|
|
|
ABSTRACT |
In this study we examined how hill running affects the Achilles tendon, a common location for injuries in runners. Twenty females ran for 10 min on each of three randomly ordered grades (-6%, 0 and +6%) at speeds selected to match the metabolic rates. Achilles tendon (AT) cross-sectional area (CSA) was imaged using Doppler ultrasound and peak vertical forces were analyzed using an instrumented treadmill. A metabolic cart and gas analyzer ensured a similar metabolic cost across grades. Data were analyzed using a forward selection regression. Results showed similar decreases in AT CSA from pre- to post-run for all three conditions of ~5 to 7% (p = 0.0001). Active peak vertical forces were different across grades (p = 0.0001) with the largest occurring during downhill running and smallest during uphill running. Since changes in AT CSA were not different between grades, each form of running appears equal and acceptable in regards to how the Achilles tendon reacts. That is, the results suggest that the Achilles tendon is affected by downhill, level, and uphill running and a decrease in CSA appears to be a normal response. |
Key words:
Doppler ultrasound imaging, cross-sectional area, peak vertical force
|
Key
Points
- Downhill (- 6%), level and uphill (+ 6%) running at different speeds each caused a statistically significant decrease in the Achilles tendon cross-sectional area in healthy, trained runners.
- The magnitude of change in Achilles tendon cross-sectional area did not differ between grades when metabolic cost of running was matched.
- Downhill running resulted in the largest peak vertical force, while uphill running resulted in the smallest.
|
Hill running can provide many health benefits to runners, but there are also potential associated risks (Ferley et al., 2013; Jamurtas et al., 2013; Koji et al., 2012). Among benefits are improved cardiovascular conditioning and increased strength (Telhan et al., 2010), favorable blood lipid changes (Jamurtas et al., 2013), reduced risk of injury due to an adaptive effect of training (Proske and Morgan, 2001), and improved performance with specificity of exercise. Understanding physical changes to body tissues during hill running is important for training and competition. Of particular relevance is how hill running affects the Achilles tendon (AT), which is a common location for injuries in runners. An estimated 52% of recreational and competitive distance runners have an AT injury at some point (Kujala et al., 2005). The progressive lengthening of an elastic structure when it is cyclically loaded (creep), as in running, may be indicative of micro-damage to the AT (Ker et al., 2000) and consequently, indicative of injury risk (Farris et al., 2012; Kongsgaard et al., 2005; Pang et al., 2006; Ying et al., 2003). One method of monitoring tendon size changes is to measure the cross-sectional area (CSA) using ultrasound imaging (Farris et al., 2011). The properties of tendons allow them to stretch withstand tension, and change length and thickness, thus allowing for economical locomotion (Wilson and Lichtwark, 2011). Tendons stretch up to 10% of their resting length, so this gives the tendon a large stretch ability relative to the length of the muscle (Wilson and Lichtwark, 2011). Recent in vivo studies that used ultrasound have shown that the human AT rapidly lengthens and shortens during the stance phase of running, which stores and returns elastic energy (Ker et al., 2000; Magnusson and Kjaer, 2003; Wilson and Lichtwark, 2011). The reutilization of this elastic energy in tendons greatly reduces energy demands in running (Cavagna et al., 1964). Researchers claim that the stretch-recoil of the tendon reduces the work that the muscle must perform and thus reduces energetic cost (Arampatzis et al., 2006; Lichtwark and Wilson, 2006; Wilson and Lichtwark, 2011). Tendons have been shown to be adaptable (Magnusson et al., 2008), but whether the tendon adapts to physical activity by changing the CSA remains unknown (Magnusson and Kjaer, 2003). Conflicting study results report that during running the tendon cross-sectional area increased (Sommer, 1987; Woo et al., 1980; 1982), remained unchanged (Buchanan and Marsh, 2001), or decreased (Tardioli, 2011; Woo et al., 1982). There remains uncertainty as to how AT CSA responds to exercise. Of particular interest is grade running, that is uphill and downhill running, and how it relates to injury risk. Gottschall and Kram (2005) compared peak impact and active ground reaction forces during uphill and downhill running. Their impact force data suggest that downhill running substantially increases the likelihood of an overuse running injury, but no active force data was affected by grade running. The Boston, Chicago, New York City, and Los Angeles marathons include hill grades ranging from 0.2% to 6%. In selecting a test grade for research, 6% would realistically be seen in racing and has also been shown to produce optimal running economy (Minetti et al., 2002). We wanted to study downhill running compared to uphill running, taking into account Achilles tendon CSA and active peak vertical forces. The primary purpose of this study was to determine the relationship between the grade of the running surface and the change in AT CSA in a population of female runners. We hypothesized: (1) the percent change of AT CSA would have the greatest decrease after running on the incline grade, (2) the percent change of AT CSA would be the least after downhill running, and (3) the percent change of AT CSA would decrease after running on the level grade.
