|
|
|
ABSTRACT |
We aimed to compare the urinary titin N-terminal fragment (UTF) concentration after concentric and eccentric exercise and to clarify the specific response of UTF to exercise-induced muscle damage (EIMD). Nine healthy young men performed 30 concentric elbow flexion exercises with maximum effort, rested for at least eight weeks, and performed eccentric exercises at the same workload using the same arm. Changes in the maximal voluntary isometric contraction (MVIC), muscle soreness (SOR), range of motion (ROM), serum creatine kinase (CK) activity, and UTF concentrations were recorded before and after for six consecutive days after exercise. There was no significant difference in workload during exercise between the two exercise types. However, serum CK activity increased after eccentric exercise (p < 0.05). Additionally, MVIC, SOR, ROM, and UTF concentration were significantly higher after eccentric exercise than after concentric exercise (p < 0.05). Although workload was the same, the UTF concentration greatly increased after eccentric exercise. Based on these results, we suggest that UTF can be a non-invasive and highly specific biomarker of EIMD. |
Key words:
Biomarker, exercise-induced muscle damage, delayed onset muscle soreness, validity, non-inversive
|
Key
Points
- This study investigated urinary titin N-terminal fragment (UTF) concentration after concentric and eccentric exercise performed at the same workload.
- Although both exercises were performed at the same workload, UTF concentrations significantly differed between the concentric and eccentric conditions.
- UTF will be useful as a new biomarker for specific measurement of EIMD because it UTF specifically responded to EIMD after eccentric contraction.
|
Exercise, including eccentric contraction and any movement that a person is unaccustomed to, can result in exercise-induced muscle damage (EIMD) (Clarkson and Hubal, 2002; Cleak and Eston, 1992). In EIMD, muscle fibers are disrupted (Yu et al., 2003), leading to inflammation that can last as long as 30 d (Nosaka and Clarkson, 1996). This causes a decrease in muscle strength and range of motion (ROM) of the joints and also a decrease in physical performance, including impaired balance and sprint ability (Khan et al., 2016). The parameters used to measure the extent of EIMD include muscle soreness (SOR), strength, ROM, swelling, and biochemical analysis (Clarkson and Hubal, 2002; Hyldahl et al., 2017). In a previous study, the extent of EIMD after 2, 6, and 24 eccentric exercises performed with maximum effort was examined. Relative to pre-exercise values, the maximum isometric torque 2 d after exercise reduced by approximately 20%, 33%, and 56%, respectively, and the peak values of serum creatine kinase (CK) activity increased by approximately 2-fold, 323-fold, and 1591-fold, respectively (Nosaka et al., 2001a). Conversely, although participants performed 100 repetitions (10 times × 10 sets) of the concentric exercise, elbow flexion, with maximum effort, the maximum isometric torque 2 d after exercise only reduced by approximately 10% compared with the pre-exercise value, and the CK activity hardly altered in any of the groups (Bottas et al., 2005). In addition to the symptoms described above, it was recently reported that the N-terminal fragment of titin leaks into urine following eccentric contraction exercise (Kanda et al., 2017). Titin, a main determinant of the extensibility of sarcomeres in muscles, is a giant tandem modular protein anchored to myosin and the Z-disc in sarcomeres (Eckels et al., 2018). Urinary titin N-terminal fragment (UTF) was initially detected in patients with Duchenne muscular dystrophy (Rouillon et al., 2014), followed by that in healthy individuals after eccentric contraction (Kanda et al., 2017). UTF concentrations reach a peak at 96 h after eccentric exercise (Yamaguchi et al., in press), and this peak is significantly correlated with other indices of muscle damage, such as isometric strength (r ≤ —0.485), ROM (r ≤ —0.485), SOR (r ≥ 0.549), and CK activity (r ≥ 0.647) (Yamaguchi et al., 2020). Although UTF concentrations get altered following eccentric contraction, it is unclear whether any changes occur after concentric contraction. Therefore, we aimed to investigate UTF concentrations after concentric and eccentric contraction exercises. Our hypothesis, which is based on the study by Bottas et al. (2005), was that UTF concentrations will remain unchanged after concentric exercise but would significantly increase after eccentric exercise. If UTF concentrations do not alter following exercise involving concentric contractions, which induce little muscle damage, UTF should only be detected specifically following EIMD caused by eccentric contraction exercises.
