|
|
|
ABSTRACT |
This study reports the effects of Q10, coenzyme Q10 or ubiquinone, a component of the electron transport chain in mitochondria, on nuclear factor kappa-light-chain-enhancer of activated B cells (NFθºB), inhibitors of kappa B (IθºB), nuclear factor (erythroid-derived 2)-like 2 (Nrf2) and hemeoxygenase 1 (HO-1) in rats after chronic exercise training for 6 weeks. 8-week old male Wistar rats were assigned randomly to one of four treatments planned in a 2 x 2 factorial arrangement of two condition (sedentary vs. exercise training), and two coenzyme Q10 levels (0 and 300 mg/kg per day for 6 weeks). The expression levels of the target proteins were determined in the heart, liver and muscle, and biochemical parameters including creatinine, urea, glucose and lipid profile were investigated in plasma. When compared with sedentary group, significant decreases in heart, liver and muscle NFθºB levels by 45%, 26% and 44% were observed in Q10 supplemented rats after exercise training, respectively, while the inhibitory protein IθºB increased by 179%, 111% and 127% in heart, liver and muscle tissues. Q10 supplementation caused an increase in Nrf2 (167%, 165% and 90%) and HO-1 (107%, 156% and 114%) after exercise training in heart, liver and muscle tissues (p < 0.05). No significant change was observed in any of the parameters associated with protein, carbohydrate and lipid metabolism, except that exercise caused a decrease in plasma triglyceride, which was further decreased by Q10. In conclusion, these results suggest that Q10 modulates the expression of NFθºB, IθºB, Nrf2 and HO-1 in exercise training, indicating an anti-inflammatory effect of Q10 and emphasizes its role in antioxidant defense. |
Key words:
Coenzyme Q10, exercise, signaling pathway
|
Key
Points
- Coenzyme Q10 is a component of the electron transport chain in mitochondria which is linked to the generation of energy in the cell.
- Coenzyme Q10 may inhibit the peroxidation of lipids, thus acting as an antioxidant and protects tissue against oxidative injury.
- Using of coenzyme Q10 can significantly elevate IθºB, Nrf2 and HO-1 and reduce NFθºB during exercise training.
|
Coenzyme Q10 (or 1,4-benzoquinone) is a component of the electron transport chain in mitochondria which is linked to the generation of energy in the cell (Bentinger et al., 2010). The deficiency of Q10 has been reported to result in poor athletic performance and/or disease pathogenesis including encephalomyopathy, cerebellar ataxia, Leigh syndrome and myopathy (Garrido-Maraver et al., 2014). The Q10 deficiency in skeletal muscle has been shown to show a spectrum of clinical manifestations and suggested to lead to a secondary impairment of mitochondrial fatty acid oxidation (Schaefer et al., 2009). In a study, Q10 has been reported to protect skeletal muscles against exercise-induced injury in rats (Kon et al., 2007). In its reduced form, Q10 holds electrons rather loosely and inhibits the peroxidation of lipids, thus acting as an antioxidant (Mellors and Tappel, 1966; Sarter, 2002), and protects against oxidative injury. Interventions including nutrition, pharmacology and exercise may induce the expression of Nuclear Factor Kappa-light-chain-enhancer of activated B cells (NFθºB) and cellular antioxidant systems via the Nuclear Factor (erythroid-derived 2)-Like 2 (Nrf2)-Kelch-like ECH-associated protein 1 (Keap-1) signaling pathway and play a role in preventing inflammatory processes (Lee et al., 2011). The inducible hemeoxygenase (HO-1) is an antioxidant stress protein, mainly induced by reactive oxygen species (ROS), cytokines and hyperthermia that has been reported to be upregulated in endurance-trained male subjects after a half marathon run (Niess et al., 1999). Despite a need of adequate antioxidant levels to attenuate exercise-induced oxidative damage, a debate exists whether antioxidant supplementations potentiate health outcomes of physical exercise. In contrast supra-physiological dosages of antioxidant supplementations may interfere with ROS-mediated cell signaling and blunt the positive effects of exercise (Atalay et al., 2006). We postulate that Q10 supplementation and exercise-induced protection can be a safe tool to control oxidative stress and inflammation. This study reports that Q10 can modulate the expression of NFθºB, IθºB, Nrf2 and HO-1 after exercise training, supporting the role of Q10 in antioxidant defense and inflammation.
