|
|
|
ABSTRACT |
The purpose of the study is to examine the effect of aerobic exercise intensity on components of the differentiated perceived exertion model in young women performing weight bearing and non-weight bearing aerobic exercise. Subjects were 18-25 yr old women who were recreationally active (n = 19; VO2max = 33.40 ml·kg-1·min-1) and trained (N = 22; VO2max = 43.3 ml·kg-1·min-1). Subjects underwent two graded exercise tests (GXT) on a treadmill and bike which were separated by 48 hours. RPE-Overall, -Legs, and -Chest, as well as oxygen uptake (VO2) and heart rate were recorded each minute. Individual regression analyses were used to identify RPE-Overall,-Legs, and -Chest at 40, 60, 80% VO2max/peak. Separate two factor (site (3) x intensity (3)) ANOVAs with repeated measures on site and intensity were computed for each training status. Furthermore, RPE responses were also examined with a one factor (site (3)) within subject ANOVA with repeated measure on site at the ventilatory breakpoint. For both the recreationally active and trained groups no significant differences were observed for RPE-Overall, -Legs, and -Chest during treadmill exercise. However, for cycling exercise results indicated that RPE-Legs was significantly greater at all exercise intensities than RPE-Overall and RPE-Chest for trained subjects while for recreationally active subjects RPE-Legs was only significantly higher at the highest exercise intensity. Responses at the ventilatory breakpoint during cycle exercise indicated that RPE-Legs was significantly greater than RPE-Chest and RPE-Overall for trained subjects but not for recreationally active subjects. Signal dominance was not observed at an intensity equivalent to the ventilatory breakpoint during treadmill exercise in either of the groups. In recreationally active and trained females signal dominance was demonstrated only during cycling exercise, but not during treadmill exercise. Signal integration could not be demonstrated during cycling and treadmill exercise at various intensities. |
Key words:
Aerobic exercise, physical exertion, oxygen consumption, physical fitness
|
Key
Points
- RPE is a valid tool to track relative exercise intensity and can be applied as differentiated and undifferentiated responses regardless of training status.
- RPE-Legs dominated the signal response in trained women during cycling exercise.
- RPE-Legs, -Chest, and -Overall did not differ significantly in trained and recreationally active women during treadmill exercise.
- RPE-Legs and -Chest contribute equally to the formation of RPE-Overall during cycling and treadmill exercise.
|
The perception of physical exertion is defined as the subjective intensity of effort, strain, discomfort and/or fatigue that one feels during exercise (Robertson and Noble, 1997). Ratings of perceived exertion (RPE) can be used to guide the time course of exercise testing (ACSM, 2000; 2006), and also to prescribe exercise intensity for training sessions (Glass et al. , 1992). RPE can be measured as overall body feelings of exertion (RPE-Overall), or differentiated feelings reflecting respiratory and metabolic functions arising from the chest (RPE-Chest) and alterations in energy producing and contractile properties of peripheral and skeletal muscle (RPE-Legs/RPE-arms). The Differentiated RPE Model holds that discrete perceptual signals are linked to specific underlying physiological events (Robertson and Noble, 1997; Weiser and Stamper, 1977). These perceptual signals are often anatomically regionalized to the active limbs, as well as to the chest. Differentiated peripheral signals are mediated by changes in skeletal muscle environment. Movement of muscles and joints provide a localized peripheral perceptual signal that can be measured as differentiated ratings for the legs (RPE-Legs) or arms (RPE-Arms). As developed tension in the active muscle groups increases so does the intensity of the peripheral signal (Allen and Pandolf, 1977). Furthermore, higher exercise intensities increase anaerobic energy demands and hence the production of tissue lactate, which has been linked to the intensity of peripheral signals of muscular effort (Allen and Pandolf, 1977). On the other hand, a rating for the chest/breathing (RPE-Chest) is a measure of respiratory/metabolic exertional signals. These signals are linked to increased