Participants and study designWe recruited nine healthy men (age: 22.9 ± 1.7 years; weight: 69.5 ± 9.2 kg; height: 172.5 ± 5.0 cm; mean ± SD) with no injuries of the muscles of their upper limbs and who had not performed regular resistance training in the 6 months prior to the experiment. We explained the purpose, procedures, risks, and benefits of the study to potential participants; they provided written informed consent before participating. The experiment was conducted according to the Declaration of Helsinki and was approved by the Waseda University ethics committee (2017-162). We frequently reminded the participants to abstain from performing any strenuous physical activities or activities that they were unaccustomed to and to refrain from taking anti-inflammatory medication during the experiment. Body weight was measured using a multifrequency impedance body composition analyzer (InBody720 Inbody Japan Inc., Tokyo, Japan), and height was measured using a wall-mounted stadiometer (SECA 213, Yagami Inc., Nagoya, Japan). The participants performed a bout of concentric exercises (CON) comprising three sets of 10 maximal concentric contractions using the dominant arm and a bout of eccentric exercises (ECC) comprising the same number of contractions using the same arm but separated by >8 weeks. All participants were allowed to choose their dominant arm for exercise because Newton et al. (2013) demonstrated that muscle damage does not differ between the dominant and nondominant arms. Moreover, to unify the work intensities for both conditions, CON and ECC were performed at the same workload measured using an isokinetic dynamometer. The reason why we chose an interval of 8 weeks between bouts was that the results of a previous study revealed that EIMD has a protective effect against subsequent muscle damage; any muscle damage induced by eccentric exercise performed within several days of another exercise is significantly reduced (Hyldahl et al., 2017; Yamaguchi et al., in press). This protective effect can last for 12 weeks (Nosaka et al., 2005); stronger the degree of EIMD elicited by the initial exercise, stronger the effect (Chen et al., 2007). This effect is also observed when the initial exercise is concentric contraction (Nosaka and Newton, 2002a). The actual duration of the effect of concentric contraction remains unknown, but it is assumed that the duration of the adaptation after concentric exercise is shorter than that after eccentric exercise because the extent of the first EIMD affects the effect size of the next adaptation. Therefore, we asked the participants to perform eccentric elbow flexion exercises of at time points separated by >8 weeks following concentric condition.
Experiment protocolThe dependent variables included the maximum voluntary isometric contraction (MVIC) torque of the elbow flexions, ROM of the elbow joint, SOR, UTF concentration, and serum CK activity. The measurements of MVIC, ROM, SOR, and UTF concentration were obtained immediately before and after and 24, 48, 72, 96, 120, and 144 h after concentric and eccentric exercises (Lavender and Nosaka, 2006). Because serum CK activity barely change following concentric exercise (Lavender and Nosaka, 2006; Pedersen et al., 2001), serum CK activity was collected when the other dependent variables were measured only for the ECC condition. All dependent variables were measured by the same investigator to ensure that the results were consistent. Based on the intraclass correlation coefficient (R) and coefficient of variation (CV), the test–retest reliabilities of the study were 1.0 and 0.0% for SOR, 0.96 and 1.5% for ROM, 0.91 and 2.9% for MVIC, 0.99 and 3.4% for serum CK activity, and 0.94 and 4.5% for UTF, respectively.
Eccentric and concentric exerciseEach subject was seated on the chair of an isokinetic dynamometer (Biodex System 3, Biodex Medical Systems, Inc. Shirley, NY, USA), and the shoulder joint angle was set at 45° flexion with 0° abduction. The participants were asked to supinate their forearm and grasp the attachment connected to the lever arm of the dynamometer. In the concentric exercise task, the participants performed 3 sets of 10 repetitions with 60-s rest between the sets of elbow flexions. In the eccentric exercise task, the participants performed the same workload recorded during concentric exercise. We forcibly extended the elbow joint from a flexion (starting position: 90°) to an extension (end position: 180°) for over 3 s (Tsuchiya et al., 2018). To ensure that participants performed only the target actions, we set the dynamometer to conduct the flexion passively from the end position to start position for 3 s for ECC and vice versa for CON. We verbally encouraged participants during exercise to maximize their efforts. The peak torque and work of each contraction were calculated by the Biodex built-in software.
Maximal voluntary isometric contraction torqueThe MVIC torque of the elbow flexions was measured by the isokinetic dynamometer (Biodex System 3, Biodex Medical Systems, Inc. Shirley, NY, USA). We set up the isokinetic dynamometer in the same position for each subject, as described earlier for exercise. We recorded the positions of the dynamometer and each attachment on the first day of the experiment and measured the MVIC values of the participants in the same position on subsequent days. The participants performed three 5-s MVICs at an elbow joint angle of 110° with 15-s rest between contractions. The highest torque of the three contractions was used as the MVIC torque for further analysis (Yamaguchi et al. in press). The MVIC torque test was conducted by the same researcher each time to obtain reliable results.
Active range of motionA semi-permanent marker was used to label the center of the acromion, lateral epicondyle, and ulnar styloid. The elbow joint angle was photographed in a relaxed and flexed state to determine the active ROM, and the angle formed between the line connecting the center of the acromion and the lateral epicondyle and that connecting the lateral epicondylitis and the ulnar styloid was calculated by the ImageJ software (version 1.39, NIH, USA). After we determined the relaxed and flexed angle, we subtracted the relaxed angle from the flexed angle to determine the ROM of the elbow joint (Nosaka et al., 2001b; Tsuchiya et al., 2018).
Muscle sorenessWe also measured SOR when participants actively extended their arms. SOR was assessed using a 100-mm visual analog scale (VAS), on which 0 indicated no pain and 100 represented extreme pain. We asked the participants to hold their shoulder joints at a flexion angle of 90° and elbow joints at an extension of 180° and to mark their levels of perceived soreness on the VAS.
