in children: Effect of gender. European Journal of Clinical Investigation 33, 199-208.
|
 Obert P., Mandigout S., Vinet A., Courteix D. (2001a) Effect of a 13-week aerobic training programme in the maximal power developed during a force-velocity test in prepubertal boys and girls. International Journal of Sports Medicine 22, 442-446.
|
 Obert P., Mandigout S., Vinet A., N'Guyen L., Stecken F., Courteix D. (2001b) Effect of aerobic training and detraining on left ventricular dimensions and diastolic function in prepubertal boys and girls. International Journal of Sports Medicine 22, 90-96.
|
 Ozmun J., Mikesky A., Surburg P. (1994) Neuromuscular adaptations following prepubescent strength training. Medicine and Science in Sports 26, 510-514.
|
 Passelergue P., Lac G. (1999) Saliva cortisol, testosterone and T/C ratio variations during a wrestling competition and during the post-competitive recovery period. International Journal of Sports Medicine 20, 109-113.
|
 Payne V., Morrow J., Johnson L., Dalton S. (1997) Resistance training in children and youth: Meta-analysis. Research Quaterly for Exercise and Sport 68, 80-88.
|
 Pediatrics A.A. (1990) Committee on sports medicine: Strength training, weight and power lifting, and body Builiding by children and adolescents. Pediatrcsc 86, 801-803.
|
 Peterson S., Gaul C., Stanton M., Hanstock C. (1999) Skeletal muscle metabolism during short-term, high-intensity exercise in prepubertal and pubertal Girls. Journal of Applied Physiology 87, 2151-2156.
|
 Pichot V., Busso T., Roche F., Garet M., Costes F., Duverney D., Lacour J., Barthélémy J. (2002) Autonomic adaptations to intensive and overload training periods: A laboratory study. Medicine and Science in Sports 34, 1660-1666.
|
 Prado L., Armstrong N, Kirby B, Welsman J. (1997) Children and Exercise XIX. Lactate, ammonia and cathecholamine metabolism after anaerobic training. London. Spon.
|
 Raglin J., Sawamura S., Alexiou S., Hassmén P., Kentta G. (2000) Training practices and staleness in 13-18-year-old swimmers: A cross-cultural study. Pediatric Exercise Science 12, 61-70.
|
 Raglin J.S., Morgan W.P. (1994) Development of a scale for use in monitoring training-induced distress in athletes. International Journal of Sports Medicine 15, 84-88.
|
 Ramsay J., Blimkie C., Smith K., Garner S., MacDougall J., Sale D. (1990) Strength training effects in prepubescent boys. Medicine and Science in Sports 22, 605-614.
|
 Ring C., Carroll D., Hoving J., Ormerod J., Harrison L., Drayson M. (2005) Effects of competition, exercise, and mental stress on secretory immunity. Journal of Sports Science 23, 501-508.
|
 Rotstein A., Dotan R., Bar-Or O., Tenembaum G. (1986) Effects of training on anaerobic threshold, maximal aerobic power and anaerobic performance of preadolescent boys. International Journal of Sports Medicine 7, 281-286.
|
 Rowland T. (1985) Aerobic response to endurance training in prepubescent children: A critical analysis. Medicine and Science in Sports and Exercise 17, 493-497.
|
 Rowland T. (2005) Children’s Exercise Physiology. Champaign. Human Kinetics.
|
 Rowland T., Goff D., Popowski B., DeLuca P., Ferrone L. (1998) Cardiac responses to exercise in child distance runners. International Journal of Sports Medicine 19, 385-390.
|
 Sailors M, , Berg K. (1987) Comparison of responses to weight training in pubescent boys and men. Journal of Sports Medicine 27, 30-37.
|
 Sale D. (1988) Neural adaptations to resistance training. Medicine and Science in Sports 20, S135-S145.
|
 Santos A., Armstrong N., De St Croix M., Sharp P., Welsman J. (2003) Optimal peak power in relation to age, body size, gender, and thigh muscle volume. Pediatric Exercise Science 15, 406-418.
|
 Sargeant A., Dolan P., Thorne A., Binkhorst R, Kemper H, Saris W. (1985) Children and Exercise XI. Effects of supplementary physical activity on body composition, aerobic, and anaerobic Power in 13-year-old boys. Champaign. Human Kinetics.
|
 Sharp R., Costill D., Fink W., King D. (1986) Effect of 8 weeks of bicycle ergometer sprint training on human muscle buffer capacity. International Journal of Sports Medicine 7, 13-17.
|
 Staron R., Karapondo D., Kraemer W., Fry A., Gordon S., Falkel J., Hagerman F., Hikida R. (1994) Skeletal Muscle Adaptations During Early Phase of Heavy Resistance Training in Men and Women. Journal of Applied Physiology 76, 1247-1255.
|
 Stratton J.R., Levy W.C., Cerqueira M.D., Schwartz R.S., Abrass I.B. (1994) Cardiovascular responses to exercise. Effects of aging and exercise training in healthy men. Circulation 89, 1648-1655.
|
 Tolfrey K., Armstrong N. (2007) Paediatric exercise science: Advances in sport and exercise ecience series. Responses to training. Edinburgh. Churchill Livingstone.
|
 Urhausen A., Kindermann W. (2002) Diagnosis of overtraining: What tools do we have?. Sports Medicine 32, 95-102.
|
 Uusitalo A. (2006) A comment on: Prevention, diagnosis and treatment of the overtraining syndrome. European Journal of Sports Science 6, 261-262.
|
 Verma S., Makindroo S., Kansal D. (1992) Decreased nocturnal catecholamine excretion: Parameter for an overtraining syndrome in athletes?. International Journal of Sports Medicine 13, 236-242.
|
 Vrigens D. (1978) Muscle strength development in the pre-pubescent and post-pubescent age. Medicine and Science in Sports 11, 152-158.
|
 Welsman J.R., Armstrong N., Withers S. (1997) Responses of young girls to two modes of aerobic training. British Journal of Sports Medicine 31, 139-142.
|
 Wilmore J.H., Stanforth P.R., Gagnon J., Rice T., Mandel S., Leon A.S., Rao D.C., Skinner J.S., Bouchard C. (2001) Cardiac output and stroke volume changes with endurance training: The HERITAGE family study. Medicine and Science in Sports and Exercise 33, 99-106.
|
 Wolfe L.A., Cunningham D.A., Boughner D.R. (1986) Physical conditioning effects on cardiac dimensions: A review of echocardiographic studies. Canadian Journal of Applied Sports Science 11, 66-79.
|
 Yoshida T., Ishiko I., Muraoka I. (1980) Effect of endurance training on cardiorespiratory functions of 5-year-old children. International Journal of Sports Medicine 1, 91-94.
|
 Yudofsky S., Hales R. (1992) The American psychiatric press textbook of neuropsychiatry. Washington. London American Psychiatric Press.
|
|
|
|
|
|
|