aerobic energy demands that occur with progressively greater exercise intensities. The strength of the respiratory- metabolic signal is associated with exercise induced increases in oxygen uptake, pulmonary ventilation, and respiratory rate with somewhat less input from chronotropic heart rate response (Borg and Dahlstrom, 1962; Cafarelli, 1977; Edwards et al., 1972; Kamon et al., 1974). One dimension of the Differentiated RPE Model considers whether the intensity of one type of perceptual signal (i.e. peripheral or respiratory/metabolic) is relatively more intense than the other. When such a difference is detected it is termed perceptual signal dominance. The undifferentiated perceptual signal represents an integration of many differentiated perceptual signals and thus forms a rating for the overall body (Pandolf et al., 1975). This concept is termed perceptual signal integration. In addition, each differentiated signal is assumed to carry a specific intensity weighting with the most intense signal dominating the sensory integration process that forms the overall body RPE (Robertson and Noble, 1997). This concept is termed perceptual signal dominance. The model of signal integration predicts that RPE-Overall is a weighted average of RPE-Legs (or RPE-Arms) and RPE-Chest. This integration likely takes place in the sensory cortex (Cafarelli, 1982; Robertson and Noble, 1997). Perceptual signal dominance has been demonstrated in adults performing cycle ergometer (non-weight-bearing) and treadmill (weight-bearing) exercise (Pandolf et al., 1975; Robertson et al., 1979a; 2004). These studies found that the differentiated RPE for the legs was consistently more intense than the respiratory/metabolic signals arising from the chest. When examining these studies collectively, the findings for adult samples generally indicate that perceptual signal dominance is present at comparatively lower exercise intensities during non-weight-bearing exercise modes but may be delayed in onset during weight-bearing exercise modes. The differentiation threshold, or the exercise intensity at which one of the differentiated signals becomes more intense than the other, appears to occur at a lower aerobic metabolic rate during cycle ergometry than treadmill exercise. During cycle ergometry, the differentiated signal from the legs is typically more intense than that from either the chest or the overall signal, with the threshold becoming apparent at comparatively low exercise intensities (Pandolf et al., 1975; Robertson et al., 1979a; Robertson et al., 2004). However, for weight-bearing exercise, such as walking and running, the threshold at which the differentiated leg signal becomes dominant occurs at higher intensities, a response that seems to be a function of body weight and walking/running speed (Robertson et al., 1982). As an extension to these previous findings the present study examined whether training status, exercise mode, and exercise intensity plays a role in the signal dominance/integration process. The study's rationale was that determining the influence of exercise intensity on the differentiated perceptions of exertion for recreationally active and trained subjects during non-weight bearing (cycle) and weight bearing (treadmill) exercise may increase the precision of exercise prescriptions. Also, there are still many elements of the differentiated perceptual rating that are not well understood, especially with respect to the two component differentiated signal model, i.e. perceptual signal dominance and mode of signal integration. Specifically, the effect of aerobic exercise intensities (low, moderate, high) in people of various physical fitness levels (recreationally active vs. trained) during different exercise modes (cycle vs. treadmill) has not been extensively examined as interactive variables within the context of the Differentiated RPE Model. Hence, the purpose of this study was to examine the effect of exercise intensity on differentiated RPE for the legs and chest during treadmill and cycling exercise in recreationally active and trained females. It was hypothesized that recreationally active females would have a higher RPE-Chest than RPE-Legs and RPE-Overall during cycling and treadmill exercise. On the other hand, it was hypothesized that trained females would have higher RPE-Legs than RPE-Chest and RPE-Overall.
