|
|
|
ABSTRACT |
In recent years, there is an increasing importance of double poling (DP) performance regarding the outcome in classic cross-country skiing (XCS) races. So far, different approaches were used to predict DP performance but there is a lack of knowledge how general strength parameters are related to DP performance parameters gathered from in field-test situations. Therefore, the aim of this study was to determine the relationship between general strength measurements of different upper-body segments and pole force measurements during a DP sprint exercise. In addition, multiple linear regressions were calculated to determine the predictive power of theses variables regarding DP sprint performance, represented as maximum velocity. Thirteen none-elite cross-country skiers performed two 60 m DP sprints at maximal speed on a tartan track using roller skis. In addition, maximum isometric and concentric strength tests were performed on a motor-driven dynamometer with four major upper-body segments (trunk flexion / extension, shoulder / elbow extension). Especially the mean pole force and the strength test parameters correlated significantly (r ≥ 0.615) in all except one comparison. However, regression analyses revealed that neither pole force parameters (R² = 0.495) nor isometric (R² = 0.456) or dynamic (R² = 0.596) strength test parameters could predict the DP performance significantly. This study showed that standardized isokinetic strength tests could be used to estimate pole force capabilities of XCS athletes. However, pole-force and strength test parameters failed to predict significantly maximal velocity during a DP sprint exercise, which might be attributed to the non-elite subject group. |
Key words:
XCS, cross-country skiing, DP, isokinetic strength test, pole force measurements, field-test condition
|
Key
Points
- Pole force during DP sprint performance and general upper-body strength correlate to each other significantly in recreational skiers.
- DP sprint performance can neither be predicted by pole force nor by upper-body strength parameters significantly.
- Standardized isokinetic strength tests could be used to estimate pole force capabilities of xc-ski athletes.
|
In cross-country skiing (XCS), the double poling (DP) technique is getting more and more important in classical races. Nowadays, an increasing number of classic sprint competitions are performed completely with the DP technique. Furthermore, the DP technique, used at the finish line often decides the outcome in classical technique mass start competitions. This development can be explained by enhanced upper-body strength capacities, combined with enhanced technical aspects in elite skiers, as well as better track preparation, and superior equipment (Holmberg et al., 2005). Therefore, it is important to understand the basic mechanisms to gain maximal DP velocity. Technical aspects, such as longer cycle length, play an important role (Stöggl and Holmberg, 2011a; Stöggl et al., 2007), but also depend on strength parameters. Whilst leg and trunk strength are known to be important factors to increase DP performance (Rud et al., 2014; Zoppirolli et al., 2017), it has been suggested that upper-body power is a decisive factor in XCS sprint (Stöggl et al., 2007). However, most of the previous studies concerning strength in DP were performed as XCS specific strength tests on a cable pulley (Hoff et al., 1999; Østerås et al., 2002), a DP ergometer (Nilsson et al., 2004) or a rollerboard (Stöggl et al., 2007) and referred to the DP performance on an ergometer or a treadmill. Hence, there is a lack of studies relating general strength, i.e., strength measured separately for different body parts to direct field-test parameters like pole force and maximal velocity (Vmax) in sprint distance. Only a few studies dealing with general strength in DP, using correlation and regression analysis to reveal that upper-body strength parameters have a profound impact on Vmax (Mikkola et al., 2010; Ng et al., 1988; Østerås et al., 2016; Stöggl et al., 2011b). However, there is, to date, only one previous study that has recorded both pole force and strength parameters in concert (Stöggl et al., 2011b). For many winter sport disciplines, several concepts for general strength tests have been developed. Olympic Training Centers (e.g. Olympic Training Center of Bavaria) regularly apply trunk and leg strength tests for elite athletes (e.g. hockey, bobsleigh) on a motor-driven dynamometer. To the best of the authors’ knowledge, in XCS these tests rarely exist, although they could give e.g. insights into the muscular status of an athlete using a high-standardized setup or allow cross-sectional and longitudinal comparisons among different athletes. It is hypothesized that the resulting pole forces and general maximum strength in the upper-body segments relate to each other strongly and predict DP performance at maximal velocity.