AnimalsTwenty-eight male Wistar rats (age: 8 week, weight: 180 ± 20 g) were housed in a controlled environment with a 12:12-h light-dark cycle at 22 °C and were provided with rat chow and water ad libitum. All experiments were conducted under the National Institute of Health’s Guidelines for the Care and Use of Laboratory Animals and approved by the Ethics Committee of the Firat University. Animals were randomly divided into the following four groups: (i) Control [Sedentary, Group I], (ii) Q10 control [Control diet + Q10, that is, sedentary, but administered with Q10, Group II] (iii) Exercise training [Control diet + subjected to chronic exercise training for six weeks, Group III], and (iv) Q10 supplemented [Control diet + subjected to physical exercise and Q10 treatment, Group IV]. Q10 was administered daily for six weeks as an oral supplement by gastric tube at a dose level of 300 mg/kg body weight. The selection of the dose (300 mg/kg b. wt.) was based on previous studies where this dosage demonstrated a significant antioxidant effect in rat (Kon et al., 2007). Composition of diet fed to animals is shown in Table 1.
Exercise protocolThe rats were subjected to treadmill exercise on a motorized rodent treadmill purchased from Commat Limited, Ankara, Turkey. The treadmill included a stimulus grid at the back end of the treadmill which provided an electric shock if the animal placed its paw on the grid. The apparatus consisted of a 5-lane animal exerciser utilizing single belt construction with dividing walls suspended over the tread surface. All exercise tests were performed during the same time period of the day to minimize diurnal effects. All rats were pre-trained in order for the animals to be exposed to the treadmill equipment and handling for 1 week. For this purpose, animals in the exercise training groups were habituated by treadmill exercise over a 5-day period such that: (i) 1st day, 10 m/min, 10 min, (ii) 2nd day, 20 m/min, 10 min, (iii) 3rd day, 25 m/min, 10 min, (iv) 4th day, 25 m/min, 20 min and (v) 5th day, 25 m/min, 30 min. After 1 week treadmill familiarization to eliminate novel and stress effects, animals in treadmill exercise groups ran on the treadmill 25 m/min, 45 min/day and five days per week for 6 weeks according to the protocol published earlier (Liu et al., 2008).
Sample collectionSedentary and exercise rats were sacrificed via cardiac puncture under ether anesthesia. Exercise groups were sacrificed 48 h after the last exercise. To minimize diurnal effects, animals were killed at the same hour. Within 1 min, the blood sample was transferred into EDTA-coated tube and plasma was separated by centrifugation at 1,750 × g for 10 min. The plasma was stored at -80 °C until the time of analysis. Heart, liver and muscle tissues were rapidly collected and frozen at -80 °C for further analyses.
Laboratory analysesPlasma was used for the determination of urea, glucose and lipid profile, using an automatic analyzer (Samsung LABGEO PT10, Samsung Electronics Co, Suwon, Korea). Repeatability and device/method precision of LABGEOPT10 was established according to the IVR-PT06 guideline. All proteins (NFθºB, IθºB, Nrf2 and HO-1) in the signal transduction pathway were analyzed by Western blot methods in heart, liver and slow-twitch muscles (soleus and gastronemius deep portion). Proteins were extracted from heart, liver and muscle tissues for Western blots (Sahin et al., 2013). For protein analyses an accurately weighed heart, liver or muscle tissues were homogenized in 1:10 (w/v) in 10 mM Tris-HCl buffer at pH 7.4, containing 0.1 mM NaCl, 0.1 mM phenylmethylsulfonyl fluoride and 5 µM soybean (soluble powder; Sigma, St. Louis, MO, USA) as trypsin inhibitor. After centrifugation at 15,000 g at 4°C for 30 min supernatant was transferred into fresh tubes for be assayed at once. Supernatants were mixed with Laemmli’s sample buffer and boiled for 5 min. Aliquots containing 20 µg of protein were subjected to 10% sodium dodecyl sulfate-polyacrylamide gel electrophoresis and subsequently transferred to nitrocellulose membrane (Schleicher and Schuell Inc., Keene, NH, USA). Nitrocellulose blots were washed twice for 5 min in phosphate buffered saline (PBS) and blocked with 1% bovine serum albumin in PBS (Phosphate Buffered Saline) for 1 h prior to the application of primary antibody. Antibodies against NFθºB, IθºB, Nrf2 and HO-1 (Abcam, Cambridge, UK) were diluted (1:1000) in the same buffer containing 0.05% Tween-20. The nitrocellulose membrane was incubated at 4°C with protein antibody at overnight. The blots were washed and incubated with horseradish peroxidase-conjugated goat anti-rat IgG (Abcam). Specific signals were detected using diaminobenzidine and hydrogen peroxide as substrates. Protein loading was controlled using β-actin antibody (Sigma). Samples were analyzed in quadruplicates for each experimental condition and protein levels were determined densitometrically using an image analysis system (Image J; National Institute of Health, Bethesda, USA).