SubjectsSubjects for this investigation were aerobically trained (n = 22) and recreationally active (n = 19) 18 - 25 year-old females. Inclusion criteria for this study were based on gender, training status and aerobic fitness. Subjects for this investigation were college age females (18-25 years old), who were recruited from physical activity classes, and/or who exercised at the university's fitness facilities. Participants were healthy, of normal body weight and were classified as either recreationally active or trained. Recreationally active was defined as participating less than or equal to twice a week in aerobic activity for a total of 80 minutes at moderate intensity (~5-6 METS) and having a maximal oxygen uptake (VO2max) that is classified at or below the average for age (≤ 35.2 ml·kg-1·min-1) (ACSM, 2006). Trained was defined as participating in aerobic activities at least five times a week for a minimum of 200 minutes at moderate (~5-6 METS) to high (~10-11 METS) intensity and having a VO2max above the superior classification for age (≥ 44.2 ml·kg-1·min-1) (ACSM, 2006). Participants with a body mass index (BMI) of ≥ 30 kg·m-2 were excluded from the study. Also, participants with known cardiovascular or pulmonary disease were excluded.
Experimental designThis investigation employed a within subject cross-sectional experimental design consisting of one load incremented treadmill exercise trial and one load-incremented cycle ergometer trial. Both load incremented trials were terminated at maximal/peak exercise intensity. The exercise trials were presented in counterbalanced order and separated by a minimum of 48h and maximum of 72h. Prior to the first exercise trial training status was determined by a questionnaire and body weight (kg) and height (cm) were measured. Each exercise trial began with an orientation phase, i.e. 15 minutes. The orientation included: (a) a definition of perceived exertion, (b) OMNI Perceived Exertion Scale anchoring procedures, and (c) OMNI Scale instructions. Each of these orientation procedures used the OMNI Scale pictorial format that was consistent with the mode to be used in the exercise trial for that day. That is, the OMNI Cycle Scale was used in the cycle test orientation and the OMNI Walk/Run Scale was used in the treadmill orientation.
Ratings of Perceived ExertionRatings of perceived exertion were measured during the treadmill protocol using the walk/run format of the Adult OMNI Scale and during the cycle protocol using the cycling format of the Adult OMNI Scale. Perceptual ratings were obtained from 40-60 seconds of each exercise minute during both protocols. An undifferentiated rating for the overall body (RPE-Overall), and differentiated ratings for peripheral perceptions of exertion in the legs (RPE-Legs) and respiratory-metabolic perceptions for the chest/breathing (RPE-Chest) were obtained. A standard definition of perceived exertion and an instructional set for the mode specific OMNI Scale were read to subjects immediately before each exercise protocol. Either the walk/run or cycle format of the OMNI Scale was viewed by subjects when their respective instructional set was read. Both scales were anchored using a combination exercise and memory procedures. Participants were counterbalanced and asked at the end of each minute (40-60 seconds) of the exercise protocol to give three ratings of perceived exertion by pointing to the appropriate numbers on the scale, i.e. a rating for their overall body, for their legs, and for their chest and breathing. A pointing procedure was used as the respiratory valve/mouthpiece prevented a verbal response. The OMNI Scale was placed in full view of the subjects during the entire exercise trial.
Exercise trialsAnthropometric measures Body mass (kg) and height (cm) were determined at the beginning of the first trial using a Detecto-Medic Scale and attached stadiometer (Detecto Scales Inc., Brooklyn, NY) and were also used to calculate BMI. Percent body fat was determined by bioelectrical impedance analysis (BIA) (TBF-300A, Tanita, Arlington Heights, IL) using the standard mode. These data were used to describe the subject cohort. Cardiorespiratory and aerobic metabolic measures Oxygen uptake (VO2, L·min-1 and ml·kg-1·min- 1), carbon dioxide production (VCO2 L·min-1, ml·kg-1·min-1) and pulmonary ventilation (VE L·min-1) during each trial were measured using an open- circuit respiratory metabolic system (TrueMax 2400, Parvo Medics, Salt Lake City, UT). Measures were determined every 15 seconds and expressed as STPD. System calibration was undertaken before each trial. A standard respiratory valve (Model 2700; Hans Rudolph, Kansas City, MO) and mouthpiece were used for all respiratory-metabolic measurements. Heart rate (HR) was measured using a Polar Monitor System (Polar Electro Inc., Woodbury, NY). Maximal/ oxygen uptake was taken as the highest value recorded during the final stage of the exercise protocols when: Any one or combination of the above criteria was accepted as confirmation of attainment of VO2max. The ventilatory breakpoint (Vpt) was determined as published by Mahon et al., 1998 for both the treadmill and cycle ergometer exercise tests using respiratory-gas exchange measurements obtained every 15 seconds.. The Vpt was determined from individual plots for each subject and was expressed in both absolute (L·min-1 and ml·kg-1·min-1) and relative (%VO2max) units.