Blood sample analysisWe obtained approximately 5 ml of blood from the antecubital vein by the standard venipuncture technique using serum separation tubes. We left the serum separation tubes to clot at room temperature for 30 min and then centrifuged the serum at 3,000 ×g for 10 min. We removed serum samples and stored them at —80°C for later analyses. We estimated serum CK activities using an automated analyzer (Model 747-400, Hitachi, Tokyo, Japan). The normal range of serum CK activities are 35–175 IU/L (Gagliano et al., 2009; Baird et al., 2012).
Titin N-terminal fragment excretion assayApproximately 3 ml of urine was collected from each subject to measure UTF concentrations via an enzyme-linked immunosorbent assay (ELISA) system using a kit (Titin N-terminal Fragment Assay Kit, Immuno-Biological Laboratories Co. Ltd., Fujioka, Japan) in accordance with previous studies (Awano et al., 2018; Maruyama et al., 2016). Samples were stored at —20°C for later analyses. Thawed urine samples were diluted by 1:5–1:350 so that the diluted samples were in the linear detection range. Diluted samples and standard solutions were added to each antibody-coated well of 96-well microplates and then incubated the microplates at 37°C for 60 min. Subsequently, the microplates were washed four times with wash buffer, and labeled antibodies were added in each well; the microplates were incubated again at 37°C for 30 min. The tetramethylbenzidine solution was incubated at room temperature for 30 min after washing five times using wash buffer. As the final step of the ELISA procedure, the stop solutions were added to each well. The absorbance was measured using a microplate reader at a main wavelength of 450 nm and sub-wavelength of 650 nm (VERSAmax; Molecular Devices, Sunyvale, CA, USA). The UTF concentration was calculated using a linear regression model, and urinary creatinine levels were estimated using an automated analyzer (Bio Majesty JCA-BM8060, JEOL, Tokyo, Japan). The UTF values were normalized relative to urinary creatine (Cr) (each raw data point in urine/Cr concentration) (Maruyama et al., 2016). The normal range of UTF concentrations in the general population is 1.47–7.14 pmol/mg/dl (Maruyama et al., 2016).
Statistical analysisValues are expressed as means ± SD. The Shapiro–Wilk test was used for normal distribution analysis; we confirmed the normal distribution of MVIC, ROM, and SOR but not CK activities or UTF; t-tests were used to compare the baseline values for MVIC, ROM, and SOR before exercise, and the Mann–Whitney U test was used for assessing UTF concentrations. Change in time course of CK activity in eccentric condition was compared using the Friedman test. When the Friedman test results indicated a significant difference, Scheffe’s post hoc test was performed for comparing values at different time points. Mauchly’s sphericity test was used to check the homogeneity of covariance before ANOVA. This test indicated a non-normal distribution of the UTF data; therefore, we applied logarithmic transformation (log10) before analysis (Hody et al., 2013). The changes in the total work during exercise of each set were compared between the groups (ECC vs. CON) by two-way ANOVA with two factors (condition × set). The changes in SOR, MVIC, ROM, and UTF after exercise were compared between the groups (ECC vs. CON) by two-way ANOVA (SOR, MVIC, ROM, and UTF) with two factors (condition × time). If a significant interaction effect found [comparison of the total work, condition (2) × set (3); comparison of each muscle damage index, condition (2) × time (8)], a post hoc test was performed to identify the time points of significant difference between groups using the Bonferroni’s method. Effect sizes were determined for these differences, and significance was set at p < 0.05. All statistical analyses were conducted using the predictive analytics software (PASW) version 24.0 for Windows (SPSS Japan Inc., Tokyo, Japan).
Baseline measurementsNo significant differences were found in the following measured values between exercise types before exercise began: ROM (CON; 121.9 ± 7.8°, ECC; 122.3 ± 8.6°, p = 0.835), SOR (CON; 0.0 ± 0.0 mm, ECC; 0.0 ± 0.0 mm), MVIC (CON; 55.6 ± 7.9 N·m, ECC; 56.5 ± 7.5 N·m, p = 0.715), and UTF (CON; 1.63 ± 0.6 pmol/mg/dl, ECC; 1.91 ± 0.66 pmol/mg/dl, p = 0.087).
Concentric and eccentric exerciseThe total work performed in 10 contractions per set was not significantly different between the sets; the total work was recorded in the first set (CON; 512.2 J ± 109.6 J, ECC; 510.8 J ± 87.1 J), in the second set (CON; 417.4 J ± 86.7 J, ECC; 413.1 J ± 76.7 J), and in the third set (CON; 376.0 ± 91.6 J, ECC; 372.2 ± 83.3 J) (Figure 1A). The peak torque of the first set of ECC (58.4 ± 11.6 N·m) was significantly higher than that of CON (44.6 ± 8.5 N·m) (Figure 1B). The number of repetitions performed in all sets of ECC was significantly lower than that of CON (Figure 1B).
