|
|
|
ABSTRACT |
Abdominal bracing is often adopted in fitness and sports conditioning programs. However, there is little information on how muscular activities during the task differ among the muscle groups located in the trunk and from those during other trunk exercises. The present study aimed to quantify muscular activity levels during abdominal bracing with respect to muscle- and exercise-related differences. Ten healthy young adult men performed five static (abdominal bracing, abdominal hollowing, prone, side, and supine plank) and five dynamic (V- sits, curl-ups, sit-ups, and back extensions on the floor and on a bench) exercises. Surface electromyogram (EMG) activities of the rectus abdominis (RA), external oblique (EO), internal oblique (IO), and erector spinae (ES) muscles were recorded in each of the exercises. The EMG data were normalized to those obtained during maximal voluntary contraction of each muscle (% EMGmax). The % EMGmax value during abdominal bracing was significantly higher in IO (60%) than in the other muscles (RA: 18%, EO: 27%, ES: 19%). The % EMGmax values for RA, EO, and ES were significantly lower in the abdominal bracing than in some of the other exercises such as V-sits and sit-ups for RA and EO and back extensions for ES muscle. However, the % EMGmax value for IO during the abdominal bracing was significantly higher than those in most of the other exercises including dynamic ones such as curl-ups and sit-ups. These results suggest that abdominal bracing is one of the most effective techniques for inducing a higher activation in deep abdominal muscles, such as IO muscle, even compared to dynamic exercises involving trunk flexion/extension movements. |
Key words:
Static and dynamic exercises, electromyogram, voluntary co-contraction, muscle- and exercise-related differences
|
Key
Points
- Trunk muscle activities during abdominal bracing was examined with regard to muscle- and exercise-related differences.
- Abdominal bracing preferentially activates internal oblique muscles even compared to dynamic exercises involving trunk flexion/extension movements.
- Abdominal bracing should be included in exercise programs when the goal is to improve spine stability.
|
Trunk stability training for enhanced health, rehabilitation, and athletic performance has received renewed emphasis (Behm et al., 2010). In the past, these types of exercises were performed only by individuals with low back problems in physical therapy clinics (McGill, 2001). In recent years, however, fitness professionals have increasingly emphasized trunk stability exercises in sports conditioning programs because it is considered that greater trunk stability may benefit sports performance by providing a foundation for greater force production in the upper and lower extremities (Willardson, 2007). Trunk muscles function in transferring torques and angular momentum during the performance of integrated kinetic chain activities, such as throwing or kicking (Kibler et al., 2006; Willardson, 2007). Weakness in the trunk musculature may interrupt the transfer of torques and angular momentum, resulting in decreased performance (Behm et al., 2010). Kibler et al., 2006 summarized trunk stability in a sporting environment as “the ability to control the position and motion of the trunk over the pelvis to allow optimum production, transfer, and control of force and motion to the terminal segment in integrated athletic activities”. Thus, specific training practices aimed at targeting the trunk stabilizing muscles are an important consideration not only for activities of daily living or rehabilitation of low back pain, but also for athletic performance (Behm et al., 2010). Monfort-Panego et al., 2009 suggested that abdominal co-contraction (bracing) is one of the most effective exercise techniques for trunk stabilization training. In fact, abdominal bracing has been shown to increase the stiffness of the spine, promoting stability in the vertebral segments (Vera-Garcia et al., 2006; 2007), and is often recommended and/or included in rehabilitation and/or fitness programs (Marshall et al., 2011; Monfort-Panego et al., 2009). Some studies (Allison et al., 1998; Bressel et al., 2011; 2012; Vera-Garcia et al., 2010) have measured the electromyogram (EMG) activities of trunk muscles during abdominal bracing exercise together with some other trunk exercises. Bressel et al., 2012, who examined EMG activities during various trunk exercises (e.g., abdominal hollowing, anteroposterior/mediolateral pelvic tilts, and swiss ball exercises) performed underwater, reported that abdominal bracing was one of the most effective exercises to