Research designThis study was a crossover design. Each individual was measured three times on different days and in different running grade conditions. We used Latin-square randomization, with participants drawing their treatment order from a container. Intra-individual comparisons were made with the independent variable being grade of the running surface (-6%, 0%, +6%). The dependent variables were change in Achilles tendon cross-sectional area from pre-run to post-run measurement, peak vertical force and plantar-flexion velocity.
ParticipantsTwenty female runners (age = 20.7 ± 1.8y, height = 1.65 ± 0.06m, mass = 60.5 ± 7.2kg, weekly mileage run = 26.8 ± 12.1km, fastest 5000m time = 18:31.59 ± 2:11.32 min, mean ± standard deviation) volunteered for this study. They ranged from recreational to collegiate Division I runners. None of the participants had suffered an Achilles tendon injury within six months or a lower extremity injury within three months of data collection, or were pregnant. Inclusion criterion was current capability to run 5000m in under 24:00 min. All procedures were granted approval by the Institutional Review Board. Power assessment indicated that with a SD of 0.04, 16 participants were needed to detect a significant difference of 4 mm2 between pre- and post-run AT CSA measurements (p = 0.05, ß = 0.8). We collected data on 20 participants, assuming a 20% dropout rate. All participants completed the study.
ProceduresEach approved participant came to the Human Performance Research Center Biomechanics lab on the Brigham Young University campus on three separate days. The time between visits was at least 48 hours, which time period was found to be adequate for a return to baseline pre-run measurements of AT CSA during pilot work. Participants came at approximately the same time of day for each visit. Participants completed a pre-participation questionnaire to determine eligibility before any data collection. Prior to arrival, each participant received instructions to maintain normal eating and hydration habits, refrain from eating a meal within two hours of testing, follow normal sleep patterns and avoid any exercise on test day previous to testing. Accordance with these parameters was assured by the pre-participation questionnaire. On the first visit they signed an approved Informed Consent form.
Data collectionAt the start of each of three visits, the participant rested prone on a treatment table for 15 minutes to adapt to lab conditions, similar to a recently established protocol (Kubo and Ikebukuro, 2012). A pillow under the ankles created a neutral resting position at the ankle joint. Following the rest period, a strap connected to the end of the table was fit around each foot to create a 90 degree angle at the ankle, which was measured by a goniometer. Doppler ultrasound (GE Logic E, GE Healthcare, Little Chalfont, UK) was used to image the Achilles tendon with a 12L probe. Test and retest reliability had an ICC3,1 of 0.97 (95% CI: 0.959, 0.986). Ultrasound transmission gel (Accelerated Care Plus, UG250, CAN) was applied to the head of the probe to collect three transverse images of the AT CSA before and after running. Images were taken between the lateral and medial malleolus. An oblique line was made between the two landmarks and the midway location was marked with a permanent marker to ensure consistency within and between trials. High-speed videography (VICON Motion Technologies, Centennial, CO, USA) was used for kinematic and kinetic data collection. Ten cameras (240 Hz) recorded the motion of the runners. A wide sliding caliper was used for anthropometric measurements including: leg length (ASIS to medial malleolus), ASIS-trochanter distance (in the sagittal plane), inter-ASIS distance, knee width, and ankle width (Scholz et al., 2008), which measurements were input into the individual subject profile on VICON. Reflective markers were placed on the participant according to the VICON Plugin Gait setup. Sixteen total markers were placed bilaterally using double-sided adhesive tape on the lower extremity over the following anatomical landmarks: anterior and posterior superior iliac spines, mid-lateral thigh, lateral knee joint center, mid-lateral shank, lateral malleolus, posterior heel, and head of second metatarsal (Figure 1). Participants ran on an instrumented treadmill (AMTI, Watertown, MA) at three different grades on three separate days. They ran in their personal running shoes, and the sequence of running grades was randomly selected for each person (-6%, 0%, +6%). The runner ran at a speed of 3.13m/s (7 mph) on the 0% grade, 3.80 m/s (8.5 mph) on the -6% grade, and 2.46m/s (5.5 mph) on the +6% grade. These differing speeds were determined in pilot testing to elicit similar metabolic cost (39.5 ± 2.9 mL/kg/min). A metabolic cart and gas analyzer (TrueMax 2400, Metabolic Measurement System, PARVO Medics, UT, USA) were used to ensure similar metabolic cost across running grades via collection of steady-state oxygen consumption data. The subject ran at the designated grade for 10 min (Figure 2). These speeds and time period were chosen to represent recovery running for a trained female as well as to minimize fatigue and prevent any confounding effects that fatigue may have on muscle and tendon behavior (Farris et al., 2012). Avoiding overall or whole-body fatigue or tiring, and to aide in obtaining unaffected pre-run measurements were the reasons there was no warm-up.