Study populationThirteen recreational cross-country skiers volunteered to participate in the present study. The study population consisted of two females and eleven males participants, who performed XCS races in their adolescence regularly. The median of self-reported fitness of the athletes was 2 (3-4 non-specific training session/week), on a scale from 1 (very good) to 6 (very poor). The skiers` mean ± SD age was 29 ± 4 years, height was 1.82 ± 0.06 m, weight was 77 ± 6 kg, classical pole length was 1.55 ± 0.06 m. The subjects were instructed to carry out only light training two days before the measurements. Participants were informed about the design of the study, with special information on possible risks and benefits, and subsequently voluntarily signed an informed consent document before the start of the study. The ethic statement for this study was approved by the Dean of the Faculty of Sports and Health Sciences of the Technical University Munich. All tests were conducted according to the Declaration of Helsinki.
Experimental setupTwo pairs of classic roller skis (same model) were used for the test (Marwe Oy, Hyvinkää, Finland) and skiers used their own ski boots. One pair of classic roller skis had a NNN (Rottefella) binding system (n = 9), and the other had a SNS (Salomon) binding system (n = 4). Prior to the test, the roller skis were pre-warmed by the skiers on the tartan track for 15 minutes, to ensure the same rolling resistance for each skier during the measurements. One-dimensional piezoelectric force transducers (Kistler Instrumente AG, Winterthur, Switzerland, length 4.5 cm, diameter 2.2 cm, weight 95 g were firmly attached (screw system) on the proximal end of the poles, underneath the grip in line with the long axis of the poles. To avoid the influence of signal drifting, the force transducers were reset immediately prior to each trial. The signals were recorded at a sampling rate of 256 Hz and stored via cable on a data logger (MSR165, MSR Electronics GmbH, Seuzach, Switzerland) placed in a belt bag. Four pairs of poles (LEKI-Sport, HM-Carbon, Kirchheim/Teck, Germany) were available to host the force transducer. Each pair was adjustable in length (7 cm) and was adapted to each individual’s classic pole length (85.0 ± 1.5% of body height). The torque of the different joints was measured with a sampling rate of 200 Hz on a motor-driven dynamometer (IsoMed 2000, D&R Ferstl GmbH, GER). During all tests, subjects were seated (backwards inclination 70° for the arm strength tests) and fixed by straps to avoid redundant movements (Figure 1 a-c).
Experimental protocolThe DP test and the strength test were performed during a 14-day period with a minimum break of 24 hours in between. For familiarization of the isokinetic strength tests, all subjects did a complete test-setup in advance. The DP test took place on an outdoor tartan track. In a first step, subjects performed a 15 min lasting DP warm-up at a self-selected effort with their own poles. Subsequently, the skiers had a passive recovery (10-15 min), to get the sensor poles and the wires adapted. The second warm-up lasted 5 min including a 60 m test sprint with the sensor poles. For the main test which started right after the second warm-up, subjects had to accelerate 40 m to reach maximal speed, immediately followed by a 60 m DP sprint. The minimum break between the two maximum trials was 3 minutes. The mean velocity of the 60 m DP sprint is called Vmax in the following manuscript. At first, part of the subjects complained about the slow surface of the tartan track, but quickly adapted. Some of the subjects actually trained regularly on tartan track. The strength tests started with a 15 min warm-up on a rowing ergometer at a self-selected resistance. The test session included an isometric and concentric test each consisting of trunk flexion and extension as well as left and right elbow and shoulder extension measurements. For both maximum voluntary isometric and concentric tests, the subjects were advised to develop force as quickly as possible and try to maintain the strength. During each test, the instructor gave maximum verbal encouragement. The isometric contraction lasted approximately 5 s. The concentric tests were performed as an extension-flexion motion, three times in a row without a break at the turning points. Three repetitions