maximize the global trunk muscle activities. In addition, Vera-Garcia et al., 2007 compared the effects of abdominal bracing and abdominal hollowing maneuvers on the control of spine motion and stability against sudden trunk perturbations in healthy males. They found that abdominal bracing was more effective than abdominal hollowing for stabilizing the spine against posterior and rapid loading. The findings cited above support the assertion that abdominal bracing is an effective technique for improving spine stability. What seems to be lacking, however, is substantial information on how the activity levels of the muscle groups located in the trunk differ during abdominal bracing and other trunk exercises such as prone plank, curl-ups, or back extensions. As cited above, EMG activities during abdominal bracing have been already reported for some of the trunk muscles, such as the rectus abdominis, external oblique, internal oblique, transversus abdominis, and erector spinae muscles (Allison et al., 1998; Bressel et al., 2011; Urquhart et al., 2005; Vera-Garcia et al., 2010). However, muscle-related differences in the activation level during abdominal bracing have not been thoroughly discussed. For example, some studies reported muscle-related differences in EMG activities during abdominal bracing (Allison et al., 1998; Urquhart et al., 2005). In the prior studies, however, the EMG activities were not normalized to the maximum value (Allison et al., 1998), or the task was performed with mild effort (Urquhart et al., 2005). Vera-Garcia et al., 2010 and Bressel et al., 2011 expressed each of the EMG activities of the trunk muscles during abdominal bracing as the value relative to that during the maximal voluntary contraction (MVC) task for the corresponding muscle. However, they focused on the difference in the activation level of each muscle between the abdominal bracing and other MVC tasks (Vera-Garcia et al., 2010), or between terrestrial and underwater conditions (Bressel et al., 2011). Thus, muscle-related differences in muscular activities during abdominal bracing are not well established. Furthermore, the exercises measured with and compared to abdominal bracing in the previous study (Bressel et al., 2012) were therapeutic aquatic exercises designed for patients with lower back pain. Trunk exercises, which are conducted in not only rehabilitative but also athletic situations, usually include much more dynamic exercises, such as V-sits and curl-ups. To clarify the efficacy of abdominal bracing as a training modality for improving the function of trunk muscles, the magnitude of muscular activities during abdominal bracing should be examined through comparison with those during other trunk exercises including dynamic tasks. In this study, we aimed to clarify the characteristics of trunk muscle activities during abdominal bracing with regard to muscle- and exercise-related differences. For comparison of exercises, 5 static exercises, which are often prescribed in rehabilitation programs, and 5 dynamic exercises, which are usually conducted for strength-training purposes, were chosen. The results of this study may be useful information for clinicians and trainers who prescribe trunk exercises including abdominal bracing.
SubjectsThis study was approved by the Ethics Committee of the National Institute of Fitness and Sports in Kanoya and was consistent with institutional ethical requirements for human experimentation in accordance with the Declaration of Helsinki. Prior to the measurement session, candidates who volunteered to participate in this study visited the laboratory and were fully informed about the procedures and possible risks involved as well as the purpose of the study. After their written informed consent was obtained, they performed all types of task involved in the study to ensure that they had no difficulties or discomfort in any procedures, as well as to familiarize themselves with the procedures. The subjects who could not perform any of the tasks properly were excluded from the study. Ten healthy young adult men participated in this study. The means and standard deviations (SDs) of their age, body height, and body mass were 21.2 ± 1.5 yr, 1. 70 ± 0.05 m, and 65.6 ± 4.8 kg, respectively. All subjects were college students majoring in physical education and were habitually active, but none had been currently involved in any type of regular exercise program (≥ 30 min·day1, ≥ 2 days·week1). In addition, none had experienced musculoskeletal injury or pain in the previous 12 months. Within 72 h of the familiarization session mentioned above, the subjects attended the measurement session.
