|
|
|
ABSTRACT |
The integration of balance and plyometric training has been shown to provide significant improvements in sprint, jump, agility, and other performance measures in young athletes. It is not known if a specific within session balance and plyometric exercise sequence provides more effective training adaptations. The objective of the present study was to investigate the effects of using a sequence of alternating pairs of exercises versus a block (series) of all balance exercises followed by a block of plyometric exercises on components of physical fitness such as muscle strength, power, speed, agility, and balance. Twenty-six male adolescent soccer players (13.9 ± 0.3 years) participated in an 8-week training program that either alternated individual balance (e.g., exercises on unstable surfaces) and plyometric (e.g., jumps, hops, rebounds) exercises or performed a block of balance exercises prior to a block of plyometric exercises within each training session. Pre- and post-training measures included proxies of strength, power, agility, sprint, and balance such as countermovement jumps, isometric back and knee extension strength, standing long jump, 10 and 30-m sprints, agility, standing stork, and Y-balance tests. Both groups exhibited significant, generally large magnitude (effect sizes) training improvements for all measures with mean performance increases of approximately >30%. There were no significant differences between the training groups over time. The results demonstrate the effectiveness of combining balance and plyometric exercises within a training session on components of physical fitness with young adolescents. The improved performance outcomes were not significantly influenced by the within session exercise sequence. |
Key words:
Power, strength, jumps, sprints, balance, children
|
Key
Points
- The combination of balance and plyometric exercises can induce significant and substantial training improvements in muscle strength, power, speed, agility, and balance with adolescent youth athletes
- The within training session sequence of balance and plyometric exercises does not substantially affect these training improvements.
|
In a recently published narrative review, Faigenbaum et al. (2009) emphasized the benefits of resistance training for young athletes to stimulate their athletic development, to tolerate the demands of long-term training and competition, and to induce long-term health promoting effects that are robust over time and track into adulthood. In a recent scoping review, Granacher et al. (2016) introduced a conceptual model for the implementation of resistance training during the stages of long-term athlete development. The authors recommended applying balance and plyometric training during the stages ‘learning to train’, ‘training to train’, and ‘training to compete’. It has previously been purported that balance is a fundamental motor skill for the development of athletic performance (Behm and Colado Sanchez, 2013). Taube et al. (2007) observed significant balance improvements following six weeks of balance training with three training sessions per week in male elite athletes (i.e., ski jumpers and Nordic combined skiers with a mean age of 14 years). Despite the fact that the balance training protocol did not contain any strength-building or plyometric exercises, there was still an increase in squat jump and countermovement jump (CMJ) performance after training. Other studies using adult participants have also reported improved jump height performance following balance training with no additional resistance training (Bruhn, 2001; Kean et al., 2006). The positive training effects of balance on jump height are presumably related to the strong correlations between balance measures and power (r = 0.511-0.827) in youth (Hammami et al., 2016a). There is evidence in the literature that plyometric training is well-suited to enhance muscular power (de Villarreal et al., 2009) and/or proxies of muscular power because plyometrics (e.g., hops, jumps) refer to exercises that link strength with speed of movement (Faigenbaum and Chu, 2001). A position stand / review by the Canadian Society for Exercise Physiology (CSEP) recommended that resistance training exercises for youth can range in complexity from simple body mass, dumbbell, or machine-type resistance exercises to more advanced techniques such as plyometrics and Olympic-style lifting (Behm et al., 2008). Consequently, a large number of studies have examined the effectiveness of plyometric training on muscle power and athletic performance particularly with youth soccer athletes (Chaouachi et al., 2014a; 2014b; Granacher et al. 2015; Meylan and Malatesta, 2009; Ramirez-Campillo et al., 2014). Chaouachi et al. (2014a) trained younger children (10-12 years) with plyometrics and traditional resistance training for 12 weeks. Using precision estimates for inferences, they found that plyometric training was 78% likely to elicit substantially better training adaptations than traditional resistance training for balance, isokinetic force, and power, as well as 5- and 20-m sprints. In a recently published systematic review and meta-analysis, Lesinski et al. (2016) demonstrated that plyometric training is effective in improving muscular strength, vertical jump height, linear sprint performance, agility, and sport-specific performance in the range of small-to-medium effect sizes in youth athletes. As both balance and plyometric training are effective training modalities for youth, it is possible that a program integrating both these physical training components may lead to an enhanced performance adaptation. It has previously been reported that balance and plyometric training can be implemented in the training routine of youth athletes as separate training regimens or in different combinations. Chaouachi et al. (2014b) demonstrated that an eight week training program with 12-15 year old boys that combined plyometric and balance exercises was as or more effective (sprint, shuttle run, leg stiffness) than a plyometric only training program, even though the combined program had less than half of the volume of plyometric exercises compared to the plyometric only program. Hammami et al. (2016b) examined the sequencing effects of balance and plyometric training on measures of physical fitness in youth soccer athletes aged 12-13 years. The authors were able to demonstrate that the blocked combination of four weeks of balance training with subsequent four weeks of plyometric training produced similar (medicine ball throw, horizontal and vertical jumps, agility and sprints) or superior (reactive strength, leg stiffness, triple hop, balance) performance enhancements as compared to the blocked combination of four weeks of plyometric training prior to four weeks of balance training. Besides the blocked / sequenced combination of balance and plyometric training, these two training regimens can also be integrated as same-session combined training. This training format using balance and plyometric exercises has not been previously investigated. In other words, balance training could be conducted during the first part of the training session and plyometric training during the second or balance and plyometric exercises could be implemented in pairs (i.e., balance exercise always precedes a plyometric exercise). However, as plyometric training can be an intense, fatiguing activity (Wadden et al., 2012); pairing balance and plyometric exercises could have fatigue-related deleterious effects upon the quality of the later training exercises (Romero-Franco and Jimenez-Reyes, 2015). Conversely, prior non-fatiguing, high intensity exercises such as plyometrics could also have potentiating effects on the subsequent training activity (Sale, 2002). Therefore, the purpose of this study was to examine the effects of eight weeks of balance and plyometric training when either conducted in the form of blocked training sessions or when applied as alternating exercise pairs on measures of physical fitness in youth soccer athletes. It was hypothesized that the blocked form (BBPT: block of all balance exercises before plyometric training exercises) would result in larger performance enhancements because blocked balance exercises are conducted in a rested state while the alternated form (ABPT: a series of two paired exercises, which alternate balance exercises before plyometric training exercises) may induce fatigue due to the combination of a balance with a plyometric exercise.