were chosen to allow the subjects to get accustomed to the fast movement. Each testing condition consisted of three trials with a three-minute break in between. The sequence of trunk, shoulder and elbow tests occurred in a randomized order (Figure 2). Isometric trunk flexion and extension tests were done seated in the reference position (0°), defined as upper body perpendicular to the thighs (Figure 1a). For the dynamic trunk tests the range of motion (ROM) was 60° (40° flexion - 20° extension) at an angular velocity of 60°/s. For the concentric and isometric shoulder and elbow tests, joint angles and velocities were chosen specifically for the purpose of XCS, according to the literature (Holmberg et al., 2005; Lindinger et al., 2009; Zoppirolli et al., 2015). Elbow extension (left and right) was done with a flexion angle of 45° (0° = straight arm) and an angle of 45° between upper arm and backrest (Figure 1b). The tests for shoulder extension were performed with a flexion angle of 90° (0° = neutral arm position; Figure 1c) and an almost fully extended elbow joint. The ROM for shoulder extension was 85° (5° - 90° shoulder flexion angle) and 110° for the elbow extension (0° - 110° elbow flexion angle). For both tests the angular velocity of joint extension was 300°/s (Lindinger et al., 2009; Zoppirolli et al., 2013), whereas the joint flexion was 150°/s to allow the subjects to focus on the joint extension.
Data analysisThe time of the 60 m DP sprint was measured with light barriers placed on the official floor markings of the track and field facility. Hence, the mean velocity was calculated as Vmax = d/t, where d is the distance (60 m) and t = time of 60 m DP sprint. The pole force as well as the torque data (left and right) were analyzed in the software ProEMG (prophysics AG, Kloten, Switzerland, v2.1.0.1). The analysis of the pole force data was done on the raw data and only the fastest DP trial was analyzed. Poling parameters were calculated as means of five consecutive cycles from pole contact (> 10 N) until the pole leaves the ground (< 10 N; Figure 3). For mean pole force (MPF) the average value and for peak pole force (PPF) the maximum value, excluding the impact pole force peak (Figure 3) from pole contact to end of poling were considered. Cycle length was calculated as cycle time multiplied by Vmax [m] and cycle rate as the reciprocal value of cycle time [Hz]. Torque data was filtered with a low-pass hardware filter of 200 Hz (6th order). The highest value from each strength test (isometric: one out of three repetitions; concentric: one out of 9 repetitions) was chosen for further calculations. For the isometric MVC contractions, the peak values were taken (Mmax, ISO). During the dynamic contractions, only the joint extension phase was analyzed (flexion phase for trunk flexion), thereby using the value at the same angular positions as for the isometric contractions (Mmax, CON). In addition, the average torque of the joint extension phase (flexion phase for trunk flexion) was calculated (MRMS). Values recorded at the beginning and ending of the concentric trunk strength (each 4°) and arm strength tests (each 2°) were excluded from the analysis due to inertial properties of the mechanical lever arm.
Statistical analysisResults are presented as means and standard deviation (± SD). Normality of data was checked using the Kolmogorov-Smirnov test. Differences between the left and right side were tested using a Student’s T-test for paired groups. To determine the relationship between strength variables, pole force and Vmax, Pearson’s product-moment correlations were calculated. A multiple linear regression procedure (enter method) was applied to determine the best predictors for 60 m DP performance. Only those variables showing a significant correlation to Vmax were used for the multiple linear regression. Pole force parameters and strength test parameters for left and right arms were summarized if necessary and normalized on the athletes’ body weight for regression analyses. A value of p < 0.05 was considered significant. Based on a relatively small study population, regression analyses had to be performed individually for each variable and cluster of variables with different muscles and joints. All statistical tests were processed using IBM SPSS 19.0 Software for Windows (SPSS Inc., Chicago, IL, USA).