ProcedureIn the measurement session, isometric MVCs for each muscle were performed for the purpose of normalization. Force during isometric MVC was measured using a custom-made force- measurement device with tension/compression load cells (LUR-A-SA1; Kyowa, Japan). The force signals obtained via a 16-bit A/D converter (Power Lab 16s; ADInstruments, Australia) were recorded on a personal computer at a sampling frequency of 2,000 Hz. In the MVC tasks, as well as subsequent trunk exercise tasks, the surface EMG activities of the trunk muscles were recorded. The repeatability of force measurements during MVC tests was assessed on 2 separate days in a pilot study with 5 young adult men. There was no significant difference between the MVC force values of the two measurements in each task. The intraclass correlation coefficients (ICC) and coefficient of variations (CV) for MVC force were 0.896 and 8.1% for trunk flexion, 0.841 and 11.2% for trunk lateral flexion, and 0.912 and 7.6% for trunk extension. Prior to maximal test, the subjects were asked to exert submaximal force isometrically at each of the test positions to familiarize themselves with the test procedure. After warming-up and a rest period of 3 min, the subjects were encouraged to exert maximal force (progressively increasing the force taking about 5 s) two times with at least 3 min between trials to exclude the influence of fatigue. Subsequent trials were performed if the difference in the peak forces of the two MVCs was greater than 5%. The trial with the highest peak force was selected for analysis. The positions and tasks for MVC were adopted on the basis of the result of Vera-Garcia et al., 2010, and each of the MVC tasks was performed as follows (Figure 1). Trunk flexion: The subjects lay supine on a stable bench seat with knees flexed, and feet flat on the seat and fixed with a strap. By the use of a custom-made belt linked with a chain, which covered the upper torso and was securely connected to the load cells, the subjects were held tightly in position. The subjects then performed maximal isometric trunk flexion in the sagittal plane. Trunk lateral flexion: The subjects lay on their left side on the seat with the legs extended, the hips and feet fixed on the seat with a strap, and the upper torso connected to the load cells using the belt. The subjects then performed maximal isometric lateral flexion (bending right) in the frontal plane. Trunk extension: The subjects lay prone on the bench with the legs extended, the hips and feet fixed on the seat with a strap, and the upper trunk connected to the load cells using the belt. The subjects then performed maximal isometric trunk extension in the sagittal plane. After the completion of MVC tasks, the subjects performed 5 static (abdominal bracing, abdominal hollowing, prone, lateral, and supine plank) and 5 dynamic (V-sits, curl-ups, sit-ups, and back extensions on the floor and on a bench) exercises. During the exercise tasks, the hip joint angles were measured using an electrogoniometer (SG110; Biometrics, UK) and recorded together with the EMG activities and stored on a personal computer. After the subjects were instructed and familiarized with the tasks, a measurement trial for each task was performed with at least 2-min rest interval between each trial. Subsequent trials in each task were performed until both the subject and the researcher considered that the task performed was successful. Static exercises were maintained for 10 s and dynamic exercises were repeated 10 times (1 s for each of raising and lowering phases), and each exercise was performed as follows. Abdominal bracing: In a standing neutral-spine position with the feet shoulder-width apart, participants were instructed to activate the abdominals maximally without hollowing the lower abdomen. Abdominal hollowing: In the same position as for the abdominal bracing task, participants were instructed to draw the navel maximally in toward the spine. Prone plank: In a prone position on the floor with the elbow angle at 90 deg (180 = full extension) and the forearms placed underneath the chest, pelvis raised off the floor and their body weight distributed on the forearms and toes, the subjects were instructed to maintain a flat position. Lateral plank: While lying on the right side on the floor with the right elbow bent at 90 deg and positioned directly under the shoulder, pelvis raised off the floor and their body weight distributed on the forearms and the right side of the foot, the subjects were instructed to maintain the position. Supine plank: In a supine position on the floor with the elbow angle at 90 deg and the forearms placed underneath the back, pelvis rose off the floor and their body weight distributed on the forearms and heels, participants were instructed to maintain the position. V-sits: In a supine position on the floor with the arms extended over the head and the legs extended, subjects were instructed to lift the legs up to a 45 deg angle and extend the arms up toward the ankle. Curl-ups: In a supine position on the floor with the hands behind the head, the knees flexed at 90 deg, and the feet flat on the floor, the subjects were instructed to curl the upper torso up to a 45 deg angle toward the knees. Sit-ups: In the same position as for the curl-up task, the subjects were instructed to raise the upper torso up to a 45 deg without the curling-up movement. Back extensions on the floor: In a prone position on the floor with the hands behind the head and the legs extended and supported by a researcher, subjects were instructed to raise the upper torso up to a 30 deg angle. Back extensions on a bench: In a prone position on a bench, with the upper torso placed over the end of the bench and bent down vertically to the floor, hands behind the head, and the legs extended and supported by a researcher, the subjects were instructed to raise the torso straight up to a 180 deg angle (parallel to the floor).