ParticipantsYoung male elite-level soccer players, between 13-14 years of age, and members of a first division Tunisian soccer club (Esperance Club Tunis, Tunisia), volunteered to participate in this study and were either enrolled in ABPT (n=13) or BBPT (n = 13) training program. With reference to the study of Hamammi et al. (2016b) an a priori power analysis (Faul et al., 2007) with an assumed Type I error of 0.01 and a type II error rate of 0.10 (90% statistical power) was conducted for results in the Y balance test as a proxy of dynamic balance and revealed that 26 persons would be sufficient to observe a medium group × test interaction effect. Participant characteristics are listed in Table 1. All participants were from similar socio-economic status and had the same daily school and soccer team-training schedules. All participants trained four times a week with a match played during the weekend over the entire intervention period (8 weeks). Since they lived in the same city, environmental conditions for testing and training were similar for all individuals. Participants were not involved in any after-school activities or any formalized strength and conditioning training programs other than their formal soccer training. To estimate the maturity status of participants, a maturity index (i.e., timing of maturation) was calculated (Table 1) (Mirwald et al., 2002). This assessment is a non-invasive and practical method of predicting years from peak height velocity (PHV) as a measure of maturity offset using height and age as variables (PHV = 7.999994 + [0.0036124 × age × height]). The equation has previously been validated with standard error of estimates reported as 0.57 and 0.59 years, respectively (Mirwald et al., 2002). Before participation in this study, the participants were given a letter that included written information about the study and a request for consent from the parents or their legal representatives to allow their children to participate in the study. Parents or legal representatives and participants provided informed consent after thorough explanation of the objectives and scope of this project, the procedures, risks, and benefits of the study. The study was conducted according to the Declaration of Helsinki and the protocol was fully approved by the Ethics Committee of the National Centre of Medicine and Science of Sports of Tunis (CNMSS) before the commencement of the assessments. No player had any history of musculoskeletal, neurological, or orthopedic disorder that might impair their ability to execute plyometric or balance training or to perform power or balance tests.
Experimental designYoung male adolescents participated in an 8-week training program with two training sessions per week. Participants were randomly allocated to a program that either alternated a series of paired individual balance and plyometric exercises (n = 13, ABPT: Table 2a) or they performed a block of balance exercises prior to a block of plyometric exercises (n = 13, BBPT: Table 2b) within each training session. Similar to other previously published training studies (Granacher et al. 2015, Hammami et al. 2016a;2016b), a true control group could not be incorporated as the two experimental groups were national level elite athletes and there were no comparable athletes available that would provide similar baseline values. Pre- mid- and post-training measures included proxies of strength, power, agility, sprint, and balance such as CMJ, reactive strength index (RSI), maximum voluntary isometric contraction (MVIC) back and knee extension strength, standing long jump (SLJ), 10-30 m sprints, agility, standing stork, and Y-balance tests as well as relative leg stiffness. As other previous studies have demonstrated the effectiveness of balance prior to a plyometric training sequence in youth (Granacher et al. 2015, Hamami et al. 2016), the objective of the present study was to compare the effect of combined balance and plyometric training when either conducted in the form of blocked training sessions or when applied as alternating exercise pairs in two comparable groups of athletes.
ProceduresAll procedures were carried out during the second half of the competitive season (March-May 2016). Before the commencement of the study and prior to the initiation of testing, all players completed a two-week orientation period (three sessions/week) to become familiar with the general environment, form and technique of each fitness test used to evaluate sprint, strength, power, agility, and balance technique for each training exercise, equipment, and the experimental procedures. During this time, the players received consistent instructions from Certified Strength and Conditioning Specialists on proper technique for the balance exercises, plyometric exercises and landing. Each subject’s data pertaining to age, height, sitting height, body mass, and body fat were collected. Height and body mass were collected using a wall-mounted stadiometer and electronic scale, respectively. Body mass index was calculated as mass per height squared (kgpm-2). The sum of skinfolds (biceps, triceps, subscapular, and suprailiac) was monitored with Harpenden skinfold calipers (Baty International,West Sussex, England). Body fat measurements were conducted according to Deurenberg et al. (1990). All anthropometric measurements were taken at pre- and post-training by the same experienced sport scientist. Performance testing occurred before (pre), after 4-weeks of training (mid) and following the 8-week training period (post). Prior to testing, participants completed a warm-up that included a 5-min jog at a self-selected comfortable pace followed by a 5-min series of dynamic stretching (i.e. hip flexion/extension, hip abduction/adduction, butt kicks). The testing protocol included assessment of lower body strength and power (isometric back and knee extension force, horizontal and vertical jumps, reactive strength, and leg stiffness), agility (4 × 9 m shuttle run), speed acceleration (from a stationary position: 10-m sprint), maximal speed (30-m sprint), and static (Standing Stork Test) and dynamic balance (Y-Balance Test). Testing was conducted pre-, mid- and post-training at the National team club: Esperance Club Tunis, Tunisia.