Change in time course and difference between conditions for each measurementFor all measurements, a condition × time interaction was observed (p < 0.001). The percentage changes in MVIC immediately following and 24, 48, 72, 96, 120, and 144 h after exercise were —32.4 ± 12.1, —37.3 ± 12.7, —28.5 ± 18.5, —22.0 ± 17.7, —13.0 ± 19.0, —11.0 ± 18.1, and —8.8 ± 17.5% after ECC and —19.2 ± 7.4, 1.5 ± 7.2, 0.2 ± 4.0, 5.0 ± 6.4, 7.6 ± 7.9, 4.6 ± 5.9, and 7.2 ± 6.5% after CON, respectively. MVIC was reduced immediately after and 24 h after exercise compared with the pre-exercise value after ECC (p = 0.01) and was lower after ECC than that after CON at 24 (p = 0.001), 48 (p = 0.012), 72 (p = 0.019), and 96 h (p = 0.048) after exercise (Figure 2A). The percentage changes in ROM immediately following and 24, 48, 72, 96, 120, and 144 h after exercise were —13.6 ± 5.8, —12.1 ± 5.8, —12.2 ± 5.5, —11.6 ± 8.1, —9.4 ± 8.2, —5.8 ± 7.5, and —5.0 ± 6.6% after ECC and —6.4 ± 4.5, —1.7 ± 2.4, —1.1 ± 2.0, —0.5 ± 3.2, —1.3 ± 2.9, —1.2 ± 2.3, and —1.2 ± 2.9% after CON, respectively. ROM was reduced immediately after and 24 and 48 h after exercise compared with the pre-exercise value after ECC (p < 0.05) and was lower after ECC than that after CON immediately following (p = 0.01), 24 (p = 0.003), 48 (p < 0.001), 72 (p = 0.001), and 96 h (p = 0.022) after exercise (Figure 2B). The percentage changes in SOR immediately fol-lowing and 24, 48, 72, 96, 120, and 144 h after exercise were 17.6 ± 18.4, 48.6 ± 16.6, 51.1 ± 19.5, 45.8 ± 23.1, 35.7 ± 23.8, 20.0 ± 16.8, and 14.4 ± 17.7% after ECC and 6.7 ± 9.7, 1.7 ± 3.3, 0.0 ± 0.0, 0.0 ± 0.0, 0.0 ± 0.0, 0.0 ± 0.0, and 0.0 ± 0.0% after CON, respectively. SOR was higher 24, 48, and 72 h after exercise than that before ECC (p < 0.05); it was higher after ECC than after CON at 24 (p < 0.001), 48 (p < 0.001), 72 (p = 0.001), 96 (p = 0.003), and 120 h (p = 0.01) after exercise (Figure 2C). The percentage changes in the UTF concentrations immediately following and 24, 48, 72, 96, 120, and 144 h after exercise were —1.91 ± 16.8, 172.4 ± 167.1, 1095.7 ± 1678.0, 3815.8 ± 6832.8, 5818.1 ± 12514.6, 4061.4 ± 7534.6, and 2708.9 ± 4778.3% after ECC and 39.5 ± 43.2, 31.1 ± 56.0, 6.7 ± 57.7, 18.0 ± 55.3, 26.6 ± 87.0, 2.5 ± 39.2, and 83.5 ± 220.1% after CON, respectively. The UTF concentration increased 72 and 96 h after exercise compared with that before ECC (p < 0.05) and was higher after ECC than that after CON at 24 (p = 0.012), 48 (p = 0.009), 72 (p = 0.001), 96 (p = 0.007), 120 (p = 0.005), and 144 h (p = 0.006) after exercise (Figure 3B).
Change in serum creatine kinase activity following eccentric exercisesThe changes in serum CK activities over time after ECC are shown in Figure 3A. The percentage changes in the serum CK activities immediately following and 24, 48, 72, 96, 120, and 144 h after exercise were 6.8 ± 5.5, 226.7 ± 359.1, 900.2 ± 1798.4, 2866.2 ± 6355.6, 5140.3 ± 11726.8, 4676.3 ± 11114.9, and 2417.9 ± 5630.6% after ECC. The serum CK activities was significantly different between different time points (p < 0.01), with post hoc tests indicating higher activities at 72, 96, 120, and 144 h after exercise than that before exercise (p < 0.03).
Peak titin N-terminal fragment concentrationA comparison of UTF concentrations during each exercise type at all time points (Figure 4) indicated that the UTF concentration was significantly higher after ECC (9.67–449.67 pmol/mg/dl) than that after CON (1.49–3.58 pmol/mg/dl) (p < 0.001).
