|
|
|
ABSTRACT |
Various substances have been introduced in relation with cardiac hypertrophy almost always with controversy in their roles in signal transduction. Those controversies may attribute to the diversity of cardiac hypertrophy. We previously showed that calcineurin was activated in physiological left ventricular hypertrophy (LVH) induced by voluntary exercise training, but not in decompensated pressure-overload LVH. In the current study, we advanced our search for the differences between the voluntary exercise-induced LVH and the pressure-overload LVH into several other hypertrophy-related substances including caveolin. Wistar rats were assigned to one of the following three groups: 10 weeks of voluntary exercise (EX), sedentary regimen (SED), and 4 weeks of ascending aortic constriction (AC). The EX rats voluntarily ran 1.6 ± 1.1 km/day in the specially manufactured cages resulting in LVH (24 % increase in left ventricular weight per body weight ratio). Myocardial tissue homogenate of the EX rats revealed different characteristics in signal transduction of hypertrophy from that of the AC. The EX rats had normal sarcoplasmic reticulum (SR) Ca2+ATPase mRNA level and normal myosin heavy chain isozyme pattern assessed by RNA protection assay, while AC rats had decreased SR Ca2+ATPase mRNA level and increased beta myosin heavy chain mRNA level. Myocardial caveolin-3 protein levels assessed by Western blotting increased in the EX rats but decreased in the AC rats. The voluntary exercise-induced LVH differed in signal transduction from the decompensated pressure-overload LVH. Caveolin-3 was induced in the voluntary exercise-induced LVH, while it was decreased in the decompensated pressure-overload LVH. |
Key words:
Exercise, hypertrophy, signal transduction
|
Key
Points
|
Caveolin is a 21- to 24-kDa principal structural protein of caveolae, vesicular invagination of the plasma membrane (Lisanti et al., 1994). The mammalian caveolin family consists of caveolin-1, -2 and -3. Among them, caveolin-3 is muscle specific and found in skeletal, cardiac and smooth muscle (Song et al., 1996). Caveolin is known to be involved in cellular signaling cascades including G-proteins, sarcoplasmic reticulum (SR) kinase, nitric oxide (NO) synthase, epidermal growth factor, platelet-derived growth factor receptors and protein kinase C (Okamoto et al., 1998). Caveolin is also a substrate of the low M(r) protein-tyrosine phosphatase (Caselli et al., 2001). It is also shown that caveolin was down-regulated by chronic beta-adrenergic stimulation (Oka et al., 1997). Caveolin is related with hypertrophy of skeletal and cardiac muscles. Myocardial caveolin-3 expression was decreased in 24-week-old SHR compared with age-matched Wistar-Kyoto (WKY) rats and 4-week-old Spontaneously Hypertensive Rat (SHR) (Toya et al., 2001). Rat neonatal cardiomyocytes had diminished hypertrophic response to overexpressing caveolin phenylephrine (Oka et al., 2001). Transgenic overexpression of caveolin-3 in skeletal muscle induced a Duchenne-like muscular dystrophy in mice (Galbiati et al., 2000). On the contrary, loss of caveolin-3 expression resulted in another form of muscular abnormality, limb-girdle type muscular dystrophy (Minetti et al., 1998). These findings may indicate that adequate regulation of caveolin level may be necessary for the maintenance of normal muscle physiology. Caveolin may be an inhibitor of some types of cardiac hypertrophy, although the specific functions of caveolin is unknown. The role of caveolin in adaptive physiological hypertrophy is unknown. Caveolin may possibly be involved in the physiological hypertrophy signaling and may decrease with transition into decompensated hypertrophy or cardiac failure. The specific purpose of this study was to compare the signal transduction, including caveolin, of cardiac hypertrophy between the hearts from rats with voluntary exercise-induced physiological left ventricular hypertrophy (LVH) and those from rats with aortic constriction-induced pathological LVH.
Group AssignmentsAll the animal experiments were approved by the Animal Experimentation Review Board of the University of Tokyo. Male Wistar rats were assigned to 1 of the following 3 groups: (1) 10-week voluntary exercise training (EX, n=9), (2) sedentary condition (SED, n=9), and (3) 4-week ascending aortic constriction (AC, n=9).
Voluntary Exercise-Induced Physiological LVH (Eto et al., )Four-week-old rats transferred to our laboratory was randomly assigned into the voluntary exercise group and sedentary group. Ones in the voluntary exercise group were individually housed in the specially manufactured cage equipped with a controlled running wheel and a distance counter. We controlled the torque of the running wheel, not the rotation speed. Therefore, what we measured as the distance was a daily work rather than the exercise intensity. Rats exercised at their favourite time, at a speed and duration of their choice (EX). Age-matched sedentary control rats were also individually housed in ordinary cages (SED). Ten weeks later, the hearts were excised and the following biochemical analyses were performed.