Maximal velocity of the 13 subjects was 21.64 ± 2.69 km/h over 60 m. The occurring PPF over 60 m sprint performance was 324.8 ± 58.7 N (right) / 311.3 ± 65.7 N (left) and the MPF was 178.2 ± 33.2 N / 169.9 ± 32.0 N. Cycle length was 4.38 ± 0.58 m and cycle rate 1.39 ± 0.19 Hz on average. Only MPF was significantly higher (4.7%, p = 0.047) in the right pole than in the left. For the strength tests, Mmax, ISO was 181.2 ± 27.2 Nm for trunk flexion, 346.9 ± 65.9 Nm for trunk extension, 127.3 ± 27.3 Nm (right) / 123.0 ± 24.7 Nm (left) for shoulder extension and 81.7 ± 17.5 Nm / 75.1 ± 15.3 Nm for elbow extension (Figure 4). The ratio between maximum isometric trunk flexion and extension torque was 53 ± 8%. Regarding Mmax, CON results were as follows: 152.4 ± 29.9 Nm for trunk flexion, 266.4 ± 59.5 Nm for trunk extension, 61.4 ± 14.6 Nm / 59.5 ± 12.6 Nm for shoulder extension and 43.4 ± 9.3 Nm / 38.9 ± 7.9 Nm for elbow extension (Figure 4). Maximum isometric and concentric elbow extension strength (Mmax, ISO and Mmax, CON) were significantly higher (7.6 ± 9.2 / 9.5 ± 11.7%, p = 0.010 / 0.014) in the right elbow than in the left. The subjects could achieve MRMS of 153.9 ± 26.9 Nm for trunk flexion, 274.9 ± 45.9 Nm for trunk extension, 82.4 ± 18.2 Nm / 75.1 ± 16.0 Nm for shoulder extension and 46.9 ± 9.3 Nm / 44.7± 8.7 Nm for elbow extension (Figure 4). Mean concentric strength (MRMS) of shoulder and elbow extension were significantly higher (8.2 ± 9.3 / 4.4 ± 7.2%, p = 0.011 / 0.044) in the right limb than in the left. The ratio between mean concentric trunk flexion and extension torque was 57 ± 8%. Regarding correlations, Mmax, ISO of the trunk correlated only to MPF (Table 1). However, there were significant correlations between Mmax, CON and MRMS trunk torque to PPF and MPF (Table 1). Maximum isometric shoulder extension torque correlated highly significant to MPF and significantly to PPF (Table 1). Concentric (Mmax, CON and MRMS) shoulder extension torque correlated only to MPF (Table 1). Maximum isometric and concentric elbow extension strength correlated significantly to PPF and MPF. In total, mean pole force (MPF) had the highest number of significant correlations to the isometric and concentric strength test parameters (17 out of 18), followed by peak pole force (7 out of 18; Table 1). The correlation analysis regarding Vmax to determine the predictors used in the regression analyses, showed significant results for all presented variables ranging from r = 0.572 (MRMS SE) to r = 0.758 (MPF; Table 2). Regression analyses could not reveal any significant predictors of maximal DP velocity (Table 3). However, their predictive power could be arranged according to their adjusted coefficient of determination (R²). Accordingly, Mmax, CON of trunk flexion, trunk extension, elbow extension and shoulder extension gave the best prediction (R2 = 0.596), followed by PPF and MPF (R2 = 0.495) and Mmax, ISO of trunk flexion, trunk extension, elbow extension and shoulder extension (R2 = 0.456; Table 3). There was no consistency in the order of β parameter estimates concerning the four different muscle segment movements trunk flexion, trunk extension, elbow extension and shoulder extension. However, trunk strength parameters maintained β values of 0.238 and higher in both concentric and isometric strength tests, whereas elbow and shoulder extension strength parameters did not (β ≥ 0.095). MPF influenced the DP performance higher compared to PPF (MPF: β = 0.603; PPF: β = 0.168; Table 3).