EMG measurements and analysisIn the isometric MVC and trunk exercise tasks, the surface EMG activities of rectus abdominis (RA), external oblique (EO), internal oblique (IO), and erector spinae (ES) muscles of the right side were measured by a bipolar configuration using a portable EMG recording apparatus (ME6000T16; MEGA Electronics, Finland). The electrode locations described by Vera-Garcia et al., 2007 were followed and a B-mode ultrasound apparatus (Prosound 2; Aloka, Japan) was used for positioning the electrodes over muscles. Ag-AgCl electrodes of 15 mm diameter (N-00-S Blue sensor; Ambu, Denmark) were attached over the bellies of the muscles with an interelectrode distance of 20 mm after the skin surface was shaved, rubbed with sandpaper, and cleaned with alcohol. Another electrode for each muscle was attached and functioned as a ground electrode as well as a preamplifier. The EMG signals were 412-fold-amplified through the preamplifier, A/D-converted through a band-pass-filter (8-500 Hz/3 dB) at a sampling frequency of 2,000 Hz, and stored on a personal computer together with the hip joint angle data for later analysis. From EMG data, the root-mean-square (RMS) amplitude of EMG for each muscle was calculated using data analysis software (Chart version 7; ADInstruments, Australia). In the MVC task, the peak amplitude of EMG (EMGmax) in each muscle was determined over a 500-ms window centered with the time at which peak torque was attained. For each of the RA, EO, and IO muscles, higher EMG amplitude obtained during either trunk flexion or trunk lateral flexion was adopted as EMGmax, and trunk extension was used for ES muscle. The EMGs of each muscle during trunk exercise tasks are expressed as the value relative to its maximum (% EMGmax). EMGs during static exercises were analyzed in an 8-s window following the first 1 s after steady contractions were achieved. EMGs during dynamic exercises were analyzed for those during the 3rd – 7th repetitions based on the hip joint angle data and averaged over the 5 repetitions. The repeatability of % EMGmax measurements during the prescribed tasks was assessed on 2 separate days in a pilot study with 5 young adult men. In each of the prescribed tasks, there was no significant difference between the % EMGmax values of the two measurements for each muscle tested. The mean values of ICCs and CVs for % EMGmax values during the trunk exercises were 0.821 and 10.1%, respectively, for RA, 0.861 and 9.2%, respectively, for EO, 0.792 and 14.7%, respectively, for IO, and 0.833 and 9.7%, respectively, for ES.
Statistical analysisDescriptive data are shown as means ± SDs. A one-way ANOVA and Bonferroni post hoc test was used to test the differences in the % EMGmax values among the muscles during abdominal bracing task. A two-way ANOVA (muscle × exercise) was used to test the effects of muscle and exercise and their interaction on % EMGmax value. When a significant interaction was found, a one-way ANOVA and Dunnett’s post hoc test was used to test differences in the % EMGmax values during exercises compared with abdominal bracing for each of the muscles. In addition, effect size (Cohen’s d) was calculated to express the magnitude of the difference between the two means of % EMGmax. The threshold level values were < 0.20 (trivial), 0.20 – 0.49 (small), 0.50 – 0.79 (medium), and ≥ 0. 80 (large) (Faul et al., 2007). Statistical significance was set at p < 0.05. All data were analyzed using SPSS software (SPSS statistics 20; IBM, Japan).