Statistical analysesRats were assigned randomly to one of four treatments planned in a 2 x 2 factorial arrangement of two condition (sedentary vs. exercise training), and two coenzyme Q10 levels (0 and 300 mg/kg). Given that assumption, a sample size of seven per treatment was included in the study. The sample size was calculated based on a power of 85% and P value of 0.05. The data were analyzed using the two-way ANOVA procedure of SPSS (IBM SPSS. 2012 Version 21.0); p < 0.05 was considered as statistically significant. All data are represented as Mean ± Standard Deviation (SD).
Liver and kidney functions tests and plasma glucose and lipid profile in exercise and Q10 supplemented rats after exercise trainingTable 2 shows the results of biochemical parameters including creatinine, urea, glucose and lipid profile in trained rats. Except for plasma creatinine and triglyceride, biochemical variables were not affected by exercise, coenzyme Q10 (q10) supplementation and exercise x Q10 interaction. Plasma creatinine was not affected by exercise and Q10 supplementation, but there was 3.9% decreases in physical exercise and Q10 treatment group’s plasma creatinine levels compared to sedentary untreated rats (exercise x Q10 interaction; p < 0.05). Exercised rats had less plasma triglyceride (0.64 vs. 0.91 mg/dL) concentration than sedentary rats (p < 0.001). Neither there was a main effect of supplemental Q10 nor exercise by Q10 interaction effect on plasma triglyceride levels in rat.
NFθºB, IθºB, Nrf2 and HO-1levels in Q10 treated rats after exercise trainingChanges of the levels of NFθºB, IθºB, Nrf2 and HO-1 of heart, liver and muscle tissues after exercise training in Q10 treated rats are shown in the Figure 1, Figure 2, Figure 3 and Figure 4. Exercised rats had greater IθºB (p < 0.001), Nrf2 (p < 0.001) and HO-1 (p < 0.01) levels in heart than sedentary rats (Figure 1). However, exercised rats had less the level of NFθºB (p < 0.05) in heart than sedentary rats. Overall, there were52.4%, 54.8% and 20.6% increases in IθºB, Nrf2 and HO-1 of heart was 12.3% a decrease in NFθºB of heart. Q10 treated rats had greater IθºB (p < 0.001), Nrf2 (p < 0.001) and HO-1 (p < 0.001) levels in heart than untreated rats. However, Q10 treated had lower levels of NFθºB (p < 0.001) in heart than untreated rats. Namely, there were increases in levels of heart IθºB, Nrf2 and HO-1 by 69.2%, 63.8% and 65.8%, respectively and decreases in heart NFθºB level by 36.4% in Q10 treated rats (p < 0.001 for all). There was no exercise by Q10 supplementation interaction effect on levels of NFθºB, IθºB, Nrf2 and HO-1 in heart tissue (p > 0.05). Exercised rats had greater the levels of hepatic IθºB (p < 0.001), Nrf2 (p < 0.001) and HO-1 (p < 0.001) than sedentary rats (Figure 2). However, exercised rats had less the level of NFθºB (p < 0.05) in liver than sedentary rats. Overall, there were 24.2%, 33.5% and 40.2% increases in hepatic IθºB, Nrf2 and HO-1 in response to exercise, although there was 9.5% a decrease in NFθºB of liver. Q10 treated rats had greater hepatic IθºB (p < 0.001), Nrf2 (p < 0.001) and HO-1 (p < 0.001) levels than untreated rats. Nevertheless, Q10 treated rats had less the level of NFθºB (p < 0.01) in liver than untreated rats. Namely, there were increases in levels of hepatic IθºB, Nrf2 and HO-1 by 63.9%, 105.5% and 85.5%, respectively (p < 0.001) and decreases in levels of hepatic NFθºB by 18.7% in Q10 treated rats (p < 0.01). There was no interaction by exercise and Q10 supplementation on the levels of NFθºB and IθºB in liver tissue (p > 0.05), but there was interaction between exercise and Q10 supplementation on the Nrf2 level (p < 0.01) and HO-1 (p < 0.05) in liver tissue. The hepatic Nrf2 and HO-1 levels increased more exercised rat than sedentary rat when supplemental Q10. Exercised rats had greater IθºB (p < 0.01), Nrf2 (p < 0.001) and HO-1 (p < 0.001) levels in muscle than