Testing protocolsAn orientation to the OMNI RPE Scales and testing procedures was presented prior to the exercise protocols. Immediately prior to the exercise tests the investigator read a standard set of scaling instructions to the subject. These included the definition of perceived exertion, scale anchors and procedures to use the OMNI Cycle Scale/OMNI Walk/Run Scale respectively. The cycle ergometer exercise test was administered using a Monark cycle ergometer (Model 864, Sweden) equipped with toe clips and a plate- loading system to apply break force. Seat height was determined before the start of the trial by adjusting the seat so that the subject had 5° knee flexion when sitting on the cycle with the foot in its lowest position. The load-incremented cycle protocol consisted of 3 minute exercise stages, with an initial resistance of 25 Watts. Resistance was incremented 25 Watts every 3 minutes. Subjects were instructed to maintain a constant cadence of 50 rev/min signaled by an electronic metronome. The resistance was set by the investigator at the beginning of each stage, the absolute value not known to the subject. The test was terminated when the subject volitionally stopped owing to fatigue, or the investigator determined that the subject could not maintain the designated pedal rate for 10 consecutive seconds. The treadmill exercise test was performed on a Trackmaster treadmill, model TMX 425C (FullVision Inc, Newton, KS). A modified Bruce protocol, with 3 minute exercise stages was employed. The treadmill speed/grade control system were preprogrammed for the modified Bruce protocol, and interfaced with the respiratory-metabolic unit for automatic stage-by-stage adjustments. For both exercise tests measurement order for RPE-Overall, RPE- Legs, and RPE-Chest was established by a counterbalance sequence. A restricted randomized procedure was used to assign subjects to the counterbalance sequence. For a given subject the same measurement order was used for each exercise minute. Subjects followed a different counterbalance sequence than was employed in the alternate exercise mode. RPE-Overall, RPE-Legs, and RPE-Chest were measured during the last 20 seconds of each exercise minute. VO2, VCO2, and VE were measured in 15 second intervals during each minute. HR was measured during the last 15 seconds of each exercise minute. The %VO2max was calculated for each minute using the average VO2 for the last 30 seconds of each minute. The highest measured HR was accepted as the maximal HR. Verbal encouragement (good job, keep it up) was given each minute throughout the trial by the investigator.
Data analysisSample size was determined by power analysis. Descriptive data for perceptual and physiological variables were calculated as mean ± standard deviation. A regression analysis was used to identify RPE-Overall, -Legs, and -Chest equivalent to 40, 60, and 80% VO2max/peak. Separate regression analyses were calculated for individual subjects. For the first research purpose separate calculations were performed for cycling and treadmill exercise. The derived RPE data (sites:-Overall, -Legs, -Chest) were examined with a two factor (site (3) x intensity (3)) ANOVA with repeated measures on site and intensity. Separate ANOVAs were calculated for each training status. Level of significance was set at p < 0. 05. Significant interactions were plotted and any significant main effects were examined with a Bonferroni post-hoc analysis. For the second research purpose, separate calculations were performed for cycling and treadmill exercise. RPE (-Overall, -Legs, -Chest) measured at the ventilatory breakpoint were examined with a one factor (site (3)) within subject ANOVA with repeated measure on site. Separate analyses were conducted for each exercise mode. Level of significance was set at p < 0.05. Significant main effects were examined with a Bonferroni post-hoc analysis.
There was no significant difference between groups regarding age, height, weight, BMI and percent body fat. VO2max were lower (p < 0.05) for the recreationally active as compared to the trained group.