In the present study general upper-body strength factors were related to each other and to a 60 m DP sprint on roller skis, on a slow surface (tartan track), considering the background that short-duration maximal speed in DP is a good predictor for DP sprint performance over race distance (1000-1500 m) (Stöggl et al., 2006). Therefore, pole forces were captured during a DP test and isometric and concentric maximum strengths in the elbow, shoulder, and trunk segments, each measured in isolation, were recorded on a motor-driven dynamometer. In addition, we have tried to quantify the importance of the different upper-body segments for achieving high DP velocities based on our athletes. The correlation analysis revealed that almost all general strength parameters (except MRMS of shoulder extension left) showed a strong correlation regarding mean pole force (r > 0.615). However, for PPF the results were not that obvious. There was less consistency regarding the correlation between PPF and the general strength parameters. Strength parameters measured in isolation, either under isometric conditions or with defined velocities and angles might not be associated with PPF in such a meaningful way as they are with MPF. Regression analyses were not significant (R² ≥ 0.456, p ≥ 0.080) and should be contemplated with caution. Despite a comparable number of athletes as in a previous study by Østerås et al. (2016), the missing of significant results might be due to the more heterogeneous subject group in the present study. Regarding the beta weights of the strength test, especially the trunk strength showed consistent results (β = 0.238 – 0.466). The same applies to the results of shoulder extension but with a lower total effect power (β = 0.111 – 0.178) whereas elbow extension varied a lot between the isometric and dynamic test (β = 0.095 – 0.320). The general problem using non-elite subjects is that most of the current research in XCS is done with at least national-elite athletes. Hence, to draw conclusions for this specific population it is first necessary to classify the recreational skiers. In general, the subjects classified themselves as an active subject group based on a 6-point Likert scale (median = 2; 3-4 non-specific training sessions per week). Regarding strength parameters the subjects showed lower values compared to Ng et al. (1988). The authors tested recreational XCS athletes that participated in a XCS race series. Comparing the results reveal a difference of 31% for maximum shoulder extension torque (MRMS: 108.1 vs. 82.4 Nm). Despite having comparable subject groups, the differences might be explained by the fact that the exact ROM in the study of Ng et al. (1988) is not presented and the angular velocity was 60°/s less, resulting in higher torque values according to the force-velocity relationship (Hill and Sec, 1938). A recently published study (Østerås et al., 2016) showed a relationship for concentric shoulder and elbow extension contractions of ~70% (elbow extension/shoulder extension, measured as 1RM) for elite female XCS athletes. In this study using MRMS, elbow extension made up only 60% (left) and 58% (right) of shoulder extension, signifying a lower elbow extension strength level for the participating subjects. The trunk strength values of the subjects were difficult to classify due to a lack of comparable studies. Comparing pole force and general poling parameters of elite XCS athletes, the non-elite skiers in this study demonstrated a 72.8 N (right pole: 324,8 vs. 252 N) (Rapp et al., 2010) and 83.5 N (right pole: 324,8 vs. 241,3 N) (Lindinger et al., 2009) higher PPF at Vmax, respectively. Additionally, compared to Lindinger et al. (2009) the poling frequency at Vmax in this study was higher (1.39 vs 1.08 Hz) concomitant with a shorter maximal cycle length (4.37 vs. 7.68 m). The higher peak pole force and poling frequency for the recreational skiers might result from the slower surface (tartan track vs. treadmill) as the test setups were similar (60 m sprint performance ~ 14 DP cycles vs. 15 complete DP cycles) (Lindinger et al., 2009). To maintain the highest possible velocity during the 60 m sprint, the athletes had to overcome the higher friction of a tartan track, resulting in higher poling frequency and shorter cycle length compared to maximal double poling on a treadmill. However, in some races during the competition season, the gliding conditions may be slow because of low ambient temperatures or wet weather conditions. Therefore, this investigation, performed on an outdoor tartan track closely resembles to slow XCS competitions. Previous findings of DP velocity on a tartan track (Stöggl et al., 2006) are comparable to those