The % EMGmax values in each muscle during the abdominal bracing were 18% in RA, 27% in EO, 60% in IO, and 19% in ES, with a significantly higher value in IO than in the other muscles (p < 0.05, Cohen’s d = 1.10 – 1.50) (Figure 2). In the comparison among the exercises, there was a significant interaction between muscle and exercise for % EMGmax values. The % EMGmax values for RA, EO, and ES were significantly lower in the abdominal bracing than in some of the other exercises such as V-sits and sit-ups for RA and EO and back extensions for ES muscle (p < 0.05, Cohen’s d = 0.80 – 3.80) (Figures 3, 4, 6). However, the % EMGmax value for IO during the abdominal bracing was significantly higher than those in most of the other exercises including dynamic ones such as curl-ups and sit-ups (p < 0.05, Cohen’s d = 0.79 – 1.69) (Figure 5).
The main findings of the study were that 1) the % EMGmax value during abdominal bracing was significantly higher in IO than in the other muscles, and 2) while the % EMGmax values for RA, EO, and ES were significantly lower in the abdominal bracing than in some of the other exercises, the % EMGmax value for IO during the abdominal bracing was significantly higher than those in most of the other exercises including the dynamic ones. These results indicate that abdominal bracing is one of the most effective techniques for inducing a higher activation in IO muscle, even compared to dynamic exercises involving trunk flexion/extension movements. It has been suggested that IO muscle plays a large role in creating abdominal bracing maneuvers (Vera-Garcia et al., 2006; 2007). In fact, Vera-Garcia et al., 2010 observed a higher activation of IO muscle during abdominal bracing. The current result agrees with this and indicates that the activation level during abdominal bracing distinctly differs between IO muscle and the other three muscles. The internal oblique muscles, together with the transversus abdominis muscles, are considered to be key deep abdominal muscles that contribute to the stability of the spine during physical movements in both athletic and daily events (Rasouli et al., 2011; Teyhen et al., 2008). However, it is also suggested that all trunk muscles play an important role in achieving spinal stability and must work harmoniously to reach this goal (Grenier and McGill, 2007; McGill et al., 2003). Taking these aspects into account together with the current results, it can be considered that abdominal bracing is a modality which induces selectively higher activity in the deep abdominal muscles of the trunk musculature which harmoniously work to stabilize the spine. The % EMGmax values in each muscle during abdominal bracing were 18% in RA, 27% in EO, 60% in IO, and 19% in ES muscles. These values are similar to those (RA: 20 – 25%, EO: 30 – 60%, IO: 50 – 80%, ES: 10 – 40%) reported in previous studies (Bressel et al., 2011; Vera-Garcia et al., 2010). However, as shown in the current and previous studies (Bressel et al., 2011; Vera-Garcia et al., 2010), it should be noted that all of the trunk muscles cannot be fully activated under abdominal bracing with maximal effort. The reason for this phenomenon is unknown. Pashler, 1994 indicated that when two tasks are performed simultaneously, the performance of each is often impaired. This phenomenon is referred to as dual-task interference (Pashler, 1994), and it often occurs even when performing relatively simple tasks, especially when the task is unfamiliar. Considering this, it seems that the task requiring simultaneous contractions of multiple muscles induces a similar phenomenon to the dual-task interference, and it might have resulted in the lower % EMGmax values during the bracing task. Furthermore, as described earlier, abdominal bracing can be considered a mode of antagonist co-contraction (Cholewicki et al., 1999; Gardner-Morse and Stokes, 2001). Some studies reported that an influence of reciprocal inhibition might be assumed to be involved as a factor limiting the maximal activation of antagonistic muscles (Serrau et al., 2011; Tyler and Hutton, 1986). If this finding can be applied to abdominal bracing, it seems that the antagonistic pairs located in the trunk might never reach their maximum level of muscle activation due to neural inhibition during voluntary co-contraction. Another interesting finding obtained here was that while % EMGmax values for RA, EO, and ES were significantly lower in the abdominal bracing than in some of the other exercises such as V-sits and sit-ups for RA and EO and back extensions for ES muscle, the % EMGmax value for IO during the abdominal bracing was significantly higher than those in most of