RPE responses
Cycle exerciseThe mean (± SD) for RPE responses of the recreationally active and trained subjects are presented in Table 1. The interaction effect for site x intensity was significant (F(1.5, 27.8) = 10.56, p = 0. 001). Simple effects post-hoc analysis indicated that for recreationally active subjects, RPE-Legs was significantly higher than RPE-Overall and RPE-Chest at 80% of VO2max, while RPE- Overall and RPE-Chest were similar to each other (Table 1). There was no difference between RPE-Overall, -Legs, and -Chest at 40 and 60% of VO2max during cycling exercise. There was also a main effect of intensity (F(1.23, 22.13) = 522, p < 0. 01) on RPE for recreationally active subjects during cylcing. Because the interaction was ordinal the main effect for intensity can also be interpreted. The main effect for intensity indicated that when averaged over site, RPE increased from 40% (RPE = 1.67) to 60% (RPE = 4.57) to 80% (RPE = 7. 61) VO2max, meaning that subjects reported higher RPEs as intensity increased. For trained subjects performing cycle exercise the interaction effect for site x intensity was significant (F(2.19, 43.83) = 11.65, p < 0.01). Simple effects post-hoc analysis indicated that for trained subjects, RPE-Legs was significantly higher than RPE-Overall and RPE-Chest, while RPE- Overall and -Chest were similar to each other at all three intensities (Table 1). There were also significant main effects of site and intensity on RPE (F(1.50, 30.15) = 1009, p < 0.01) for trained subjects. Post-hoc comparisons for intensity indicated that when averaged over site, RPE increased from 40% (RPE = 1.61) to 60% (RPE = 4.44) to 80% (RPE = 7.46) VO2max. The main effect for site indicated that RPE-Legs was significantly higher than RPE-Overall and RPE-Chest for all intensities in trained subjects during cycling exercise.
Treadmill exerciseNo site x intensity interaction effect on RPE was found for recreationally active subjects during treadmill exercise. The main effect for measurement site was not significant while the main effect for intensity was significant (F(1.10, 19.87) = 280, p < 0.01). Post-hoc comparisons for intensity indicated that when averaged over site (i.e. Overall, Legs, Chest), RPE increased from 40% (RPE = 1.58) to 60% (RPE = 3.92) to 80% (RPE = 6.57) VO2max. No site x intensity interaction effect on RPE was found for trained subjects during treadmill exercise. The main effect for measurement site was not significant while the main effect for intensity was significant (F(1.30, 27.39) = 440, p < 0.01). Post-hoc comparisons for intensity indicated that when averaged over site (i.e. Overall, Legs, Chest), RPE increased from 40% (RPE = 1.21) to 60% (RPE = 3.85) to 80% (RPE = 6.74) VO2max.
Ventilatory breakpointThe main effect of site for RPE measured at the ventilatory breakpoint was not significant for the recreationally active subjects during cycling exercise. This finding indicates that at a cycle intensity equivalent to the ventilatory breakpoint, recreationally active subjects did not report a dominant RPE. However, a significant main effect for site was reported for the trained subjects (F(1.57, 31.35) = 12.13, p < 0.01). Post-hoc comparisons indicated that at the ventilatory breakpoint trained subjects reported significantly higher RPE-Legs than RPE-Overall and RPE-Chest during cycle exercise. This finding is also depicted in Table 2. There was no main effect of site on RPE at the ventilatory breakpoint for either activity group during treadmill exercise. These results indicate that neither recreationally active nor trained subjects experienced a dominant signal at the ventilatory breakpoint during treadmill exercise (Table 2).