Physical fitness testsMuscular power: CMJ test was performed using an Ergo jump system (Ergojump apparatus; Globus Italia, Codogne, Italy) according to the procedure described by Chaouachi et al. (2014a). During CMJ, participants were instructed to place their hands on the hips to minimize lateral and horizontal displacement during performance, to prevent any influence of arm movements on the vertical jumps, and to avoid coordination as a confounding variable in the assessment of the leg extensors’ neuromuscular performance. Participants also had to leave the ground with the knees and ankles extended and land in the same position and location to minimize horizontal displacement and influence on flight time. The CMJ involved the participants lowering themselves as quickly as possible from an upright standing position to a self-selected depth, followed immediately by a vertical jump. Participants were instructed to “jump as high as possible”. Three trials were performed with approximately 2 min. recovery and the best result was used for analysis. For the standing long jump (SLJ) test, participants stood stationary with the toes aligned level with the start line and were instructed to push off vigorously and jump forward as far as possible. Participants were allowed the use of a countermovement with arms and body swing. The distance jumped from the start line at takeoff to the position of the heel upon landing was measured in centimeters using a metal tape measure. Participants completed three trials, with the highest score being recorded. With the Triple Hop Test (THT), the tape measure was fixed to the ground, perpendicular to a starting line. Participants were instructed to stand behind the starting line with their non-dominant leg forward and the dominant leg off the ground. The leg used to kick a soccer ball was identified as the dominant leg. The subject performed three consecutive maximal hops forward on the same leg to reach the maximal horizontal distance. Arm swing was allowed. The investigator measured the distance hopped from the starting line to the point where the heel hit on the completion of the third and final hop. Relative leg stiffness (kiloNewtons/meter•kg) was calculated from the average contact time and flight time together with body mass (Dalleau et al., 2004). Jump height and ground contact time were averaged across the hops and used to calculate the reactive strength index as follows: RSI = Jump height (m)/ground contact time (s). Both tests were repeated three times, and the maximum distance achieved during the three trials was recorded in centimeters and was used for analysis. Reactive strength index and leg stiffness was measured respectively during maximal and sub-maximal hopping as previously reported (Chaouachi et al., 2014b; Dalleau et al., 2004; Hammami et al., 2016b). The maximal hopping protocol involved participants performing five repeated bilateral maximal vertical hops in place on the contact mat. Participants were instructed to maximize jump height and minimize ground contact time. The first jump in each trial was discounted, whereas the remaining 4 hops were averaged for analysis of reactive strength index as follows: RSI = Jump height (m)/ground contact time (s) (Flanagan and Comyns, 2008). Relative leg stiffness was measured during submaximal hopping by modeling the vertical ground reaction force, based on the flight and contact time during hopping. Participants were asked to hop bilaterally for 20 consecutive hops at 2.0 Hz. An electronic metronome helped the subjects to maintain the required frequencies by means of an auditory signal. The initial five and last five hops were discarded and the subsequent 10 hops averaged to provide a measure of leg stiffness. Relative leg stiffness (kiloNewtons per meter•kg) was calculated from the average contact time and flight time across the 10 hops, together with body mass (Dalleau et al., 2004). Muscular strength: Maximal isometric knee extensor strength was measured, on the participant’s dominant leg using a calibrated hand-held dynamometer (Microfet 2, Hoggan Health Industries, Inc., Draper, UT). Specifics of the test position, stabilization, and dynamometer placement used in this study were chosen according to the instrument manual instructions as previously described (Rouissi et al., 2016). The dynamometer incorporates a load cell and has a digital display. The hand-held dynamometer was placed perpendicular to the anterior aspect of the tibia, 5 cm proximal of the medial malleolus. Participants were seated at the edge of an examination table and positioned so that both feet were off the ground, hips and knees both flexed at 90°. Participants were instructed to fold their arms across their chest to avoid hand support and maximally extend their knee against the tester’s resistance. The participants exerted a maximal isometric muscle contraction lasting 3-5 seconds while the dynamometer was held stationary. This was repeated two times with a 30-s rest period and the highest value was recorded for analysis. One adult examiner, with sufficient strength and body mass that ensured an isometric contraction by the youth, conducted all measures of hand-held dynamometry. High hand-held dynamometry reliability measurements in similar pediatric population in our laboratory have been reported elsewhere (Rouissi et al., 2016). MVIC back extensor strength was measured in kilograms using a back and leg dynamometer (Takei, Tokyo, Japan). Participants stood on the dynamometer foot stand with feet shoulder-width apart and gripped the handle bar positioned across the thigh. The chain length on the dynamometer was adjusted so that the legs were straight and the hips were flexed at a 30° angle to position the bar at the level of the patella. Participants were then asked to straighten their backs (i.e., stand upright) without bending their knees and lifted the dynamometer chain, with the pulling force applied on the handle, pulling upwards as strongly as possible. Participants completed three trials, the highest score being recorded as the measurement of maximal back strength under isometric conditions. A thirty-second rest interval was provided between each trial. Previous test retest reliability scores have been shown to be reliable in a similar athletic pediatric population (Hammami et al., 2016a). Speed: Acceleration and maximal running speed were evaluated using a stationary 10-m sprint, and 30-m maximal speed test. Stationary 10-m sprint involved sprinting 10-m as fast as possible from a stationary standing start position just behind the first timing gate. Start stance was consistent for each subject. Maximal speed 30-m sprint involved sprinting 30-m as fast as possible from a moving start. Players were located 20 cm behind the start line position and were instructed to run as quickly as possible along the 30-m distance. Time was automatically recorded using photocell gates (Brower Timing Systems, Salt Lake City, Utah, USA, accuracy of 0.01 s) placed 0.4-m above the ground. Participants performed 2 trials with at least 2 minutes of rest between trials. The run with the lowest 30-m time was selected for analysis. Previous test retest reliability scores have been shown to be reliable in a similar athletic pediatric population (Hammami et al., 2016a; 2016b). Agility: Agility was evaluated with the 4×9-m shuttle run test. With participants standing behind a starting line, they started the electronic clock by passing through the first timing gate. At the end of the 9-m section, participants were asked to step with one foot beyond a marker while reversing running direction and sprinting back to the start where the same reversal of movement direction was required. After the fourth 9-m section, the subject passed through the second timing gate to stop the electronic clock. The best time of two consecutive trials was recorded for the statistical analysis. At least 2 minutes of rest was provided