Data processingThree images were taken of each right and left AT CSA, outlined manually using internal software of the GE Logic, and averaged (Farris et al., 2011). The right and left CSA were then averaged together for pre-run measurements and post-run measurements for each of the grade conditions. Position data, collected from the locations of the reflective markers, were reconstructed into three-dimensional coordinates using VICON Nexus software (VICON Motion Technologies, Centennial, CO, USA). The instrumented treadmill recorded active peak vertical ground reaction forces at 1200 Hz. A static trial was recorded before running, where the participant stood still on the treadmill for 1 s in anatomical position but with palms facing medially. These coordinate data were smoothed with a 50 Hz low-pass Butterworth filter. Kinetic data were recorded for 10 s at the zero, second, fourth, sixth, eighth and tenth minute of running. We used the data from the eighth minute for analysis, which included the last 2 min of the 10-min run. This ensured that subjects were thoroughly warmed-up physiologically and biomechanically prior to this part of data collection. The data were then imported into custom-made software using Microsoft Visual Basic 2012 (Microsoft Corporation, Redmond, WA, USA) for additional calculations. Software analyzed and averaged peak vertical force and plantar-flexion velocity for 8 steps of the runner.
Statistical analysisA forward selection regression was performed to determine the effect of grade on Achilles tendon cross-sectional area, peak vertical force and plantar-flexion velocity. The alpha level was set at α ≤ 0.05. Leg dominance and foot length were used as co-variates in the analysis. Statistical analysis was performed using Statistical Analysis Software (SAS Version 9.3, SAS Institute, Inc. Cary, NC, USA).
Table 1 lists the results, means, standard deviations and statistical significances for the dependent variables. There was a significant change in AT CSA from pre-run to post-run measurement (F = -6.62, p < 0.001). The average pre-run value was 0.39 cm2 while the average post-run value was 0.36 cm2. The average percent changes were as follows: -7.14% for downhill running, -6.30% for level running, and -4.87% for uphill running. While this looks like a trend across surfaces, the grades were non-significant (F = 1.29, p = 0.284). Active peak vertical forces were statistically different from one another across grades (F = 45.54, p < 0.001). The greatest average peak force was 2.72 BW for the downhill grade, the second largest was 2.52 BW for the level grade, and the smallest was 2.36 BW for the uphill grade. Average plantar-flexion velocity during uphill running was statistically different from downhill running (p = 0.013) and level running (p = 0.039). Leg dominance (p = 0.013) and foot length (p = 0.035) were significant covariates in analysis of plantar-flexion velocity.