Signal dominanceThe findings of this investigation indicated that during cycle exercise a dominant perceptual signal was evident for recreationally active women at a high relative exercise intensity (i.e. 80% VO2max), while trained women experienced a dominant perceptual signal at low through high relative exercise intensities (40 to 80% VO2 peak). RPE-Legs was significantly higher than RPE-Overall and -Chest for trained subjects at all cycle exercise intensities. RPE-Legs appeared as the dominant signal for recreationally active subjects only during exercise at the 80%VO2max intensity. Previous studies concluded that the peripheral differentiated signal likely dominates the whole body sensory integration process during aerobic exercise on a cycle ergometer. In contrast, perceptual signal dominance could not be established during treadmill exercise for recreationally active and trained women. At any exercise intensity, RPE-Overall, -Legs and -Chest did not differ significantly. Furthermore, it was not possible to determine if RPE-Overall appeared to be an average of the differentiated signals arising from the legs and chest. For treadmill exercise at low, moderate, and high intensity the present results showed no difference between differentiated and undifferentiated RPE for recreationally active as well as trained subjects. This finding is in agreement with results previously reported by Seip and colleagues (Seip et al., 1991) as well as Rutkowski and colleagues (Rutkowski et al., 2004). On the other hand, they are somewhat in contrast to findings observed by Robertson and colleagues (1982) who demonstrated that in adults the onset of a dominant perceptual signal is keyed to a specific locomotor speed. However, even in Robertson's et al. (1982) study, subjects did not evidence a dominant perceptual signal at speeds less than 4 min?h-1. Since none of the subjects reached walking speeds above 4 min?h-1 the present findings are at least partially in agreement with Robertson and colleagues' study. Similar responses to those noted above were found for perceptual signal dominance at the ventilatory breakpoint during cycle ergometer and treadmill exercise. Neither respiratory- metabolic nor peripheral ratings of perceived exertion appeared to dominate the overall body sensory integration process at exercise intensities equivalent to the ventilatory breakpoint for recreationally active and trained subjects during treadmill exercise. Only the trained subjects experienced dominant perceptual signals from the legs during cycle ergometry at an intensity equivalent to the ventilatory breakpoint.
Mode of signal integrationDuring cycle exercise it was not possible to determine if RPE-Overall appeared as an average of the differentiated signals arising from the legs and chest. While the difference in RPE was statistically significant at all cycle exercise intensities for trained subjects and at the high exercise intensity for the recreationally active subjects, the absolute value of the difference was very small. As such, it appears that RPE-Legs and -Chest contributed equally to the formation of RPE-Overall during cycling exercise in recreationally active and trained women. However, for treadmill exercise the differentiated ratings did not differ from the undifferentiated rating for recreationally active and trained subjects at any of the exercise intensities and it appeared that RPE-Legs and -Chest contributed equally to the formation of RPE-Overall. Similar responses to those noted above were found for mode of integration at the ventilatory breakpoint during cycle ergometer and treadmill exercise. Only the trained subjects experienced dominant perceptual signals from the legs during cycle ergometry at an intensity equivalent to the ventilatory breakpoint. This response provided evidence for perceptual signal integration at an intensity equivalent to the ventilatory breakpoint. Since a difference between the differentiated and undifferentiated ratings was not observed, RPE-Legs and -Chest contributed equally to the formation of RPE-Overall. The literature shows equivocal findings regarding perceptual signal dominance and integration at the ventilatory breakpoint and thus more research in this area is needed (Demello et al., 1987; Robertson et al., 2000). The findings of the present study for cycling exercise, support previous reports (Robertson et al., 1982; 2000; 2001) that examined the two components of the differentiated perceived exertion model (e.g. signal dominance and mode of integration). However, since in the present study both differentiated signals were similar they may have had equal input into the sensory integration process during cycle exercise. For treadmill exercise at low, moderate, and high intensity the present results showed no difference between differentiated and undifferentiated RPE for recreationally active as well as trained subjects. Because the differentiated RPE for the legs and chest did not differ it appeared that RPE-Legs and -Chest contributed equally to the formation of RPE-Overall and thus the whole-body sensory integration process.