between trials. Previous test retest reliability scores have been shown to be reliable in a similar athletic pediatric population (Hammami et al., 2016a; 2016b). Balance: Static balance was assessed utilizing the Stork stand balance protocol (Hammami et al., 2016a; 2016b). To perform the Stork stand test, participants stood with their opposite foot against the inside of the supporting knee and both hands on the hips. On the command, the subject raised the heel of their foot from the floor and attempted to maintain their balance as long as possible. The trial ended if the subject either moved his hands from his hips, the ball of the dominant foot moved from its original position, or if the heel touched the floor. This test was carried out on the dominant leg acting as the standing leg. The test was timed (s) using a stopwatch. The recorded score (duration in seconds) was the best of three attempts. Previous test - retest reliability scores for balance measures from our laboratory with a similar pediatric population have been high (Chaouachi et al., 2014b; Hammami et al., 2016a; 2016b). Dynamic balance was tested using the lower quarter Y balance test (YBT). Before the test started, participants’ length of the right leg was assessed in supine lying position by measuring the distance from the anterior superior iliac spine to the most distal aspect of the medial malleolus. Further, participants practiced 6 trials per reach direction to get familiarized with the testing procedures. All trials were conducted barefooted. The protocol used for the completion of the YBT is similar to that described previously (Hammami et al., 2016a; 2016b) and has been reported to possess high reliability in a similar pediatric population (Hammami et al., 2016a; 2016b). Participants stood on the dominant leg, with the most distal aspect of their great toe on the center of the footplate from the YBT Kit. The participants were then asked to push the reach-indicator block with the free limb in the anterior, posterior medial, and posterior lateral directions in relation to the stance foot on the central footplate, while maintaining their single-limb stance (Hammami et al., 2016a; 2016b). A test trial was classified invalid if the participants (1) did not touch the line with the reach foot while maintaining weight bearing on the stance leg, (2) lifted the stance foot from the footplate center, (3) lost balance at any point during the trial, (4) did not maintain start and return positions for one full second, or (5) touched down the reach foot to gain considerable support. The variables of interest for the study included the maximal reach for each direction. The average maximum normalized reach across the three directions was calculated in order to record a composite score for each subject. YBT measures were normalized by dividing each excursion distance by the participant's leg length, then multiplying by 100. Thus, normalized values can be viewed as a percentage of excursions distance in relation to the participant's leg length (Hammami et al., 2016a; 2016b). The test was demonstrated by a member of the research team prior to the participant completing three practice trials in each direction. Following the completion of the test trials, each participant was given a 1-minute rest period and then conducted two test trials in each direction. The examiner manually measured the distance from the centre of the grid to the touch point and the results were documented after each reach. A composite score (CS) was calculated and taken as the dependent variable using the following formula: CS = ([maximum anterior reach distance + maximum posteromedial reach distance + maximum posterolateral reach distance] / [leg length x 3]) x 100.
Training program interventionsThe 8-week training program with two training sessions per week consisted of either an APBT (Table 2a) or BBPT (Table 2b). At least 90 seconds rest was allowed after each set. Although the participants were involved with soccer-specific training (practices) four times a week in addition to a weekend match, none were involved in any other after-school activities or strength and conditioning training programs. Training for the study was always performed before soccer specific training, which consisted mainly of technical and tactical skill development. Throughout the experiment, the internal training load of all players was monitored using the session rate of perceived exertion (Session-RPE) method according to the procedures proposed by Impellizzeri et al. (2004) and expressed in arbitrary units (AU). About 30-minutes after training sessions, subjects were asked to rate the global intensity of the entire workout session using the category ratio-10 RPE scale with which all athletes were previously habituated. A daily training load was created by multiplying the training duration (in minutes) by the session RPE score. The weekly training load was determined by summing the daily training loads for each athlete during each week.
Statistical analysisData are presented as group mean values and standard deviations. After normal distribution was examined, an independent samples t-test was used to determine significant differences in pre-testing values between groups. The within session exercise sequence of balance and plyometric training on variables of physical fitness were analyzed in separate 2 (Group: ABPT, BBPT) × 3 (Time: pre, mid, post) ANOVAs with repeated measures on “Time”. When Group x Time interactions reached the level of significance, group-specific and Bonferroni corrected post-hoc tests were calculated to identify the comparisons that were statistically significant. Additionally, the classification of effect sizes was determined by converting partial eta-squared to Cohen’s d. For a two-group comparison, rendering an ANOVA with a F(1, df) statistic, where F = MSgroups / MSwithin, we have: eta2 = MSgroups / [MSgroups + df ’ MSwithin] and : d = 2’√(F / (df+2)). The effect size is a measure of the effectiveness of a treatment and it helps to determine whether a statistically significant difference is a difference of practical concern. According to Cohen (1988), effect sizes can be classified as small (0.00 ≤ d ≤ 0.49), medium (0.50 ≤ d ≤ 0.79), and large (d ≥ 0.80). The significance level was set at p < 0.05. Intraclass correlation coefficients were analyzed to assess the intrasession reliability of the researchers and participants (2 trials performed for each measure at pre-test) (Shrout and Fleiss, 1979). All analyses were performed using Statistical Package for Social Sciences (SPSS) version 23.0.
All participants received treatment conditions as allocated. Twenty-six pre- or PHV soccer players completed the training program (Table 2a and Table 2b). Participants attended all training sessions and none reported any training- or test-related injury. Internal training load was not significantly different between the two experimental groups across the training period. Weekly session-RPE was 1019.51, and 1009.78 arbitrary units for the ABPT and BBPT respectively (p R 0.05). Table 3 illustrates the excellent intraclass correlation coefficients of all measures. Table 4 describes mean and percentage changes with pre-, mid-, and post-intervention results for all outcome variables. Within subject effect sizes for Groups APBT and BBPT are presented in Table 5. No statistically significant between-group differences were found in all anthropometric and physical fitness pre-training values.
Muscular powerThe statistical analysis indicated significant main effects of “Time” for CMJ height (F2, 96 = 69.31, p < 0.001), SLJ (F2, 96 = 65.34, p < 0.001), THT (F2, 96 = 27.54, p < 0.001), RSI (F2, 96 = 74.62, p < 0.001), and relative leg stiffness during hopping (F2, 96 = 33.77, p < 0.001) (Table 4). However, we could not detect any significant main effects of “Group” and no significant “Group x Time” interactions for measures of muscular power.