The aim of this study was to determine the relationship between the grade of the running surface (-6%, 0%, +6%) and AT CSA changes in female runners. Our findings suggest that running does indeed affect AT CSA. Running at a consistent energy output (39.5 ± 2.9 mL·kg-1·min-1) for a ten minute run at submaximal effort caused on average between 4.87 and 7.14% decreases in AT CSA. Our measurement of AT CSA in female runners (0.39 cm2) was lower than previously published findings of samples which included men and women and various age ranges (0.43 cm2 to 0.71 cm2) (Farris et al, 2012; Kongsgaard et al., 2005; Pang et al., 2006; Ying et al., 2003). We attribute the smaller tendon CSA in our study to be the result of our participants being all female and that they were of a similar slender and smaller body structure. The decrease in AT CSA noted from pre to postrun is thought to be the result of tendon stretching and thinning (Lichtwark and Wilson, 2006). AT CSA decreased in every condition and there were no significant differences in AT CSA changes between conditions. We reject our hypotheses that the greatest decrease in AT CSA would occur after uphill running and the smallest decrease would occur after downhill running. The lack of significance in our results may be because the AT of the trained runners was already adapted to the loads of running (Magnusson and Kjaer, 2003). The AT has been noted for its dynamic behavior during exercise (Farris et al., 2012) and its adaptable nature affords it the ability to respond to changing demands (Magnusson et al., 2008). From pre- to post-run AT CSA, the decreases were between 4.87 and 7.14%. We thought there would be greater tendon changes across grades because of different forces that hill running causes. Hill running seems demanding on a runner because it requires synchronized muscular contraction around the hips, knees, and ankles while simultaneously supporting a runner’s full body mass. We did find significant differences in active peak vertical force across grade conditions. The peak force for level running at 3.13 m·s-1 was 2.52 BW. This agrees with the previously reported value of 2.5 BW reported at a pace of 3 m·s-1, which force was just slightly smaller and pace slightly slower than ours (Gottschall and Kram, 2005). We reported the greatest peak vertical force occurred during running on a downhill grade and the smallest peak force on the uphill grade, +8% larger and -6% smaller when compared to level running, respectively. The results obtained in our study agree with those of previous researchers and support the theory that uphill, downhill and level grade conditions result in different peak vertical forces (Iversen and McMahon, 1992; Hreljac et al., 2000). Our findings differ from a study which found that neither downhill nor uphill running affected active peak vertical force. This is likely because our study matched metabolic cost across grades, while theirs used the same speed across grades. (Gottschall and Kram, 2005). Because changes in AT CSA were not different between downhill, level, and uphill running, each form of running appears equal and acceptable in regards to how the Achilles tendon is impacted. That is, the results suggest that the Achilles tendon is affected by running and a decrease in CSA appears to be a normal response. However when considering forces, since downhill running has previously been shown as more likely to result in an overuse injury due to increased forces (Gottshall and Kram, 2005), runners should transition slowly to this type of training in order to adapt to these greater forces. Other force data suggests that the probability for musculoskeletal injury increases during downhill running and decreases during uphill running (Hreljac et al., 2000). Though active peak vertical forces were significantly different across grades, they did not cause significantly different changes in AT CSA across grades at metabolically matched speeds. Reasons that elucidate the benefits or harm in the extent of AT CSA changes, along with the exact reasoning why these changes occur are yet to be understood. The participants were trained and well-accustomed to the demands of running. They were also habituated to running at a moderate pace for durations much longer than ten minutes, which was the running time in our study. Our study design did not include sufficient time or intensity for the runners to experience notable fatigue. Faster running speeds result in greater muscle-tendon forces, joint loads and cartilage stresses (Harrison et al., 2014). Uphill running causes greater ankle range of motion while the foot is in contact with the ground (Swanson and Caldwell, 2000). We found no difference in CSA changes across grades. The lack of differences can perhaps be explained by greater force on the tendon in the faster downhill trials, but also a smaller ankle range of motion. Conversely, there is a larger ankle range of motion and less force on the tendon in the slower uphill trials. This was a single study and to fully understand the effect of uphill and downhill running on CSA, future research should look at constant speed running across grades. Other future studies should look at how duration of run and recovery time between exercise bouts affects CSA. There were some limitations related to this study. All participants were trained runners so results may have been skewed because of well-adapted tendons. There may be a greater change in AT CSA among untrained participants, because of the lack of adaptation of the AT. Reflective markers were manually placed on the anatomical landmarks for each participant, so they may have been placed on slightly different positions of the body for each running session. We made one main inference. The order of grade treatment (-6%, 0%, +6%) was randomly assigned, so we can claim that the changes in active peak vertical force and plantar-flexion velocity were caused by the individual grade and speed combinations. Participants were not randomly selected from a population, so results may only be attributable to a population of female runners with similar characteristics.