Weight-bearing and non-weight-bearing exerciseNon-weight-bearing and weight-bearing exercise modes were examined as separate elements of the experimental paradigm. This was done to explore the possibility that the differentiated perceived exertion model would be expressed differently depending on the weight bearing properties of the exercise testing mode. It was anticipated that the different modes would require different skeletal muscle recruitment patterns, producing mode specific dominant RPE signals. It can be concluded from the present findings that the weight-bearing properties of exercise mode did not have an effect on signal dominance and integration at relative exercise intensities ranging from 40 to 80% VO2max in recreationally active and trained individuals. Although, it was thought that non-weight-bearing and weight-bearing exercise would elicit muscle specific physiological events leading to differences between the differentiated RPEs this only seemed to be the case for cycling exercise in this study. Thus, the expectation that perceptual signal dominance would be present at comparatively lower exercise intensities during non-weight- bearing exercise, such as cycling, and have a delayed onset during weight-bearing exercise (Pandolf et al., 1975; Robertson et al., 1979b; Robertson et al., 2004) was only partially supported. In light of current findings, future investigations regarding perceptual signal dominance and integration should examine the potential impact of gender difference, aging, a range of running speeds, weight-bearing vs. non-weight bearing exercise modes, and constant load vs. continuous and intermittent exercise protocols.
Overall, these results demonstrate that RPE is a valid tool to track relative exercise intensity and that it can be applied as differentiated or undifferentiated responses regardless of training status. Furthermore, it appears that both RPE-Chest and RPE-Legs mediate RPE- Overall equally during cycling and treadmill exercise.
|
AUTHOR BIOGRAPHY |
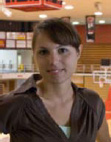 |
Melinda R. Bolgar |
Employment: Assistant Professor in the department of Health, Physical Education and Recreation at Jacksonville State University. |
Degree: PhD |
Research interests: |
E-mail: mbolgar@jsu.edu |
|
 |
Carol E. Baker |
Employment: The former Director of the Office of Measurement and Evaluation of Teaching at the University of Pittsburgh. |
Degree: PhD |
Research interests: |
E-mail: goss@pitt.edu |
|
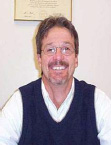 |
Fredric. L. Goss |
Employment: Associate Professor in the Department of Health and Physical Activity and is also a Co-Director of the Center for Exercise and Health-Fitness Research and the University Wellness Center at the University of Pittsburgh. |
Degree: PhD |
Research interests: Scaling exertional perceptions, adult fitness and wellness, combined arm and leg exercise. |
E-mail: goss@pitt.edu |
|
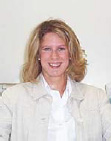 |
Elizabeth Nagle |
Employment: Assistant Professor in the Department of Health and Physical Activity at the University of Pittsburgh. |
Degree: PhD |
Research interests: Physiologic responses to aquatic exercise, body composition, and cardiovascular, metabolic, and hemodynamic responses to exercise |
E-mail: nagle@pitt.edu |
|
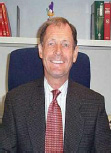 |
Robert J. Robertson |
Employment: Professor in the Department of Health and Physical Activity and is also a Co-Director of the Center for Exercise and Health-Fitness Research at the University of Pittsburgh. |
Degree: PhD |
Research interests: Scaling exertional perceptions and directed the investigative team that developed and validated the OMNI pictorial scale. |
E-mail: rrobert@pitt.edu |
|
|
|
REFERENCES |
 Allen P.D., Pandolf K.B. (1977) Perceived exertion associated with breathing hyperoxic mixtures during submaximal work. Medicine and Science in Sports and Exercise 99, 122-127.
|
 American College of Sports Medicine (2000) Guidelines for exercise testing and prescription. Philadelphia, PA. Lippincott Williams and Wilkins.
|
 American College of Sports Medicine (2006) Guidelines for exercise testing and prescription. Philadelphia, PA. Lippincott Williams & Wilkins.
|
 Borg G., Dahlstrom H. (1962) The reliability and validity of a physical work test. Acta Physiologica Scandinavica 555, 353-361.
|
 Cafarelli E. (1977) Peripheral and central inputs to the effort sense during cycling exercise. European Journal of Applied Physiology and Occupational Physiolog 337, 181-189.
|
 Cafarelli E. (1982) Peripheral contributions to the perception of effort. Medicine and Science in Sports and Exercise 114, 382-389.