in this study. It should be noted that we cannot ensure that the rolling resistance of the two pairs of roller skis used in this study (even if they were the same model) was identical and therefore cannot exclude a bias based on individual rolling resistance in our data. Additionally, there were different weather conditions in the field test (classified in 3 categories: 1 = mostly nice with dry track (5 subjects); 2 = mostly nice with wet track (5 subjects); and 3 = mostly rainy with wet track (3 subjects), which might have influenced the participants’ performance. Especially the comparison of pole forces and general strength has been neglected so far. Only Stöggl et al. (2011b) confirmed that PPF and the impulse of pole force in DP at submaximal speeds were related significantly to 1RM in bench pull and maximal power output in bench press. In the present study, MPF presented a good parameter to compare to the upper-body strength tests. Isometric and concentric trunk and arm strength showed almost similar importance to reach high MPF. Regarding the DP technique and propulsion, the trunk should have a more stabilizing function in comparison to the arms. However, the DP technique has developed over the previous decades. In the past, it was recommended to fully extend in the elbow joint, whereas nowadays the arms stay more benched throughout the whole DP cycle, due to a smaller minimum elbow angle (Holmberg et al., 2005). Holmberg et al. (2005) defined this technique as ‘wide elbow’ pattern. The elbows are stretched out sidewise, whereas the hands stay close to the trunk. Comparing the isometric with the dynamic upper-body muscle strength tests, the present study showed, the concentric strength tests to be more predictive (R² = 0.596, n.s.) for DP sprint performance than the isometric test (R² = 0.456, n.s.), which is logical since XCS is a dynamic sport. Other authors confirmed the positive contribution of concentric upper-body strength to maximal velocity (Stöggl et al., 2011b) sprint performance (Østerås et al., 2016) and 10 km race performance (85% of Vmax) (Ng et al., 1988). The prediction probability of the pole forces is slightly increased compared to the isometric test (R² = 0.495, n.s.). Additionally, the multiple regression of the present study showed that MPF had a greater effect on Vmax compared to PPF (β: 0.603 vs. 0.168). Holmberg et al. (2005) found a positive correlation for both relative (r = 0.66) and absolute (r = 0.70) PPF to 85% of Vmax, resembling the correlation results in this study for PPF and MPF (Table 2). Quantifying the importance of the different upper-body segments for achieving high DP velocities, Østerås et al. (2016) concluded that the impact of maximum strength in elbow and shoulder segments increased with increasing demands of power production (3-min test: r = 0.54 (n. s.) and 0.58; 30-s test: r = 0.87 and 0.89). On the other hand, maximum trunk flexion strength had a similar importance for poling efficiency and power output across the whole intensity spectrum (3-min test: r = 0.66; 30-s test: r = 0.65). The correlation results for shoulder and elbow extension of the 30-s test presented by Østerås et al. (2016) are higher compared to those of the 60 m test in the present study (r = 0.673 / 0.729; Table 2), whereas the results of trunk flexion are similar. A reason for the lower correlation might be that compared to elite athletes, recreational skiers cannot use their full muscle potential for the propulsion due to a less efficient poling technique and intermuscular coordination (e.g. propulsive force) (Stöggl and Holmberg, 2015) especially at high velocities. Besides, the differences regarding the overall test setup (cable pulley ergometer vs. DP sprint) can be another reason for the differences, as the planting of the pole has a higher coordinative component compared to tests on a cable pulley. Additionally, Østerås et al. (2016) used common resistance machines for 1-RM tests to determine the strength abilities. Hence, regarding the dynamic strength test in this study, differences in velocity, range of motion as well as different position for assessing the peak values could further influence the results. Furthermore, the beta values of the multiple regression showed that the concentric trunk strength (trunk flexion: β = 0.466; trunk extension: β = 0.270) had more influence on Vmax compared to relative shoulder (β = 0.178) and elbow (β = 0.095) strength (Table 3). The results of the trunk tests are in accordance with Østerås et al. (2016) as well as Mikkola et al. (2010). Taken together these studies demonstrate the importance of trunk performance capacities regarding the production of propulsive force in XCS.