Muscular strengthFor measures of muscular strength, significant main effects of “Time” were observed for back (F2, 96 = 17.89, p < 0.001), and KE MVIC tests (F2, 96 = 119.10, p < 0.001) (Table 4). A significant “Group x Time” interaction was found for the KE MVIC test (F2, 96 = 4.04, p < 0.05). Post-hoc analyses indicated significant improvements from pre- to mid- (p = 0.004), pre- to post- (p < 0.001), and mid- to post-training (p < 0.001) with group APBT. Group BBPT showed performance enhancements from pre- to mid- (p < 0.001), pre- to post- (p < 0.001), and mid- to post-training (p = 0.006).
SpeedWith regards to the 10-m and 30-m sprint tests, the analyses showed significant main effects of “Time” (10-m: F2, 96 = 41.50, p < 0.001, 30-m: F2, 96 = 30.43, p < 0.001). Significant “Group x Time” interactions were found for both parameters (10-m: F2, 96 = 4.75, p = 0.027, 30-m: F2, 96 = 4.48, p = 0.037)(Table 4). Post-hoc analyses indicated significant improvements in 10-m sprint time from pre- to post- (p < 0.001), and mid- to post-training (p < 0.001) with group APBT. Group ABPT additionally achieved significant improvements in 30-m sprint time from pre- to post- (p = 0.013) and from mid- to post-training (p < 0.001). Group BBPT significantly improved their 10-m sprint time from pre- to mid- (p = 0.009), pre- to post- (p < 0.001), and mid- to post-training (p = 0.008). Further, performance enhancements for 30-m sprint time were detected from pre- to mid- (p = 0.006) and pre- to post-training (p < 0.001) with group BBPT.
AgilityThe statistical analysis indicated significant main effects of “Time” for the 4 x 9-m agility test (F2, 96 = 188.90, p < 0.001) but no significant main effect of “Group” and no significant “Group x Time” interaction (Table 4).
BalanceSignificant main effects of “Time” but not of “Group” were observed for the Standing Stork test (F2, 96 = 19.94, p < 0.001) and the Y-balance test (F2, 96 = 35.92, p < 0.001) (Table 4). The analysis indicated a significant “Group x Time” interaction for the Y-balance test (F2, 96 = 4.05, p = 0.024). For group ABPT, significant pre-mid- (p < 0.001), pre-post- (p < 0.001), and mid-post-training changes (p = 0.042) were found for the Y-balance test. For group BBPT, significant pre-post-training changes were found only (p = 0.024).
The most important findings in the present study were that an eight-week training program combining balance and plyometric exercise training with male adolescent soccer athletes with a mean age of 14 years provided significant and generally large magnitude improvements with a variety of strength, power, agility, sprint, and balance tests. Secondly, the particular sequencing of balance and plyometric exercises within the training sessions (alternating vs. block of exercises) did not demonstrate any significant training advantages. Specifically, alternating pairs of balance and plyometric exercises (ABPT: perform a balance exercise followed by a plyometric exercise) induced 10 large, 1 medium and 1 small magnitude (overall 32.6%↑) increases in performance measures compared to 10 large and 2 medium magnitude (overall 31.3%↑) improvements when using a block exercise scheme (BBPT: perform all balance exercises first followed by all plyometric exercises)(Table 5). Hence, either training regimen was effective for improving strength, power, agility, sprint, and balance with pre-PHV and PHV soccer athletes. The myth that resistance training could be harmful for the development of children has been refuted over the past couple of decades with a great number of position stands, reviews, and research articles (Behm et al., 2008; Faigenbaum et al., 2009; Falk and Tenenbaum, 1996). However, since the balance and co-ordination of children are immature (Payne and Isaacs, 2005), the recommendations of many of these earlier reviews were for children to perform resistance exercises with low to moderate intensities or loads with higher repetitions to ensure safety and avoid injuries (Blimkie, 1993; Faigenbaum et al., 1996). More recently, the literature has accepted that youth with proper supervision and preparation can train with power movements such as plyometrics and Olympic style lifting (Behm et al., 2008). A CSEP position stand /review (Behm et al., 2008) recommended that resistance training progressions for children can be achieved by enhancing movement speed with plyometric drills and Olympic-style lifts. Similar to the present findings, a number of research articles have reported that plyometric training is highly effective and in some reports more effective than traditional resistance training for enhancing strength, power, sprint, agility, and balance performance. Eight (Meylan and Malatesta, 2009) and twelve week (Chaouachi et al., 2014a) plyometric training programs with early pubertal athletes found significant improvements in measures such as CMJ and hurdle jump height, sprint and agility test time (Meylan and Malatesta, 2009) as well as balance, isokinetic force, power, and 5-20-m sprints (Chaouachi et al., 2014a). Lloyd et al. (2016) examined eighty 12-16 year old youth and found an age dependent training response (6 weeks training) with plyometric training eliciting the greatest gains across all performance variables in pre-PHV children, whereas combined training (plyometrics and traditional resistance training) was the most effective in eliciting changes in all performance variables for the post-PHV cohort. However, de Hoyo et al. (2016) did not find a plyometric training advantage and reported similar training effects when employing low to moderate load strength training methods such as full-back squat, resisted sprint with sled towing, and plyometric and specific drills training (8 weeks) on sprinting, and jumping, with under 19-year-old soccer players. Compared to the other studies illustrating plyometric training advantages, deHoyo et al. (2016) employed a lower frequency (1-2/week vs. 2/week) and lower volume (1-3 sets vs. 2-5 sets) of training. Lesinski et al. (2016) in their systematic review and meta-analysis reported that plyometric training is effective in improving muscular strength, vertical jump height, sprint performance, agility, and sport-specific performance in the range of small-to-medium effect sizes in youth athletes. Similarly, both Fernandez-Fernandez et al. (2016) and Ramirez-Campillo et al. (2014) reported small to moderate magnitude performance enhancements with jumps, sprints, agility and other measures following 8 and 7 weeks of plyometric training with young tennis (12.5 ± 0.3 years) and soccer (13.2 ± 1.8 years) players respectively. On the other hand, Johnson et al. (2011) in their literature review on young children suggested that plyometric training had a large effect on improving the ability to jump, run, kicking distance, balance, and agility, but only a small effect on improving strength. Similarly, Sohnlein et al. (2014) also reported large magnitude improvements in sprint, agility, bounding, and long jump performance following 16 weeks of plyometric training of 11.2–14.7 year old soccer players. Contrary to the aforementioned reviews that reported small to medium sized effects, the present study showed generally large magnitude effects and substantial relative (31.3-32.6%) training-induced increases. A major contributor to these