the other exercises such as sit-ups and curl-ups. This implies that abdominal bracing is one of the most effective techniques for inducing a higher activation in deep abdominal muscles, such as IO muscle, even compared to dynamic exercises involving trunk flexion/extension movements. From an athletic perspective, dynamic exercises involving spine flexion and extension are usually preferred for strengthening the trunk muscles (Hibbs et al., 2008). In addition, a recent review article (Martuscello et al., 2013) suggested that multi-joint free weight exercises, rather than trunk-specific exercises, should be implemented in training programs in order to adequately strengthen the trunk muscles. These types of exercise, however, are recommended for advanced trained individuals because the lumbar spine is subjected to high loads, which are not advisable for inexperienced individuals or patients with spine instability, spine lesion, or lower back pain (Monfort-Panego et al., 2009). Therefore, from a clinical point of view, static exercises are usually recommended for rehabilitation and/or fitness programs at the expense of muscular activity. In IO muscle, nevertheless, abdominal bracing showed greater activity than most of the other exercises including dynamic ones. This suggests that IO muscle is not highly activated by most exercises conducted in many athletic and rehabilitative programs, but is selectively recruited by such specific exercise as abdominal bracing. It is recommended that in the initial stage of spine-strengthening programs, participants should be instructed to become aware of motor patterns and to recruit muscles in isolation (Hibbs et al., 2008). These programs can then progress to functional positions and dynamic activities (Akuthota and Nadler, 2004). It is also suggested that trunk stability training should range from isolated activation of the deep abdominal muscles, such as internal oblique or transversus abdominis, to lifting weights on uneven surfaces (Hibbs et al., 2008). This is due to the different functional roles of the muscles during specific exercise tasks. Therefore, it is advised that exercises should be performed to activate the trunk musculature in all three planes and full ranges of motion for developing total spine stability (Bergmark, 1989). In addition, trunk stabilization and trunk-strengthening programs that target the deep abdominal muscles have been designed to improve motor control and strength of the trunk region (Teyhen et al., 2008). Considering these, abdominal bracing should be included in both rehabilitation and athletic training programs when the goal is to improve spine stability. However, as demonstrated by the present study, it should be noted that abdominal bracing is not the best exercise for maximizing the activities of all the trunk muscles. In view of establishing the efficacy of a training modality for improving muscle function, it must be considered whether the muscle activation during the exercise is sufficient in terms of training intensity. A recent study (MacKenzie et al., 2010) reported that a resistance training program, in which subjects performed voluntary co-contractions of antagonistic pairs (elbow flexors and extensors), produced significant increases in the strength capability of both muscle pairs without the use of an external load as resistance. Although the intensity level during maximal voluntary co-contraction exercise was not discussed in their study, other studies showed that muscular activity levels of elbow flexors and extensors during the task were about 40 – 70% of those during MVC (Serrau et al., 2011; Tyler and Hutton, 1986). Hence, the 60% EMGmax value in IO muscle as shown in the current study can be considered sufficient to be a training stimulus for improving the function of the deep abdominal muscles. Hides et al., 2012 applied a training modality with isometric voluntary contractions of abdominal muscles plus abdominal drawing in upright and forward lean positions to Australian Football League players with or without lower back pain. As a result, they observed that the training modality had more positive effect on multifidus CSA than a Pilates program with abdominal drawing in horizontal positions. This finding supports the assumption mentioned above and, at the same time, indicates that abdominal bracing can be a training modality for strengthening the muscle groups which function to stabilize spine even for athletes. In any case, no study has tried to examine how a training modality with abdominal bracing influences neuromuscular function which may contribute to spinal stability. Further study is needed to clarify this.