sedentary rats (Figure 3). However, exercised rats had less the level of NFθºB (p < 0.001) in muscle than sedentary rats. There were 22.3%, 25.8% and 41.2% increases in IθºB, Nrf2 and HO-1 levels of muscle in response to exercise and there was 23.0% a decrease in NFθºB of muscle of in exercised rats. Q10 treated rats had greater IθºB (p < 0.001), Nrf2 (p < 0.001) and HO-1 (p < 0.001) levels in muscle than untreated rats. Nevertheless, Q10 treated had less the NFθºB level (p < 0.001) in muscle than untreated rats. There were increases in levels of muscle IθºB, Nrf2 and HO-1 by 73.9%, 52.3% and 53.6%, respectively and decreases in muscle NFθºB level by 27.9% in Q10 treated rats (p < 0.001 for all). Except for IθºB level, all protein levels in muscle tissue altered by exercise by Q10 supplementation interaction effect (p > 0.05). There were 43.8% decreases in exercise and Q10 treatment groups NFθºB levels whereas, 90.3% and 114.1 increases in Nrf2 and HO-1 levels compared to sedentary untreated rats in muscle tissue.
This study reports the effect of Q10 on the levels of biochemical parameters in serum and levels of NFθºB, IθºB, Nrf2 and HO-1 of the heart, liver and slow-twitch muscles (soleus and gastronemius deep portion) in rats after exercise training. There were no significant change plasmalevels of urea, creatinine or glucose in any of the groups; exercise, however, caused a significant decrease in triglyceride, which was further reduced in Q10 supplemented rats. These data suggest that Q10 does not directly interfere in protein, carbohydrate or lipid metabolism. An increase in energy expenditure caused by exercise training and possible metabolic alterations might be the major cause behind the improvements in the triglycerides profiles and a better aerobic capacity with enhanced utilization of lipids. Additionally, Alleva et al. (1995) reported that coenzyme Q10 is an intracellular antioxidant that protects the membrane phospholipids and mitochondrial membrane protein. Moreover, Q10 supplementation significantly affected the protein levels of NFθºB, IθºB, Nrf2 and HO-1 in exercise group when compared with the sedentary-untreated groups. The protein level of NFθºB was considerably low in all three tissues analyzed in exercised and Q10 treated rats compared with sedentary untreated rats. The expression of IθºB, which is an inhibitory protein that keeps NFθºB in an inactive state, however, increased significantly in these tissues. Earlier, Q10 has been reported to decrease oxidative stress by acting as an antioxidant and free-radical scavenger (Zhang et al., 2013) and hence it can suppress the level of NFθºB. Exercise enhances the redox-regulation involving NFθºB and Nrf2 which contribute to control oxidative stress (George et al., 2008). It has previously been reported that exercise training induces expression of antioxidant enzymes, HO-1 and superoxide dismutase and increased NFθºB-DNA binding activity in rat tissues (George et al., 2008). NFθºB is a transcription factor which plays a critical role in inflammatory responses, cellular growth, and apoptosis (Ali and Mann, 2004). The regulatory impact of Q10 on basic molecular mechanism is controversial: in an earlier study NFθºB has been shown to be up regulated, while the mitochondrial complex-1 down regulated in response to Q10 supplementation (Ebadi et al., 2004). It would be pertinent to mention that in the inner mitochondrial membrane, Q10 functions as an electron carrier from enzyme complex I and complex II to complex III. Nevertheless, in this study, the levels of NFθºB in the heart, liver and muscle decreased after exercise training and Q10 supplementation; levels of NFθºB, however, were lower in exercised and exercised supplemented groups when compared with the sedentary untreated group. There was a significant increase in IθºB, the small inhibitory protein that engages NFθºB in exercised and