The purpose of the present study was to clarify whether UTF specifically indicated EIMD by investigating UTF concentration after concentric or eccentric contraction exercises. Although both types of exercise were performed at the same workload (Figure 1), MVIC and ROM decreased and SOR and UTF concentrations increased significantly after ECC compared with those after CON (Figures 2-3). These results suggest that EIMD was induced by eccentric contraction, as shown in a previous study (Lavender and Nosaka, 2006), and UTF was specifically responsive to EIMD. Therefore, findings of the present study clarify that the titin N-terminal fragment, which is a muscle structural protein, leaks into urine after anaerobic eccentric exercise and not after concentric exercise. After ECC, MVIC decreased by 37.3%, ROM decreased by 12.2%, and SOR increased by 51.1% 24 h or later after exercise. After CON, MVIC increased by 0.2%, ROM decreased by 1.6%, and SOR increased by 1.7% (Figure 2-3). The changes in SOR for ECC and in MVIC, ROM, and SOR for CON were similar to those obtained by Lavender and Nosaka (2006). However, the rate of change in MVIC and displacement of ROM obtained after ECC were lower than those determined by Lavender and Nosaka (2006). Nonetheless, Tsuchiya et al. (2018) reported that the rate of change in MVIC and displacement of ROM 24 h after 30 maximal voluntary eccentric exercises at the elbow flexion were decreased by 29.2% and 12.9%, respectively. Thus, we assumed that the results for MVIC and ROM obtained here were within the ranges found by other researchers. Moreover, CK activity increased only 24-fold compared with the baseline after ECC in the present study, whereas it increased nearly 90-fold in previous studies (Lavender and Nosaka, 2006). Chen (2006) reported that CK activity can be classified into the following four reaction groups: low (<500 IU/L), medium (500–2000 IU/L), high (2000–10,000 IU/L), and higher responder (>10,000 IU/L). The results of the present study (3346 IU/L) would place CK activity in the high responder category, and the results of Lavender and Nosaka (10,601 IU/L) would place it in the higher responder category. Because CK activity in the present study was in the high responder category despite lower concentrations than those reported in previous studies (Lavender and Nosaka, 2006), the exercise intensity in the present study was considered sufficient to promote the onset of EIMD. The rise in UTF concentrations after ECC was significantly higher than that observed after CON (Figure 3B). To the best of our knowledge, these results are the first findings and UTF is a biomarker that can specifically detect EIMD. In the present study, UTF concentrations were significantly elevated 24 h after exercise, peaked after 96 h, and remained significantly higher than the pre-exercise value even after 144 h. Kanda et al. (2017) conducted a study in which calf raise exercises were performed and reported that UTF concentrations peaked 96 h after exercise, which is a result consistent with those of the present study. Conversely, Maruyama et al. (2016) conducted a study in which UTF concentrations were examined after 10 km of running; they reported that the peak was reached 9 h after exercise, and values recovered to pre-exercise levels 66 h after exercise. The difference between the two results may be owing to the difference in exercise mode adopted as a fatigue bout, and UTF concentrations after anaerobic exercise may peak later than aerobic exercise. Levels of blood biomarkers such as CK activity and Mb peak at 72–120 h after anaerobic exercise (Baird et al., 2012) and at 6 h after aerobic exercise such as uphill running, in which EIMD does not develop (Malm et al., 2004). The difference in these results is attributed to differences in the mechanism by which the target biochemical substance leaks from the muscle cells between anaerobic and aerobic exercise. Nosaka et al. (2002a; 2002b) reported that as a result of performing 3 sets of 10 eccentric contractions of the elbow flexions, which were 50% of the maximum isometric contraction of the elbow flexions, using a dumbbell on days 1, 3, and 5 for a week, serum CK activity significantly increased on day 3 and peaked on day 5 after the first exercise on day 1. The leakage of CK activity into the blood after anaerobic exercise was induced by disruptions in the contractile apparatus caused by mechanical and metabolic stress (Koch et al., 2014). The delayed elevation of serum CK activities is owing to the activation of muscle proteases by the increase in intramuscular Ca2+ concentrations during resting. The amount of CK activity leakage into the blood peaked at 5 days after eccentric exercise (Pandurangan and Hwang, 2012). Conversely, Totsuka et al. (2002) reported that as a result of performing 90 min of bicycle exercise for three consecutive days, serum CK activity significantly increased at 3 h and peaked at 3 days after the first aerobic exercise. While undergoing long-term aerobic exercise requiring a large amount of energy, muscle cells are temporarily filled with CK, and a concentration gradient is found between the inside and outside of the muscle cell. Because the concentration gradient promotes the permeability of the muscle cell membrane, CK leaks into the blood without damaging the membrane. Although the mechanism by which titin fragments and CK are generated is different, the leakage mechanism is expected to be similar as long as both of the biochemicals are present in muscle cells. According to Eckels et al. (2018), titin filaments act specifically during eccentric contraction and cooperate with actin and myosin filaments to generate great tension. However, it has been reported that titin, which activates the tension of eccentric contraction to complement actin and myosin filaments, is unfortunately degraded after intense eccentric contraction (Eckels et al., 2018). In a study in which muscle biopsies were performed before and after eccentric exercise and the content of titin was examined, titin reduced by 30% after exercise compared with that before exercise (Trappe et al., 2002). Furthermore, muscle biopsies were performed before and after