In summary, abdominal bracing was shown to be one of the most effective exercise techniques for IO muscle even compared to dynamic exercises involving trunk flexion/extension movements. Thus, abdominal bracing should be included in exercise programs when the goal is to improve trunk stability, although further investigation focusing on its actual effects on spinal stability in rehabilitation and/or athletic event is needed.
ACKNOWLEDGEMENTS |
This research was supported by the Sasakawa Scientific Research Grant from The Japan Science Society (24-620). |
|
AUTHOR BIOGRAPHY |
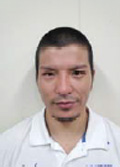 |
Sumiaki Maeo |
Employment: National Institute of Fitness and Sports in Kanoya, Research Fellow of Japan Society for the Promotion of Science |
Degree: MSc |
Research interests: Exercise physiology, Neurosciece |
E-mail: smaeo1985@gmail.com |
|
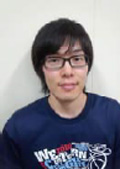 |
Takumi Takahashi |
Employment: National Institute of Fitness and Sports in Kanoya |
Degree: BSc |
Research interests: Exercise physiology |
E-mail: m126007@sky.nifs-k.ac.jp |
|
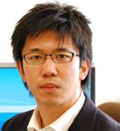 |
Yohei Takai |
Employment: National Institute of Fitness and Sports in Kanoya |
Degree: PhD |
Research interests: Exercise physiology, Aging, Growth and development |
E-mail: y-takai@nifs-k.ac.jp |
|
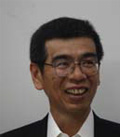 |
Hiroaki Kanehisa |
Employment: National Institute of Fitness and Sports in Kanoya |
Degree: PhD |
Research interests: Exercise physiology |
E-mail: hkane@nifs-k.ac.jp |
|
|
|
REFERENCES |
 Akuthota V., Nadler S.F. (2004) Core strengthening. Archives of Physical Medicine and Rehabilitation 85, S86-92.
|
 Allison G.T., Godfrey P., Robinson G. (1998) EMG signal amplitude assessment during abdominal bracing and hollowing. Journal of Electromyography and Kinesiology 8, 51-57.
|
 Behm D.G., Drinkwater E.J., Willardson J.M., Cowley P.M. (2010) The use of instability to train the core musculature. Applied Physiology, Nutrition, and Metabolism 35, 91-108.
|
 Bergmark A. (1989) Stability of the lumbar spine. A study in mechanical engineering. Acta Orthopaedica Scandinavica Supplementum 230, 1-54.
|
 Bressel E., Dolny D.G., Gibbons M. (2011) Trunk muscle activity during exercises performed on land and in water. Medicine and Science in Sports and Exercise 43, 1927-1932.
|
 Bressel E., Dolny D.G., Vandenberg C., Cronin J.B. (2012) Trunk muscle activity during spine stabilization exercises performed in a pool. Physical Therapy in Sport 13, 67-72.
|
 Cholewicki J., Juluru K., McGill S.M. (1999) Intra-abdominal pressure mechanism for stabilizing the lumbar spine. Journal of Biomechanics 32, 13-17.
|
 Faul F., Erdfelder E., Lang A.G., Buchner A. (2007) G*Power 3: a flexible statistical power analysis program for the social, behavioral, and biomedical sciences. Behavior Research Methods 39, 175-191.
|
 Gardner-Morse M.G., Stokes I.A. (2001) Trunk stiffness increases with steady-state effort. Journal of Biomechanics 34, 457-463.
|
 Grenier S.G., McGill S.M. (2007) Quantification of lumbar stability by using 2 different abdominal activation strategies. Archives of Physical Medicine and Rehabilitation 88, 54-62.
|
 Hibbs A.E., Thompson K.G., French D., Wrigley A., Spears I. (2008) Optimizing performance by improving core stability and core strength. Sports Medicine 38, 995-1008.