exercised+supplemented groups when compared with the respective the sedentary untreated group, indicating a decrease in the level of NFθºB. The increase was the highest in the exercise group supplemented with Q10. A similar pattern was observed with the levels of Nrf2 and HO-1 in the heart, liver and muscle. The levels of Nrf2 and HO-1 increased significantly in all groups over sedentary untreated group, but the increase was the highest in Q10 supplemented exercise group. The Nrf2 is a transcription factor that binds to the antioxidant response element (ARE), thus increasing the transcription of a variety of cytoprotective genes. It is released from its sequestering protein Keap1 by distinct mechanisms. In the absence of Keap1, Nrf2 translocates into the nucleus, binds to the ARE and drive the expression of Nrf2 target genes which includes HO-1 and other genes such as NAD(P)H quinone oxidoreductase 1, glutamate-cysteine ligase, and glutathione S transferases (Kansanen et al., 2013). This study demonstrates an increase in Nrf2 with a concomitant increase in HO-1 in all groups, including the Q10 sedentary and exercise groups. Inducible HO-1 is an antioxidant stress protein, mainly induced by ROS, cytokines and hyperthermia, which has been reported to increase in exercise (Paine et al., 2010; Park and Kim, 2014). Consistent with our and others results exercise training stimulates transcription factors (Nrf2 and NFθºB), decreases oxidative stress and increases the antioxidant defenses (George et al., 2008). Nevertheless, the regulatory pathways leading to an increased expression of HO-1 after exercise has not fully been understood, but a causal involvement of a cytokine-mediated generation of ROS has been proposed (Niess et al., 1999). Wang et al. (2015) showed that antioxidant supplementation exhibits protection from alcoholic myopathy due to oxidative stress by regulation of HO-1 and Nrf2 pathways. Oxidative stress contributes to cellular dysfunction (Noh et al., 2013), although the biological basis of the precise functional role of mitochondria in this dysfunction is not completely understood. Q10, which is an essential cofactor of the electron transport chain and a potent antioxidant, might protect the cell against oxidative stress by scavenging ROS. In our study supplementation with Q10 further increased the level of HO-1. Consistent with our results, in a previous study, in H4IIE hepatoma cells, Q10 inhibited DMN-induced liver fibrosis through Nrf2/ARE activation, while protective effects of Q10 was abolished in Nrf2-null MEF cells (Choi et al. 2009). The authors postulated that Q10-mediated Nrf2 activation may result from the sustained auto-oxidation of the quinone moiety of Q10 (Choi et al. 2009). Therefore, this study provides further evidence to support that exercise training caused an increase in Nrf2, which was more evident in Q10 supplemented rats. Modulation of Nrf2 by exercise and Q10 supplementation points out the potential therapeutic role of Q10 in metabolic stress, when the requirement for energy is increased.
Taken together, this study implies that exercise training provides beneficial adaptations on the activity of NFθºB, IθºB, Nrf2 and HO-1, which is further enhanced by Q10 supplementation.
ACKNOWLEDGEMENTS |
Authors thank Firat University Scientific Research Projects Unit (FUBAP) for supporting this study (BSY.12.02). This work was also supported in part by the Turkish Academy of Sciences (KS). This paper featuring eight authors: RP, CO, MT, NS, SA, VC, MA and KS. The study was designed by KS and MA; data were collected and analyzed by RP, MT, CO, VC and NS; data interpretation and manuscript preparation were undertaken by KS, SA, CO and MA. All authors approved the final version of the paper. None of the authors had a conflict of interest. |
|
AUTHOR BIOGRAPHY |
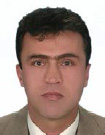 |
Ragip Pala |