eccentric exercise, and the structure of the muscle fibers was confirmed using an electron microscope. Z- and M-lines were largely streamed after exercise; the N-terminus of titin attached to M-line was dissociated, and the content of titin was reduced (Macaluso et al., 2014; Yu et al., 2003). The mechanism by which muscle fibers are destroyed is the degradation by proteases in addition to direct destruction by mechanical stress (Ono et al., 2016). Calpain 3, a Ca2+-dependent cysteine protease that functions in skeletal muscle specifically cleaves and degrades titin (Raastad et al., 2010) and is confirmed to be present in myocytes after eccentric exercise but is not confirmed to be present after concentric exercise (Ato et al., 2017; Ono et al., 2016; Vissing et al., 2008). Based on the above-mentioned studies (Ato et al., 2017; Ono et al., 2016; Vissing et al., 2008), as a mechanism by which titin is excreted in urine, titin is degraded by calpain 3 and released into urine via blood after eccentric contraction performed in the descending limb of the sliding filament theory; conversely, because calpain 3 is not expressed after concentric exercise, titin may not be degraded and released in urine. The present study has two limitations. The first is that the peak torque is different between the two types of exercise. In a present study, although the workload of the exercise task was controlled between the conditions (Figure 1A), a significant difference was confirmed in the peak torque of the first set (Figure 1B). Thus, the difference in peak torque during exercise probably influenced the difference between the conditions of all the measured values. However, when 100 repetitions of concentric exercise were performed with maximum effort, there was almost no change in the muscle strength and SOR (Bottas et al., 2005), but even if 30 repetitions of eccentric exercise were performed at 40% of the maximum torque, the MVIC decreases in approximately 20% and SOR increases in 25% 48 h after exercise (Chen et al., 2007). Therefore, because concentric contractions do not result in EIMD even if they are performed with maximum effort and high frequency and eccentric contractions occur at low effort and low frequency contraction, it is considered that the difference in the contraction mode influenced the onset of EIMD rather than the peak torque, as evidenced by the results of the present study. The second is that the peak UTF concentration clearly differs between the low and high responders (Figure 4). The peak UTF concentration after eccentric exercise obtained in the present study showed a large difference between low and high responders (7.43 vs. 449.70 pmol/mg/dl). Gender, age, muscle mass, genetic factors, ethnicity, contraction times, effort, intensity, number of contractions, muscle length, selected muscle groups, frequency of daily exposure to eccentric contractions, and training frequency can cause differences in the EIMD symptoms among individuals (Brancaccio et al., 2007; Hyldahl et al., 2017; Koch et al., 2014). In the present study, because all factors except for genetics and frequency of exposure to eccentric contractions on a daily basis are the same, the cause of the difference in UTF concentrations may be this one or both. The participants in this study performed limited resistance training for six months but had no control over the frequency of exposure to eccentric contractions on a daily basis. Furthermore, genetic differences among individuals affect the degree of EIMD (Pimenta et al., 2012). Therefore, future studies are required to determine the factors affecting UTF concentrations by examining the effects of daily exposure to eccentric contractions and the genetics of the individual participants.
In the present study, although eccentric exercise was performed as the same workload as the concentric condition, UTF concentrations increased only after eccentric contraction. This result suggests that UTF will be useful as a new biomarker for specific measurement of EIMD.
ACKNOWLEDGEMENTS |
We thank the participants who participated in this study. This work was supported from Yamaha Motor Foundation for Sports, a Waseda University Grant for Special Research Projects (Project number: 2018B-293), and JSPS KAKENHI Grant Number (Project number: 19K11452). The authors have no conflict of interest directly relevant to the content of the study. The authors declare the present study comply with the current laws of the country in which they were performed. |
|
AUTHOR BIOGRAPHY |
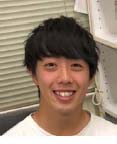 |
Shota Yamaguchi |
Employment: Graduate School of Sport Sciences, Waseda University, Saitama, Japan |
Degree: MSc |
Research interests: Exercise-induced muscle damage |
E-mail: y.shota-56@akane.waseda.jp |
|
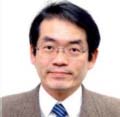 |
Katsuhiko Suzuki |
Employment: Faculty of Sport Sciences, Waseda University, Saitama, Japan |
Degree: MD, PhD |
Research interests: Exercise and Immunology |
E-mail: katsu.suzu@waseda.jp |
|
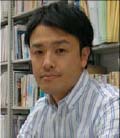 |
Takayuki Inami |
Employment: Institute of Physical Education, Keio University, Japan |
Degree: PhD |
Research interests: Muscle Biomechanics, Sports Medicine |
E-mail: inamit@keio.jp |
|
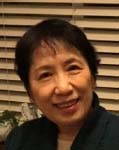 |
Kazue Kanda |
Employment: Institute for Nanoscience & Nanotechnology, Waseda University, Shinjuku, Tokyo, JAPAN |
Degree: PhD |
Research interests: Sports Science, Muscle Damage |
E-mail: rurishijimikanda@gmail.com |
|
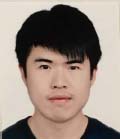 |
Zhao Hanye |
Employment: Graduate School of Sport Sciences, Waseda University. |
Degree: MSc |
Research interests: Muscular activation, training volume |
E-mail: zhaohanye@toki.waseda.jp |
|
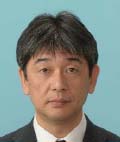 |
Junichi Okada |
Employment: Faculty of Sport Sciences, Waseda University, Japan |
Degree: PhD |
Research interests: Strength training and Weightlifting |
E-mail: jun1okd@waseda.jp |
|
|
|
REFERENCES |
 Ato S., Makanae Y., Kido K., Sase K., Yoshii N., Fujita S. (2017) The effect of different acute muscle contraction regimens on the expression of muscle proteolytic signaling proteins and genes. Physiological Reports 5, pii: e13364.