Downhill, uphill and level running each cause a statistically significant decrease in the AT CSA. The AT CSA does not differ between grades when metabolic cost of running is matched. The Achilles tendon is capable of adapting and this may have been the case with our participants, as our study used all trained runners. Downhill running resulted in the largest peak vertical force, while uphill running resulted in the smallest. Since changes in AT CSA were not different between grades, each form of running appears equal and acceptable in regards to how the Achilles tendon reacts. That is, our results suggest that the Achilles tendon is affected by downhill, level, and uphill running and a decrease in CSA appears to be a normal response.
|
AUTHOR BIOGRAPHY |
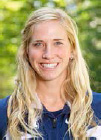 |
Katy Andrews Neves |
Employment: Department of Exercise Sciences, Brigham Young University. |
Degree: MSc |
Research interests: Performance in sport (distance running) |
E-mail: katyandrews100@gmail.com |
|
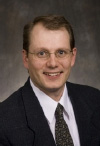 |
A. Wayne Johnson |
Employment: Department of Exercise Sciences, Brigham Young University. |
Degree: PhD, PT |
Research interests: Rehabilitation and rehabilitative ultrasound imaging of spinal, leg and foot muscles, Minimalist shoe running, Whole-body vibration, Flexibility, and outcomes of rehabilitation. |
E-mail: wayne_johnson@byu.edu |
|
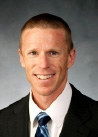 |
Iain Hunter |
Employment: Department of Exercise Sciences, Brigham Young University. |
Degree: PhD |
Research interests: Biomechanics of distance running related to performance and injury |
E-mail: iain_hunter@byu.edu |
|
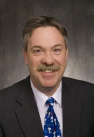 |
J. William Myrer |
Employment: Department of Exercise Sciences, Brigham Young University. |
Degree: PhD |
Research interests: Diagnostic Ultrasound, Flexibility, Topical Analgesics, Therapeutic Modalities, Rehabilitation. |
E-mail: bill_myrer@byu.edu |
|
|
|
REFERENCES |
 Arampatzis A., De Monte G., Karamanidis K., Morey-Klapsing G., Stafilidis S., Brüggemann G.-P. (2006) Influence of the muscle-tendon unit's mechanical and morphological properties on running economy. The Journal of Experimental Biology 209, 3345-3357.
|
 Buchanan C.I., Marsh R. L. (2001) Effects of long-term exercise on the biomechanical properties of the Achilles tendon of guinea fowl. Journal of Applied Physiology 90, 164-171.
|
 Cavagna G.A., Saibene F.P., Margaria R. (1964) Mechanical work in running. Journal of Applied Physiology 19, 249-256.
|
 Farris D.J., Trewartha G., McGuigan M.P. (2011) Could intra-tendinous hyperthermia during running explain chronic injury of the human Achilles tendon?. Journal of Biomechanics 44, 822-826.
|
 Farris D.J., Trewartha G., McGuigan M.P. (2012) The effects of a 30-min run on the mechanics of the human Achilles tendon. European Journal of Applied Physiology 112, 653-660.
|
 Ferley D.D., Osborn R.W., Vukovich M.D. (2013) The effects of uphill vs. level-grade high-intensity interval training on VO, V-max, V-LT, and T-max in well-trained distance runners. Journal of Strength and Conditioning Research 27, 1549-1559.
|
 Gottschall J.S., Kram R. (2005) Ground reaction forces during downhill and uphill running. Journal of Biomechanics 38, 445-452.
|
 Harrison S.M., Whitton R.C., Kawcak C.E., Stover S.M., Pandy M.G. (2014) Evaluation of a subject-specific finite-element model of the equine metacarpophalangeal joint under physiological load. Journal of Biomechanics 47, 65-73.
|
 Hreljac A., Marshall R.N., Hume P.A. (2000) Evaluation of lower extremity overuse injury potential in runners. Medicine and Science in Sports and Exercise 32, 1635-1641.
|
 Iversen J.R., McMahon T.A. (1992) Running on an incline. Journal of Biomechanical Engineering 114, 435-441.
|
 Jamurtas A.Z., Garyfallopoulou A., Theodorou A.A., Zalavras A., Paschalis V., Deli C. K., Nikolaidis M.G., Fatouros I.G., Koutedakis Y. (2013) A single bout of downhill running transiently increases HOMA-IR without altering adipokine response in healthy adult women. European Journal of Applied Physiology 113, 2925-2932.
|
 Ker R.F., Wang X.T., Pike A.V. (2000) Fatigue quality of mammalian tendons. Journal of Experimental Biology 203, 1317-1327.