Employment: Assist. Prof., Firat University, Physical Education and Sport Faculty, Turkey |
Degree: PhD |
Research interests: Sports Physiology, Exercise |
E-mail: rpala@firat.edu.tr |
|
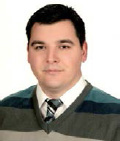 |
Cemal Orhan |
Employment: Assoc. Prof., Firat Univ. Veterinary Faculty, Depart. of Nutrition, Turkey |
Degree: PhD |
Research interests: Chronic diseases, phytochemicals |
E-mail: corhan@firat.edu.tr |
|
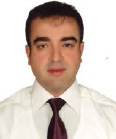 |
Mehmet Tuzcu |
Employment: Assist. Prof., Firat Univ. Science Faculty, Department of Biology, Turkey |
Degree: PhD |
Research interests: Chronic diseases, molecular mechanism of phytochemicals |
E-mail: mtuzcu@firat.edu.tr |
|
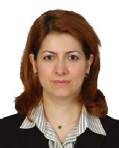 |
Nurhan Sahin |
Employment: Prof., Firat University Veterinary Faculty, Department of Nutrition, Turkey |
Degree: PhD |
Research interests: Nutrition, Chronic diseases, phytochemicals, vitamins, minerals |
E-mail: nsahin@firat.edu.tr |
|
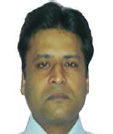 |
Shakir Ali |
Employment: Prof. Jamia Hamdard Univ., Faculty of Science, Depart. of Biochemistry, India |
Degree: PhD |
Research interests: Chronic Inflammatory Disorders, Biochemical Mechanisms and Therapeutic Insights, Bioinformatics |
E-mail: sali@jamiahamdard.ac.in |
|
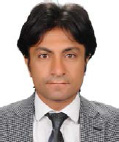 |
Vedat Cinar |
Employment: Prof., Firat University, Physical Education and Sport Faculty, Turkey |
Degree: PhD |
Research interests: Sports Physiology, Exercise |
E-mail: vcinar@firat.edu.tr |
|
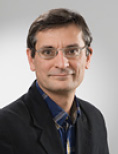 |
Mustafa Atalay |
Employment: Adjunct Prof., Senior Lecturer, Institute of Biomedicine, Physiology, University of Eastern Finland, Kuopio, Finland. |
Degree: MD, MPH, PhD |
Research interests: Redox regulation and molecular mechanisms of training responses. |
E-mail: mustafa.atalay@uef.fi |
|
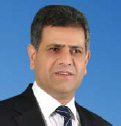 |
Kazim Sahin |
Employment: Prof., Firat University Veterinary Faculty, Department of Nutrition, Turkey |
Degree: PhD |
Research interests: Nutrition, on vitamin, mineral, and phytochemical metabolism, cancer prevention, stress, exercise and obesity |
E-mail: nsahinkm@yahoo.com |
|
|
|
REFERENCES |
 Ali S., Mann D.A. (2004) Signal transduction via the NFθºB pathway: a targeted treatment modality for infection, inflammation and repair. Cell Biochemistry and Function 22, 67-79.
|
 Alleva R., Tomasetti M., Battino M., Curatola G., Littarru G.P., Folkers K. (1995) The roles of coenzyme Q10 and vitamin E on the peroxidation of human low density lipoprotein subfractions. Proceedings of the National Academy of Sciences of the United States of America 26, 9388-9391.
|
 Atalay M., Lappalainen J., Sen C.K. (2006) Dietary antioxidants for the athlete. Current Sports Medicine Reports 5, 182-186.
|
 Bentinger M., Tekle M., Dallner G. (2010) Coenzyme Q biosynthesis and functions. Biochemical and Biophysical Research Communications 396, 74-79.
|
 Choi H.K., Pokharel Y.R., Lim S.C., Han H.K., Ryu C.S., Kim S.K., Kwak M.K., Kang K.W. (2009) Inhibition of liver fibrosis by solubilized coenzyme Q10: Role of Nrf2 activation in inhibiting transforming growth factor-beta1 expression. Toxicology and Applied Pharmacology 240, 377-384.
|
 Ebadi M., Sharma S.K., Wanpen S., Amornpan A. (2004) Coenzyme Q10 inhibits mitochondrial complex-1 down-regulation and nuclear factor-kappa B activation. Journal of Cellular and Molecular Medicine 8, 213-222.
|
 Garrido-Maraver J., Cordero M.D., Oropesa-Ávila M., Fernández Vega A., de la Mata M., Delgado Pavón A., de Miguel M., Pérez Calero C., Villanueva Paz M., Cotán D., Sánchez-Alcázar J.A. (2014) Coenzyme q10 therapy. Molecular Syndromology 5, 187-197.
|
 George L, Asghar M., Lokhandwala M.F. (2008) Exercise Stimulates Transcription Factors (Nrf2 & NFθºB), Increases Antioxidant Defenses, Decreases Oxidative Stress, and Restores Renal Dopamine D1 Receptor Function in Aging. The FASEB Journal 22, 1159.6-.