substantial gains may be the inclusion of balance exercises in the present study. There are strong correlations reported between balance measures and power (r = 0.511-0.827) in youth (Hammami et al., 2016a). A number of studies using both children and adults have reported performance enhancements with balance training alone without resistance training. Six weeks of balance training of elite male ski jumpers and Nordic athletes (14.5 ± 1.0 years) augmented squat and CMJ performance (Taube et al., 2007). Research studies using adult participants have also reported improved jump height performance following balance or sensorimotor training (Bruhn, 2001; Kean et al., 2006). These balance training-induced improvements have been ascribed to enhance anticipatory and task related EMG activity (Kean et al., 2006). Increased anticipatory muscle activation can stiffen the musculotendinous structures prior to and upon initiation of movement, optimizing the stretch-shortening cycle, reducing electromechanical delay and contact / amortization periods when running or jumping. It has also been rationalized that the erect human body acts as inverted pendulum and that enhanced balance would ensure less deviation from the vertical when performing vertical jumps optimizing the directionality of power production (Kean et al., 2006). Improved balance would also aid in maintaining a strong metastable base when sequencing from stability to instability when sprinting, agility, changing direction and other athletic maneuvers (Kibele et al., 2015). Plyometric power training with the associated high reaction forces, short contact (amortization) times and emphasis on movement velocity (Cappa and Behm, 2011; 2013) can lead to training-induced increases in motor unit recruitment, firing frequency, synchronization, decreased co-contractile (antagonist) activity, as well as increased muscle protein synthesis (Behm, 1995). Since an unstable base impairs force and power output (Behm et al., 2010), a more stable or balanced equilibrium upon landing or initiating movement during plyometric actions could augment the aforementioned plyometric (power)-training adaptations. The complementary contribution of balance training to plyometric training was demonstrated by Chaouachi et al. (2014b) with an eight-week training program (12-15-year-old boys) that combined plyometric and balance exercises. The combined training incorporated less than half the volume of plyometric exercises as the plyometric only program but was as effective or more effective (sprint, shuttle run, leg stiffness) than the plyometric only program for improving performance. A related research and practical question would then be; in order to optimize the balance and plyometric training synergy, is there a preferred order of training to achieve the greatest results? Hammami et al. (2016b) used youth soccer athletes aged 12-13 years to show that four weeks of balance training followed by four weeks of plyometric training produced similar or superior training improvements compared to the reverse order of training (four weeks of plyometric training prior to four weeks of balance training). It is interesting to note that the percentage improvements achieved at four weeks in the present study exceeded the relative improvements in the Hammami et al. (2016b) study suggesting that combining balance and plyometric exercises within the same training sessions provides greater training advantages than separating them into four week blocks. The present study tackled another related question as to whether there is an optimal within session sequence of balance and plyometric exercises. The results from the present study illustrated no significant training differences whether the balance and plyometric exercises were alternated in pairs (sequences of individual balance followed by plyometric exercise) or alternated as blocks of exercises (all balance exercises prior to all plyometric exercises). As the balance exercises are lower intensity activities, whether they are performed in an alternating fashion or as blocks, they did not seem to promote a consequential degree of fatigue to the subsequent plyometric exercises. However, the reverse order of plyometric exercises prior to balance measures has been shown to adversely affect balance and CMJ height; likely due to plyometric-induced fatigue (Romero-Franco and Jimenez-Reyes, 2015). The large magnitude and relative (%) changes with either of these routines might be facilitated by transient improvements in balance (aforementioned inverted pendulum effect) or a potentiating effect of the prior exercises. Potentiation of subsequent performance can occur with lower (Turki et al., 2011) and moderate (Chaouachi et al., 2011) intensity conditioning exercises. For example, 10 minutes of dynamic stretching were sufficient to potentiate vertical jump height and reaction force (Turki et al., 2011). Potentiation could involve peripheral muscle (i.e. myosin regulatory light chain phosphorylation) or neural (i.e. increased motor unit recruitment, rate coding or synchronization) mechanisms (Behm, 2004; Sale, 2002). A limitation of the present study is that the specific sample population of male, 14-year-old trained soccer athletes may limit the application of the findings to more diverse segments of the populations. Furthermore, longer duration (> 8 weeks) and higher frequency (>2 sessions / week) training programs might elicit different results. Further research should extend into different age groups, sexes, trained status with longer duration and higher frequency training programs. A further limitation is that the present study did not include a control group. However, it has previously been shown in (randomized) controlled studies that plyometric and balance training are effective means to enhance physical fitness in youth. In addition, our research hypothesis focused on specific sequencing effects of balance and plyometric exercises with one training session. We did not intend to evaluate whether balance and plyometric training are in general effective. Finally, from an ethical point of view it is not feasible to include (passive) control groups in an athletic population.
In conclusion, the combination of balance and plyometric exercises within an eight-week training program induced significant improvements in components of physical fitness (muscle strength, power, speed, agility, and balance) with male adolescent soccer athletes. However, the sequencing of the balance and plyometric exercises whether in an alternating or block arrangement did not substantially affected the extent of the positive training adaptations. When planning and implementing a plyometric training program for youth athletes, a similar volume of balance exercises should be incorporated into the training plan to optimize the training adaptations (Chaouachi et al., 2014b). While balance exercises should be emphasized early in the training program for youth and preferably prior to plyometric training (Hammami et al., 2016b), the sequence of within training session balance and plyometric exercises does not significantly affect the training outcomes. It is important to consider overall sequencing effects of balance and plyometric training based on our previous study (Hammami et al., 2016b) and with regards to mesocycles.