|
 Awano H., Matsumoto M., Nagai M., Shirakawa T., Maruyama N., Iijima K., Nabeshima Y.I., Matsuo M. (2018) Diagnostic and clinical significance of the titin fragment in urine of Duchenne muscular dystrophy patients. Clinica Chimica Acta 476, 111-116.
|
 Baird M.F., Graham S.M., Baker J.S., Bickerstaff G.F. (2012) Creatine-kinase- and exercise-related muscle damage implications for muscle performance and recovery. Journal of Nutrition and Metabolism 2012, 960363.
|
 Bottas R., Linnamo V., Nicol C., Komi P.V. (2005) Repeated maximal eccentric actions causes long-lasting disturbances in movement control. European Journal of Applied Physiology 94, 62-69.
|
 Brancaccio P., Maffulli N., Limongelli F.M. (2007) Creatine kinase monitoring in sport medicine. British Medical Bulletin 81-82, 209-230.
|
 Chen T.C. (2006) Variability in muscle damage after eccentric exercise and the repeated bout effect. Research Quarterly for Exercise and Sport 77, 362-371.
|
 Chen T.C., Chen H.L., Lin M.J., Wu C.J., Nosaka K. (2009) Muscle damage responses of the elbow flexors to four maximal eccentric exercise bouts performed every 4 weeks. European Journal of Applied Physiology 106, 267-275.
|
 Chen T.C., Nosaka K., Sacco P. (2007) Intensity of eccentric exercise, shift of optimum angle, and the magnitude of repeated-bout effect. Journal of Applied Physiology (1985) 102, 992-999.
|
 Clarkson P.M., Hubal M.J. (2002) Exercise-induced muscle damage in humans. American Journal of Physical Medicine & Rehabilitation 81, S52-S69.
|
 Cleak M.J., Eston R.G. (1992) Delayed onset muscle soreness: mechanisms and management. Journal of Sports Sciences 10, 325-341.
|
 Eckels E.C., Tapia-Rojo R., Rivas-Pardo J.A., Fernandez J.M. (2018) The work of titin protein folding as a major driver in muscle contraction. Annual Review of Physiology 80, 327-351.
|
 Gagliano M., Corona D., Giuffrida G., Giaquinta A., Tallarita T., Zerbo D., Sorbello M., Paratore A., Virgilio C., Cappellani A., Veroux P., Veroux M. (2009) Low-intensity body building exercise induced rhabdomyolysis: a case report. Cases Journal 2, 7.
|
 Herzog W., Leonard T.R., Joumaa V., Mehta A. (2008) Mysteries of muscle contraction. Journal of Applied Biomechanics 24, 1-13.
|
 Hody S., Rogister B., Leprince P., Wang F., Croisier J.L. (2013) Muscle fatigue experienced during maximal eccentric exercise is predictive of the plasma creatine kinase (CK) response. Scandinavian Journal of Medicine & Science in Sports 23, 501-507.
|
 Hyldahl R.D., Chen T.C., Nosaka K. (2017) Mechanisms and mediators of the skeletal muscle repeated bout effect. Exercise and Sport Sciences Reviews 45, 24-33.
|
 Kanda K., Sakuma J., Akimoto T., Kawakami Y., Suzuki K. (2017) Detection of titin fragments in urine in response to exercise-induced muscle damage. PLoS One 12, e0181623.
|
 Kanda K., Sugama K., Sakuma J., Kawakami Y., Suzuki K. (2014) Evaluation of serum leaking enzymes and investigation into new biomarkers for exercise-induced muscle damage. Exercise Immunology Review 20, 39-54.
|
 Kanehisa H., Miyashita M. (1983) Effect of isometric and isokinetic muscle training on static strength and dynamic power. European Journal of Applied Physiology and Occupational Physiology 50, 365-371.
|
 Khan M.A., Moiz J.A., Raza S., Verma S., Shareef M.Y., Anwer S., Alghadir A. (2016) Physical and balance performance following exercise induced muscle damage in male soccer players. Journal of Physical Therapy Science 28, 2942-2949.
|
 Koch A.J., Pereira R., Machado M. (2014) The creatine kinase response to resistance exercise. Journal of Musculoskeletal and Neuronal Interactions 14, 68-77.
|
 Lavender A.P., Nosaka K. (2006) Changes in fluctuation of isometric force following eccentric and concentric exercise of the elbow flexors. European Journal of Applied Physiology 96, 235-240.
|
 Macaluso F., Isaacs A.W., Di Felice V., Myburgh K.H. (2014) Acute change of titin at mid-sarcomere remains despite 8 wk of plyometric training. Journal of Applied Physiology (1985) 116, 1512-1519.
|
 Malm C., Sjodin T.L., Sjoberg B., Lenkei R., Renstrom P., Lundberg I.E., Ekblom B. (2004) Leukocytes, cytokines, growth factors and hormones in human skeletal muscle and blood after uphill or downhill running. Journal of Physiology 556, 983-1000.