ImplicationIn practice, it is possible to utilize concentric and isometric upper-body strength tests to reach judgments about the DP force, especially the mean pole force (Table 1). Further diagnostic tests, particularly for sprint skiers, could be enriched by maximum strength tests. A pure concentric strength test is conceivable as well and would minimize the measuring effort. Given the fact that there were only slight differences between left and right arm strength (Mmax, CON shoulder extension: 1.9 Nm, elbow extension: 4.5 Nm), it is also possible to test only one arm of the skier. However, it is important to point out that higher torque values in the strength test did not necessarily induce higher pole forces and the other way around. Technique and coordination patterns also are essential parameters to reach high DP velocities (Stöggl et al., 2011b).
Pole forces (especially MPF), obtained at maximal velocity and general upper-body strength related to each other strongly. General upper-body strength and pole force parameters did not predict maximal DP velocity significantly. Nevertheless, the concentric strength testing is a measuring method with a high potential regarding the increasing influence of the upper-body strength in today’s cross-country skiing. Future research should help to clarify the importance of the strained upper-body muscles in DP.
ACKNOWLEDGEMENTS |
The authors like to thank the skiers including the cross-country ski team ‘Forever Nordic’ for their participation and cooperation during the study. Many thanks also to the Olympic Training Center of Bavaria for providing the possibilities to conduct the general strength tests. This work was supported by the German Research Foundation (DFG) and the Technical University of Munich (TUM) in the framework of the Open Access Publishing Program. There is no conflict of interest between the authors. The experiments comply with the current laws of Germany. |
|
AUTHOR BIOGRAPHY |
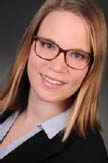 |
Esther Mende |
Employment: Department of Sport and Health Sciences, Technical University of Munich, Germany |
Degree: MSc |
Research interests: The analysis of strength parameter especially in the field of double poling. |
E-mail: Esther.Mende@mri.tum.de |
|
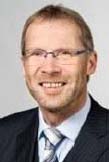 |
Ansgar Schwirtz |
Employment: Head of the Professorship of Biomechanics in sports, Technical University of Munich. In addition he supervises and consults national athletes of the Olympic Training Center of Bavaria and is leading the scientific commission in Nordic winter sports (Ski-jumping, Nordic-combined). |
Degree: PhD |
Research interests: Nordic winter sports (Ski-jumping, Nordic-combined). |
E-mail: Ansgar.Schwirtz@tum.de |
|
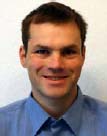 |
Florian K. Paternoster |
Employment: A research fellow at the Professorship of Biomechanics in sports, Technical University of Munich. |
Degree: PhD |
Research interests: Exercise physiology and exercise immunology |
E-mail: Florian.Paternoster@tum.de |
|
|
|
REFERENCES |
 Hill A.V., Sec R.S. (1938) The heat of shortening and the dynamic constants of muscle. Proceedings of the Royal Society of London. Series B - Biological Sciences 126, 136-195.
|
 Hoff J., Helgerud J., Wisløff U. (1999) Maximal strength training improves work economy in trained female cross-country skiers. Medicine & Science in Sports & Exercise 31, 870-877.
|
 Holmberg H.C., Lindinger S., Stöggl T., Eitzlmair E., Müller E. (2005) Biomechanical analysis of double poling in elite cross-country skiers. Medicine & Science in Sports & Exercise 37, 807-818.
|
 Lindinger S.J., Stöggl T., Müller E., Holmberg H.C. (2009) Control of speed during the Double Poling Technique performed by Elite Cross-Country Skiers. Medicine & Science in Sports & Exercise 41, 210-220.