ACKNOWLEDGEMENTS |
The authors declare no conflicts of interest, financial or otherwise. |
|
AUTHOR BIOGRAPHY |
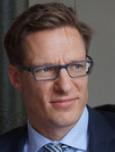 |
Urs Granacher |
Employment: Full Professor in Training and Movement Sciences at University of Potsdam, Germany |
Degree: PhD & Habilitation |
Research interests: Biomechanics, exercise science, postural control, muscle strength |
E-mail: urs.granacher@uni-potsdam.de |
|
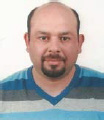 |
Issam Makhlouf |
Employment: Assistant professor at the high Institute of Sport and Physical Education, Sfax, Tunisia |
Degree: PhD |
Research interests: Soccer performance, training and testing |
E-mail: makhloufissam@yahoo.fr |
|
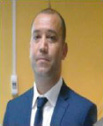 |
Raouf Hammami |
Employment: Assistant professor, National Center of Medicine and Science in sport (CNMSS), Tunis, Tunisia |
Degree: PhD |
Research interests: Exercise physiology |
E-mail: raouf.hammami@yahoo.fr |
|
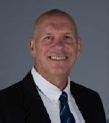 |
David G Behm |
Employment: University Research Professor |
Degree: PhD |
Research interests: Applied neuromuscular physiology and sport science |
E-mail: dbehm@mun.ca |
|
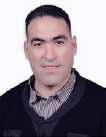 |
Anis Chaouachi |
Employment: Associate Professor and Head of the Tunisian Research Laboratory “Sport Performance Optimisation" National Center of Medicine and Science in sport (CNMSS), Tunis, Tunisia |
Degree: PhD & Habilitation |
Research interests: Applied physiology and sport science |
E-mail: anis.chaouachi@email.ati.tn |
|
|
|
REFERENCES |
 Behm D.G. (1995) Neuromuscular implications and applications of resistance training. The Journal of Strength and Conditioning Research 9, 264-274.
|
 Behm D.G. (2004) Force maintenance with submaximal fatiguing contractions. Canadian Journal of Applied Physiology 29, 274-290.
|
 Behm D.G., Colado Sanchez, J.C. (2013) Instability resistance training across the exercise continuum. Sports Health 5, 500-503.
|
 Behm D.G., Drinkwater E.J., Willardson J.M., Cowley P.M. (2010) The use of instability to train the core musculature. Applied Physiology, Nutrition, and Metabolism 35, 91-108.
|
 Behm D.G., Faigenbaum A.D., Falk B., Klentrou P. (2008) Canadian Society for Exercise Physiology position paper: resistance training in children and adolescents. Applied Physiology, Nutrition, and Metabolism 33, 547-561.
|
 Blimkie C.J. (1993) Resistance traing during preadolescence. Sports Medicine 15, 389-407.
|
 Bruhn S.G., Guber M. (2001) Proprioception training for the prevention and rehabilitation of knee joint injuries. European Journal of Sports Traumatology and Related Research 23, 82-89.
|
 Cappa D.F., Behm D.G. (2011) Training Specificity of Hurdle vs. Countermovement Jump Training. The Journal of Strength and Conditioning Research 25, 2715-2720.
|
 Cappa D.F., Behm D.G. (2013) Neuromuscular characteristics of drop and hurdle jumps with different types of landings. The Journal of Strength and Conditioning Research 27, 3011-3020.
|
 Chaouachi A., Hammami R., Kaabi S., Chamari K., Drinkwater E.J., Behm D.G. (2014a) Olympic weightlifting and plyometric training with children provides similar or greater performance improvements than traditional resistance training. The Journal of Strength and Conditioning Research 28, 1483-1496.
|
 Chaouachi A., Othman A.B., Hammami R., Drinkwater E.J., Behm D.G. (2014b) The combination of plyometric and balance training improves sprint and shuttle run performances more often than plyometric-only training with children. The Journal of Strength and Conditioning Research 28, 401-412.
|
 Chaouachi A., Poulos N., Abed F., Turki O., Brughelli M., Chamari K., Drinkwater E.J., Behm D.G. (2011) Volume, intensity, and timing of muscle power potentiation are variable. Applied Physiology, Nutrition, and Metabolism 36, 736-747.
|
 Cohen J. (1988) Statistical power for the behavioral sciences. Hillsdale, NJ. Erlbaum.
|
 Dalleau G., Belli A., Viale F., Lacour J.R., Bourdin M. (2004) A simple method for field measurements of leg stiffness in hopping. International Journal of Sports Medicine 25, 170-6.
|
 de Hoyo M., Gonzalo-Skok O., Sanudo B., Carrascal C., Plaza-Armas J.R., Camacho-Candil F., Otero-Esquina C. (2016) Comparative Effects of In-Season Full-Back Squat, Resisted Sprint Training, and Plyometric Training on Explosive Performance in U-19 Elite Soccer Players. The Journal of Strength and Conditioning Research 30, 368-377.
|
 de Villarreal E.S., Kellis E., Kraemer W.J., Izquierdo M. (2009) Determining variables of plyometric training for improving vertical jump height performance: a meta-analysis. The Journal of Strength and Conditioning Research 23, 495-506.
|
 Deurenberg P., Pieters J.J., Hautvast J.G. (1990) The assessment of the body fat percentage by skinfold thickness measurements in childhood and young adolescence. The British Journal of Nutrition 63, 293-303.
|
 Faigenbaum A.D., Chu D.A. (2001) Plyometric training for children and adolescents. Indianapolis. American College of Sports Medicine (ACSM) - Current Comment. Human Kinetics Publisher.
|
 Faigenbaum A.D., Kraemer W.J., Blimkie C.J., Jeffreys I., Micheli L.J., Nitka M., Rowland T.W. (2009) Youth resistance training: updated position statement paper from the national strength and conditioning association. The Journal of Strength and Conditioning Research 23, S60-S79.
|
 Faigenbaum A.D., Kraemer W.J., Cahill B., Chandler J., Dziados J., Elfrink L.D., Formann E., Gaudiose M., Micheli L., Nitka M., Roberts S. (1996) Youth resistance training: Position statement paper and literature review. Strength and Conditioning Journal 18, 62-75.