|
 Koji N., Junichi A., Naomi T., Satsuki U. (2012) Cool water immersion after downhill running suppresses exercise-induced muscle damage in the rat soleus muscle. Journal of Physical Therapy Science 24, 613-616.
|
 Kongsgaard M., Aagaard P., Kjaer M., Magnusson S.P. (2005) Structural Achilles tendon properties in athletes subjected to different exercise modes and in Achilles tendon rupture patients. Journal of Applied Physiology 99, 1965-1971.
|
 Kubo K., Ikebukuro T. (2012) Blood circulation of patellar and Achilles tendons during contractions and heating. Medicine and Science in Sports and Exercise 44, 2111-2117.
|
 Kujala U.M., Sarna S., Kaprio J. (2005) Cumulative incidence of Achilles tendon rupture and tendinopathy in male former elite atheletes. Clinical Journal of Sports Medicine 15, 133-135.
|
 Lichtwark G.A., Wilson A.M. (2006) Interactions between the human gastrocnemius muscle and the Achilles tendon during incline, level and decline locomotion. The Journal of Experimental Biology 209, 4379-4388.
|
 Magnusson S.P., Kjaer M. (2003) Region-specific differences in Achilles tendon cross-sectional area in runners and non-runners. European Journal of Applied Physiology 90, 549-553.
|
 Magnusson S.P., Narici M.V., Maganaris C.N., Kjaer M. (2008) Human tendon behaviour and adaptation, in vivo. Journal of Physiology-London 586, 71-81.
|
 Minetti A.E., Moia C., Roi G.S., Susta D., Ferretti G. (2002) Energy cost of walking and running at extreme uphill and downhill slopes. Journal of Applied Physiology 93, 1039-1046.
|
 Paluska S.A. (2005) An overview of hip injuries in running. Sports Medicine 35, 991-1014.
|
 Pang B., Ying J. (2006) Sonographic measurement of Achilles tendons in asymptomatic subjects variation with age, body height, and dominance of ankle. Journal of Ultrasound in Medicine 25, 1291-1296.
|
 Proske U., Morgan D.L. (2001) Muscle damage from eccentric exercise: mechanism, mechanical signs, adaptation and clinical applications. The Journal of Physiology 537, 333-345.
|
 Scholz M.N., Bobbert M.F., van Soest A.J., Clark J.R., van Heerden J. (2008) Running biomechanics: shorter heels, better economy. Journal of Experimental Biology 211, 3266-3271.
|
 Sommer H.M. (1987) The biomechanical and metabolic effects of a running regime on the Achilles-tendon in the rat. International Orthopaedics 11, 71-75.
|
 Swanson S.C., Caldwell G.E. (2000) An integrated biomechanical analysis of high speed incline and level treadmill running. Medicine and Science in Sports and Exercise 32, 1146-1155.
|
 Tardioli A. (2011) Structural changes in the Achilles tendon in response to a marathon: ultrasonographically detectable changes immediately and at 2 weeks postmarathon. In: 2nd Congress of European College of Sport and Exercise Physicians (ECOSEP) 12th Scientific Conference, September 9-11, 2010, London. British Journal of Sports Medicine 45, e1-.
|
 Telhan G., Franz J.R., Dicharry J., Wilder R.P., Riley P.O., Kerrigan D.C. (2010) Lower limb joint kinetics during moderately sloped running. Journal of Athletic Training 45, 16-21.
|
 Wilson A., Lichtwark G. (2011) The anatomical arrangement of muscle and tendon enhances limb versatility and locomotor performance. Philosophical Transactions of the Royal Society B-Biological Sciences 366, 1540-1553.
|
 Woo S.L.Y., Gomez M.A., Woo Y.K., Akeson W.H. (1982) Mechanical properties of tendon and ligaments. The relationship of immobilization and exercise on tissue remodeling. Biorhelolgy 19, 397-408.
|
 Woo S.L.Y., Ritter M.A., Amiel D., Sanders T.M., Gomez M.A., Kuel S.C., Garfin S.R., Akeson W.H. (1980) The biomechanical and biochemical-properties of swine tendons - long-term effects of exercise on the digital extensors. Connective Tissue Research 7, 177-183.
|
 Ying M., Yeung E., Li B., Li W., Lui M., Tsoi C. (2003) Sonographic evaluation of the size of Achilles tendon: the effect of exercise and dominance of the ankle. Ultrasound in Medicine & Biology 29, 637-642.
|
|
|
|
|
|
|