|
 Maruyama N., Asai T., Abe C., Inada A., Kawauchi T., Miyashita K., Maeda M., Matsuo M., Nabeshima Y.I. (2016) Establishment of a highly sensitive sandwich ELISA for the N-terminal fragment of titin in urine. Scientific Reports 6, 39375.
|
 Newham D.J., Mills K.R., Quigley B.M., Edwards R.H. (1983) Pain and fatigue after concentric and eccentric muscle contractions. Clinical science (London) 64, 55-62.
|
 Newton M.J., Sacco P., Chapman D., Nosaka K. (2013) Do dominant and non-dominant arms respond similarly to maximal eccentric exercise of the elbow flexors?. Journal of Science and Medicine in Sport 16, 166-171.
|
 Nosaka K., Clarkson P.M. (1996) Changes in indicators of inflammation after eccentric exercise of the elbow flexors. Medicine and Science in Sports and Exercise 28, 953-961.
|
 Nosaka K., Newton M. (2002a) Concentric or eccentric training effect on eccentric exercise-induced muscle damage. Medicine & Science in Sports & Exercise 34, 63-69.
|
 Nosaka K., Newton M. (2002b) Repeated eccentric exercise bouts do not exacerbate muscle damage and repair. The Journal of Strength & Conditioning Research 16, 117-122.
|
 Nosaka K., Newton M.J., Sacco P. (2005) Attenuation of protective effect against eccentric exercise-induced muscle damage. Canadian Journal of Applied Physiology 30, 529-542.
|
 Nosaka K., Sakamoto K., Newton M., Sacco P. (2001a) The repeated bout effect of reduced-load eccentric exercise on elbow flexor muscle damage. European Journal of 85, 34-40.
|
 Nosaka K., Sakamoto K.E.I., Newton M., Sacco P. (2001b) How long does the protective effect on eccentric exercise-induced muscle damage last?. Medicine & Science in Sports & Exercise 33, 1490-1495.
|
 Ono Y., Ojima K., Shinkai-Ouchi F., Hata S., Sorimachi H. (2016) An eccentric calpain, CAPN3/p94/calpain-3. Biochimie 122, 169-187.
|
 Pandurangan M., Hwang I. (2012) The role of calpain in skeletal muscle. Animal Cells and Systems 16, 431-437.
|
 Pedersen B.K., Steensberg A., Fischer C., Keller C., Ostrowski K., Schjerling P. (2001) Exercise and cytokines with particular focus on muscle-derived IL-6. Exercise Immunology Review 7, 18-31.
|
 Pimenta E.M., Coelho D.B., Cruz I.R., Morandi R.F., Veneroso C.E., de Azambuja Pussieldi G., Carvalho M.R., Silami-Garcia E., De Paz, Fernandez J.A. (2012) The ACTN3 genotype in soccer players in response to acute eccentric training. European Journal of Applied Physiology 112, 1495-1503.
|
 Raastad T., Owe S.G., Paulsen G., Enns D., Overgaard K., Crameri R., Kiil S., Belcastro A., Bergersen L., Hallen J. (2010) Changes in calpain activity, muscle structure, and function after eccentric exercise. Medicine & Science in Sports & Exercise 42, 86-95.
|
 Rouillon J., Zocevic A., Leger T., Garcia C., Camadro J.M., Udd B., Wong B., Servais L., Voit T., Svinartchouk F. (2014) Proteomics profiling of urine reveals specific titin fragments as biomarkers of Duchenne muscular dystrophy. Neuromuscular Disorders 24, 563-573.
|
 Totsuka M., Nakaji S., Suzuki K., Sugawara K., Sato K. (2002) Break point of serum creatine kinase release after endurance exercise. Journal of Applied Physiology 93, 1280-1286.
|
 Trappe T.A., Carrithers J.A., White F., Lambert C.P., Evans W.J., Dennis R.A. (2002) Titin and nebulin content in human skeletal muscle following eccentric resistance exercise. Muscle & Nerve 25, 289-292.
|
 Tsuchiya Y., Nakazato K., Ochi E. (2018) Contralateral repeated bout effect after eccentric exercise on muscular activation. European Journal of Applied Physiology 118, 1997-2005.
|
 Vissing K., Overgaard K., Nedergaard A., Fredsted A., Schjerling P. (2008) Effects of concentric and repeated eccentric exercise on muscle damage and calpain-calpastatin gene expression in human skeletal muscle. European Journal of Applied Physiology 103, 323-332.
|
 Yamaguchi S., Suzuki K., Kanda K., Inami T., Okada J. () Change in urinary titin N-terminal fragment as a biomarker of exercise-induced muscle damage in the repeated bout effect. Journal of Science and Medicine in Sport.
|
 Yamaguchi S., Suzuki K., Kanda K., Okada J. (2020) N-terminal fragments of titin in urine as a biomarker for eccentric exercise-induced muscle damage. The Journal of Physical Fitness and Sports Medicine 9, 21-29.
|
 Yu J.G., Furst D.O., Thornell L.E. (2003) The mode of myofibril remodelling in human skeletal muscle affected by DOMS induced by eccentric contractions. Histochemistry and Cell Biology 119, 383-393.
|
|
|
|
|
|
|