|
 Falk B., Tenenbaum G. (1996) The effectiveness of resistance training in children. Sports Medicine 22, 176-186.
|
 Faul F., Erdfelder E., Lang A.G., Buchner A. (2007) G*Power 3: a flexible statistical power analysis program for the social, behavioral, and biomedical sciences. Behavioral Research Methods 39, 175-191.
|
 Fernandez-Fernandez J., de Villarreal E.S., Sanz-Rivas D., Moya M. (2016) The Effects of 8-Week Plyometric Training on Physical Performance in Young Tennis Players. Pediatric Exercise Science 28, 77-86.
|
 Flanagan E.P., Comyns T.M. (2008) The Use of Contact Time and the Reactive Strength Index to Optimize Fast Stretch-Shortening Cycle Training. The Strength and Conditioning Journal 30, 32-38.
|
 Granacher U., Lesinski M., Büsch D., Muehlbauer T., Prieske O., Puta C., Gollhofer A., Behm D.G. (2016) Effects of resistance training in youth athletes on muscular fitness and athletic performance: A conceptual model for long-term athlete development. Frontiers in Physiology 7, 164-.
|
 Granacher U., Prieske O., Majewski M., Busch D., Muehlbauer T. (2015) The Role of Instability with Plyometric Training in Sub-elite Adolescent Soccer Players. International Journal of Sports Medicine 36, 386-94.
|
 Hammami R., Chaouachi A., Makhlouf I., Granacher U., Behm D.G. (2016a) Associations Between Balance and Muscle Strength, Power Performance in Male Youth Athletes of Different Maturity Status. Pediatric Exercise Science 28, 521-534.
|
 Hammami R., Granacher U., Makhlouf I., Behm D.G., Chaouachi A. (2016b) Sequencing effects of balance and plyometric training on physical performance in youth soccer athletes. The Journal of Strength and Conditioning Research 30, 3278-3289.
|
 Impellizzeri F.M., Rampinini E., Coutts A.J., Sassi A., Marcora S.M. (2004) Use of RPE-based training load in soccer. Medicine and Science in Sports and Exercise 36, 1042-1047.
|
 Johnson B.A., Salzberg C.L., Stevenson D.A. (2011) A systematic review: plyometric training programs for young children. The Journal of Strength and Conditioning Research 25, 2623-2633.
|
 Kean C.O., Behm D.G., Young W.B. (2006) Fixed foot balance training increases rectus femoris activation during landing and jump height in recreationally active women. Journal of Sports Science and Medicine 5, 138-148.
|
 Kibele A., Granacher U., Muehlbauer T., Behm D.G. (2015) Stable, Unstable and Metastable States of Equilibrium: Definitions and Applications to Human Movement. Journal of Sports Science and Medicine 14, 885-887.
|
 Lesinski M., Prieske O., Granacher U. (2016) Effects and dose-response relationships of resistance training on physical performance in youth athletes: a systematic review and meta-analysis. British Journal of Sports Medicine 50, 781-795.
|
 Lloyd R.S., Radnor J.M., De Ste Croix M.B., Cronin J.B., Oliver J.L. (2016) Changes in Sprint and Jump Performances After Traditional, Plyometric, and Combined Resistance Training in Male Youth Pre- and Post-Peak Height Velocity. The Journal of Strength and Conditioning Research 30, 1239-1247.
|
 Meylan C., Malatesta D. (2009) Effects of in-season plyometric training within soccer practice on explosive actions of young players. The Journal of Strength and Conditioning Research 23, 2605-2613.
|
 Mirwald R.L., Baxter-Jones A.D., Bailey D.A., Beunen G.P. (2002) An assessment of maturity from anthropometric measurements. Medicine and Science in Sports and Exercise 34, 689-694.
|
 Payne V.G., Isaacs L.D. (2005) . Human Motor Development. A Lifespan Approach. Toronto, Ontario. McGraw Hill Publishers.
|
 Ramirez-Campillo R., Meylan C., Alvarez C., Henriquez-Olguin C., Martinez C., Canas-Jamett R., Andrade D.C., Izquierdo M. (2014) Effects of in-season low-volume high-intensity plyometric training on explosive actions and endurance of young soccer players. The Journal of Strength and Conditioning Research 28, 1335-1342.
|
 Romero-Franco N., Jimenez-Reyes P. (2015) Unipedal Postural Balance and Countermovement Jumps After a Warm-up and Plyometric Training Session: A Randomized Controlled Trial. The Journal of Strength and Conditioning Research 29, 3216-3222.
|
 Rouissi M., Chtara M., Owen A., Chaalali A., Chaouachi A., Gabbett T., Chamari K. (2016) Effect of leg dominance on change of direction ability amongst young elite soccer players. Journal of Sports Science 34, 542-548.
|
 Sale D.G. (2002) Postactivation potentiation: role in human performance. Exercise and Sport Sciences Review 30, 138-143.
|
 Shrout P.E., Fleiss J.L. (1979) Intraclass correlations: uses in assessing rater reliability. Psychological Bulletin 86, 420-428.
|
 Sohnlein Q., Muller E., Stoggl T.L. (2014) The effect of 16-week plyometric training on explosive actions in early to mid-puberty elite soccer players. The Journal of Strength and Conditioning Research 28, 2105-2114.
|
 Taube W., Kullmann N., Leukel C., Kurz O., Amtage F., Gollhofer A. (2007) Differential reflex adaptations following sensorimotor and strength training in young elite athletes. International Journal of Sports Medicine 28, 999-1005.
|
 Turki O., Chaouachi A., Drinkwater E.J., Chtara M., Chamari K., Amri M., Behm D.G. (2011) Ten minutes of dynamic stretching is sufficient to potentiate vertical jump performance characteristics. The Journal of Strength and Conditioning Research 25, 2453-2463.
|
 Wadden K.P., Button D.C., Kibele A., Behm D.G. (2012) Neuromuscular fatigue recovery following rapid and slow stretch-shortening cycle movements. Applied Physiology, Nutrition, and Metabolism 37, 437-447.
|
|
|
|
|
|
|