|
 Hides J.A., Stanton W.R., Mendis M.D., Gildea J., Sexton M.J. (2012) Effect of motor control training on muscle size and football games missed from injury. Medicine and Science in Sports and Exercise 44, 1141-1149.
|
 Kibler W.B., Press J., Sciascia A. (2006) The role of core stability in athletic function. Sports Medicine 36, 189-198.
|
 MacKenzie S.J., Rannelli L.A., Yurchevich J.J. (2010) Neuromuscular adaptations following antagonist resisted training. Journal of Strength and Conditioning Research 24, 156-164.
|
 Marshall P.W., Desai I., Robbins D.W. (2011) Core stability exercises in individuals with and without chronic nonspecific low back pain. Journal of Strength and Conditioning Research 25, 3404-3411.
|
 Martuscello J.M., Nuzzo J.L., Ashley C.D., Campbell B.I., Orriola J.J., Mayer J.M. (2013) Journal of Strength and Conditioning Research. Systematic review of core muscle activity during physical fitness exercises. (In press).
|
 McGill S.M. (2001) Low back stability: from formal description to issues for performance and rehabilitation. Exercise and Sport Sciences Reviews 29, 26-31.
|
 McGill S.M., Grenier S., Kavcic N., Cholewicki J. (2003) Coordination of muscle activity to assure stability of the lumbar spine. Journal of Electromyography and Kinesiology 13, 353-359.
|
 Monfort-Panego M., Vera-Garcia F.J., Sanchez-Zuriaga D., Sarti-Martinez M.A. (2009) Electromyographic studies in abdominal exercises: a literature synthesis. Journal of Manipulative and Physiological Therapeutics 32, 232-244.
|
 Pashler H. (1994) Dual-task interference in simple tasks: data and theory. Psychological Bulletin 116, 220-244.
|
 Rasouli O., Arab A.M., Amiri M., Jaberzadeh S. (2011) Ultrasound measurement of deep abdominal muscle activity in sitting positions with different stability levels in subjects with and without chronic low back pain. Manual Therapy 16, 388-393.
|
 Serrau V., Driss T., Vandewalle H., Behm D.G., Lesne-Chabran E., Le Pellec-Muller A. (2011) Muscle activation of the elbow flexor and extensor muscles during self-resistance exercises: comparison of unilateral maximal co-contraction and bilateral self-resistance. Journal of Strength and Conditioning Research 26, 2468-2477.
|
 Teyhen D.S., Rieger J.L., Westrick R.B., Miller A.C., Molloy J.M., Childs J.D. (2008) Changes in deep abdominal muscle thickness during common trunk-strengthening exercises using ultrasound imaging. The Journal of Orthopaedic and Sports Physical Therapy 38, 596-605.
|
 Tyler A.E., Hutton R.S. (1986) Was Sherrington right about co-contractions?. Brain Research 370, 171-175.
|
 Urquhart D.M., Hodges P.W., Allen T.J., Story I.H. (2005) Abdominal muscle recruitment during a range of voluntary exercises. Manual Therapy 10, 144-153.
|
 Vera-Garcia F.J., Brown S.H., Gray J.R., McGill S.M. (2006) Effects of different levels of torso coactivation on trunk muscular and kinematic responses to posteriorly applied sudden loads. Clinical Biomechanics 21, 443-455.
|
 Vera-Garcia F.J., Elvira J.L., Brown S.H., McGill S.M. (2007) Effects of abdominal stabilization maneuvers on the control of spine motion and stability against sudden trunk perturbations. Journal of Electromyography and Kinesiology 17, 556-567.
|
 Vera-Garcia F.J., Moreside J.M., McGill S.M. (2010) MVC techniques to normalize trunk muscle EMG in healthy women. Journal of Electromyography and Kinesiology 20, 10-16.
|
 Willardson J.M. (2007) Core stability training: applications to sports conditioning programs. Journal of Strength and Conditioning Research 21, 979-985.
|
|
|
|
|
|
|