|
 Demello J.J., Cureton K.J., Boineau R.E., Singh M.M. (1987) Ratings of perceived exertion at the lactate threshold in trained and untrained men and women. Medicine and Science in Sports and Exercise 119, 354-362.
|
 Edwards R.H., Melcher A., Hesser C.M., Wigertz O., Ekelund L.G. (1972) Physiological correlates of perceived exertion in continuous and intermittent exercise with the same average power output. European Journal of Clinical Investigation 22, 108-114.
|
 Glass S.C., Knowlton R.G., Becque M.D. (1992) Accuracy of RPE from graded exercise to establish exercise training intensity. Medicine and Science in Sports Exercise 224, 1303-1307.
|
 Kamon E., Pandolf K., Cafarelli E. (1974) The relationship between perceptual information and physiological responses to exercise in the heat. Journal of Human Ergology (Tokyo) 33, 45-54.
|
 Mahon A.D., Gay J.A., Stolen K.Q. (1998) Differentiated ratings of perceived exertion at ventilatory threshold in children and adults. European Journal of Applied Physiology and Occupational Physiology 778, 115-120.
|
 Pandolf K.B., Burse R.L., Goldman R.F. (1975) Differentiated ratings of perceived exertion during physical conditioning of older individuals using leg-weight loading. Perceptual Motor Skills 440, 563-574.
|
 Robertson R.J., Caspersen C.J., Allison T.G., Skrinar G.S., Abbott R.A., Metz K.F. (1982) Differentiated perceptions of exertion and energy cost of young women while carrying loads. European Journal of Applied Physiology and Occupational Physiology 449, 69-78.
|
 Robertson R.J., Gillespie R.L., Mc Carthy J., Rose K.D. (1979a) Differentiated perceptions of exertion: part I. mode of integration of regional signals. Perceptual Motor Skills 449, 683-689.
|
 Robertson R.J., Gillespie R.L., Mc Carthy J., Rose K.D. (1979b) Differentiated perceptions of exertion: part II relationship to local and central physiological responses. Perceptual Motor Skills 449, 691-697.
|
 Robertson R.J., Goss F.L., Boer N., Gallagher J.D., Thompkins T., Bufalino K., Balasekaran G., Meckes C., Pintar J., Williams A. (2001) OMNI scale perceived exertion at ventilatory breakpoint in children: response normalized. Medicine and Science in Sports and Exercise 333, 1946-1952.
|
 Robertson R.J., Goss F.L., Dube J., Rutkowski J., Dupain M., Brennan C., Andreacci J. (2004) Validation of the adult OMNI scale of perceived exertion for cycle ergometer exercise. Medicine and Science in Sports and Exercise 336, 102-108.
|
 Robertson R.J., Moyna N.M., Sward K.L., Millich N.B., Goss F.L., Thompson P.D. (2000) Gender comparison of RPE at absolute and relative physiological criteria. Medicine and Science in Sports and Exercise 332, 2120-2129.
|
 Robertson R.J., Noble B.J. (1997) Perception of physical exertion: methods, mediators, and applications. Exercise and Sport Science Review 225, 407-452.
|
 Rutkowski J.J., Robertson R.J., Tseh W.D., Caputo J.L., Keefer D.J., Sutika K.M., Morgan D.W. (2004) Assessment of RPE signal dominance at slow-to-moderate walking speeds in children using the OMNI Perceived Exertion Scale. Pediatric Exercise Science 116, 334-342.
|
 Seip R. L., Snead D., Pierce E. F., Stein P., Weltman A. (1991) Perceptual responses and blood lactate concentration: effect of training state. Medicine and Science in Sports and Exercise 223, 80-87.
|
 Weiser P.C., Stamper D.A., Borg G. (1977) Physical work and effort. Psychophysiological interactions leading to increased effort, leg fatigue, and respiratory distress during prolonged, strenuous bicycle riding. New York. Pergamon Press.
|
|
|
|
|
|
|