|
 IBM SPSS. (2012) IBM SPSS Statistics for Windows, Version 21.0. Armonk, NY. IBM Corp.
|
 Kansanen E., Kuosmanen S.M., Leinonen H., Levonen A.L. (2013) The Keap1-Nrf2 pathway: Mechanisms of activation and dysregulation in cancer. Redox Biology 1, 45-49.
|
 Kon M., Kimura F., Akimoto T., Tanabe K., Murase Y., Ikemune S., Kono I. (2007) Effect of Coenzyme Q10 supplementation on exercise-induced muscular injury of rats. Exercise Immunology Review 13, 76-88.
|
 Kumar K.J., Yang H.L., Tsai Y.C., Hung P.C., Chang S.H., Lo H.W., Shen P.C., Chen S.C., Wang H.M., Wang S.Y., Chou C.W., Hseu Y.C. (2013) Lucidone protects human skin keratinocytes against free radical-induced oxidative damage and inflammation through the up-regulation of HO-1/Nrf2 antioxidant genes and down-regulation of NF-θºB signaling pathway. Food and Chemical Toxicolog 59, 55-66.
|
 Lee S., Park Y., Zuidema M.Y., Hannink M., Zhang C. (2011) Effects of interventions on oxidative stress and inflammation of cardiovascular diseases. World Journal of Cardiology 3, 18-24.
|
 Liu Y.F., Chen H.I., Yu L., Kuo Y.M., Wu F.S., Chuang J.I., Liao P.C., Jen C.J. (2008) Upregulation of hippocampal TrkB and synaptotagmin is involved in treadmill exercise-enhanced aversive memory in mice. Neurobiology of Learning and Memory 90, 81-89.
|
 Mellors A., Tappel A. (1966) Quinones and quinols as inhibitors of lipid peroxidation. Lipids 1, 282-284.
|
 Niess A.M., Passek F., Lorenz I., Schneider E.M., Dickhutha H-H, Northoff H., Fehrenbach E. (1999) Expression of the antioxidant stress protein heme oxygenase-1 (HO-1) in human leukocytes. Free Radical Biology and Medicine 26, 184-192.
|
 Noh Y.H., Kim K.Y., Shim M.S., Choi S.H., Choi S., Ellisman M.H., Weinreb R.N., Perkins G.A., Ju W.K. (2013) Inhibition of oxidative stress by coenzyme Q10 increases mitochondrial mass and improves bioenergetic function in optic nerve head astrocytes. Cell Death and Disease 4, e820-.
|
 Paine A., Eiz-Vesper B., Blasczyk R., Immenschuh S. (2010) Signaling to heme oxygenase-1 and its anti-inflammatory therapeutic potential. Biochemical Pharmacology 80, 1895-1903.
|
 Park J.S., Kim H.S. (2014) Regulation of hemeoxygenase-1 gene expression by Nrf2 and c-Jun in tertiary butylhydroquinone-stimulated rat primary astrocytes. Biochemical and Biophysical Research Communications 447, 672-677.
|
 Sahin K., Orhan C., Tuzcu M., Borawska M.H., Jabłonski J., Guler O., Sahin N., Hayirli A. (2013) Berberis vulgaris root extract alleviates the adverse effects of heat stress via modulating hepatic nuclear transcription factors in quails. The British Journal of Nutrition 110, 609-616.
|
 Sarter B. (2002) Coenzyme Q10 and cardiovascular disease: a review. The Journal of Cardiovascular Nursing 16, 9-20.
|
 Schaefer J., Navas P., Horvath Z., Jackson S. (2009) Myopathic coenzyme Q10 deficiency. Acta Myologica 28, 41-.
|
 Wang J., Zhang L., Zhang Y., Luo M., Wu Q., Yu L., Chu H. (2014) Transcriptional upregulation centra of HO-1 by EGB via the MAPKs/Nrf2 pathway in mouse C2C12 myoblasts. Toxicology in vitro 29, 380-388.
|
 Zhang Y.P., Song C.Y., Yuan Y., Eber A., Rodriguez Y., Levitt R.C., Takacs P., Yang Z., Goldberg R., Candiotti K.A. (2013) Diabetic neuropathic pain development in type 2 diabetic mouse model and the prophylactic and therapeutic effects of coenzyme Q10. Neurobiology of Disease 58, 169-178.
|
|
|
|
|
|
|