|
 Mikkola J., Laaksonen M., Holmberg H.C., Vesterinen V., Nummela A. (2010) Determinants of a simulated cross-country skiing sprint competition using V2 skating technique on roller skis. Journal of Strength and Conditioning Research 24, 920-928.
|
 Ng A.V., Demment R.B., Bassett D.R., Bussan M.J., Clark R.R., Kuta J.M., Schauer J.E. (1988) Characteristics and Performance of Male Citizen Cross-Country Ski Racers. International Journal of Sports Medicine 09, 205-209.
|
 Nilsson J., Holmberg H.C., Tveit P., Hallen J. (2004) Effects of 20-s and 180-s double poling interval training in cross-country skiers. European Journal of Applied Physiology 92, 121-127.
|
 Rapp, W., Holmberg, H.C. and Lindinger, S. (2010) Muskuläre
Aktivierung von Arm und Schultermuskeln beim
Doppelstockeinsatz im Skilanglauf. In: Biomechanik -
Grundlagenforschung und Anwendung. Eds: Wank, V. & Heger,
H., 197 Schriften der Deutschen Vereinigung für
Sportwissenschaft. Tübingen: Feldhaus Verlag, 181-189.
|
 Rud B., Secher N.H., Nilsson J., Smith G., Hallén J. (2014) Metabolic and mechanical involvement of arms and legs in simulated double pole skiing. Scandinavian Journal of Medicine & Science in Sports 24, 913-919.
|
 Stöggl T., Holmberg H.C. (2011a) Force interaction and 3D pole movement in double poling. Scandinavian Journal of Medicine & Science in Sports 21, e393-e404.
|
 Stöggl T., Holmberg H.C. (2015) Three-dimensional Force and Kinematic Interactions in V1 Skating at High Speeds. Medicine & Science in Sports & Exercise 47, 1232-1242.
|
 Stöggl T., Lindinger S., Müller E. (2006) Reliability and Validity of Test Concepts for the Cross-Country Skiing Sprint. Medicine & Science in Sports & Exercise 38, 586-591.
|
 Stöggl T., Lindinger S., Müller E. (2007) Evaluation of an upper-body strength test for the cross-country skiing sprint. Medicine & Science in Sports & Exercise 39, 1160-1169.
|
 Stöggl T., Müller E., Ainegren M., Holmberg H.C. (2011b) General strength and kinetics: fundamental to sprinting faster in cross country skiing?. Scandinavian Journal of Medicine & Science in Sports 21, 791-803.
|
 Zoppirolli C., Holmberg H.C., Pellegrini B., Quaglia D., Bortolan L., Schena F. (2013) The effectiveness of stretch–shortening cycling in upper-limb extensor muscles during elite cross-country skiing with the double-poling technique. Journal of Electromyography & Kinesiology 23, 1512-1519.
|
 Zoppirolli C., Pellegrini B., Bortolan L., Schena F. (2015) Energetics and biomechanics of double poling in regional and high-level cross-country skiers. European Journal of Applied Physiology 115, 969-979.
|
 Zoppirolli C., Pellegrini B., Modena R., Savoldelli A., Bortolan L., Schena F. (2017) Changes in upper and lower body muscle involvement at increasing double poling velocities: an ecological study. Scandinavian Journal of Medicine & Science in Sports 27, 1292-1299.
|
 Østerås H., Helgerud J., Hoff J. (2002) Maximal strength-training effects on force-velocity and force-power relationships explain increases in aerobic performance in humans. European Journal of Applied Physiology 88, 255-263.
|
 Østerås S., Welde B., Danielsen J., Tillaar R.V., Ettema G., Sandbakk O. (2016) The contribution of upper-body strength, body composition and maximal oxygen uptake to predict double poling power and overall performance in female cross-country skiers. Journal of Strength and Conditioning Research 30, 2557-2564.
|
|
|
|
|
|
|