Pressure-Overload Induced Pathological LVHTen-week-old rats were anesthetized with intraperitoneal injection of sodium pentobarbital (50 mg·kg-1). A ligature was placed around the ascending aorta, and an 18-gauge needle was set parallel to it. Then, the needle was quickly removed, leaving the ascending aorta constricted to the diameter of the needle (Aoyagi et al., 1999). Four weeks later, the biochemical assessments were performed.
RNA Protection ActivityTotal RNA was extracted from the left ventricular tissue with TRIZOL (GIBCO, Carlsbad, CA), and used in the following RNase protection assays with probes for alpha- and beta-myosin heavy chain (MyHC), sarcoplasmic reticulum Ca2+ ATPase (SERCA2a), atrial-type natriuretic peptide (ANP), brain-type natriuretic peptide (BNP), alpha-skeletal and alpha-cardiac actins, and glyceraldehyde 3-phosphate dehydrogenase (GAPDH) as an internal control. Pathological examination in our previous study (Eto et al., 2000) revealed no significant fibrosis in either of the LVH models. Therefore, the left ventricular crude homogenates for the RNase protection assay and the Western blot were considered to consist of mainly cardiac myocytes. All plasmids for riboprobes were sequenced, and linearized at the 3’-end of the antisense strand with appropriate restriction enzymes. The StyI-digested rat GAPDH probe (pTRI-GAPDH-Rat, Ambion) protected a 134-bp fragment (complimentary to nucleotides 369-503 of Accession number X002310). The probe specific for the rat SERCA2a gene protected a 333-bp fragment of SERCA2a (3014-3347 nucleotides of X15635). The probe for rat MyHC was complimentary to the 3’-end of beta-MyHC mRNA; it protected a 300-bp fragment (5626-5925 nucleotides of X15939), but also hybridized to alpha-MyHC, and protected a 175-bp fragment (5656-5830 nucleotides of X15938). The probes specific for the rat ANP and BNP genes protected a 212-bp fragment and a 183-bp fragment of ANP (96-307 nucleotides of E00443) and BNP (246-428 nucleotides of M25297), respectively. The probes specific for the rat alpha-skeletal actin and alpha-cardiac actin genes protected a 195-bp fragment and a 100-bp fragment of alpha skeletal actin (2841-3037 nucleotides of V01218) and alpha cardiac actin (1111-1210 nucleotides of X80130), respectively. Riboprobes were labeled with [alpha-32P] UTP using Maxiscript kit (Ambion, Austin, TX). The MyHC and the SERCA2a probes had a specific activity of ~0.5x108 and ~1.0x108 cpm/µg, respectively, and were used with 3 µg total RNA. The GAPDH probe (specific activity; ~2x108 cpm/µg) was mixed in each sample as an internal control. After incubation at 42°C overnight, the RNA-probe mix was digested with RNase A/T1 (1:100, RPAII, Ambion, Austin, TX) at 37°C for 30 min, and protected fragments were separated on a 6% denaturing polyacrylamide gel. Radioactivity of each band was quantified by densitometry. Signals were corrected for background and were normalized to GAPDH levels.
Western Blot Analysis for Myocardial Caveolin ContentPreparation of tissue extracts was performed as previously described (Yonekura et al., 2000). Briefly, hearts were homogenized in a buffer containing 50mM Tris-HCl (pH6.8), 2% sodium dodecyl sulfate (SDS), 6% 2-Mercaptoethanol, 10% glycerol, and protease inhibitors, and separated by 15% SDS-polyacrylamide gel. The proteins were then transferred onto poly vinylidene difluoride (PVDF) membranes. The membranes were incubated in tris-saline buffer (TBS) containing 0.1% Tween-20, 5% non-fat dry milk, 5% bovine serum albumin for 1 hour at room temperature. The membranes were then treated with each primary antibody (anti-caveolin-1, anti-caveolin-2, and anti-caveolin-3 monoclonal antibodies (Transduction Laboratories, Lexington, KY)) for two hours followed by washing and incubation with a secondary antibody. The signals were detected by SuperSignal West Dura Extended Duration Substrate (Pierce, Rockford, IL), exposed to Hyperfilm electrochemiluminescence (ECL) (Amersham Pharmacia Biotech, Buckinghamshire, UK), and quantitated by a densitometer.
Statistical AnalysesDifferences among the groups were compared by non-repeated measures ANOVA and Student-Newman-Keuls test. Data were expressed as the mean (SD). P < 0.05 was considered significant.
The EX rats ran 1.6 (1.1) km·day-1, and developed LVH. LV weight (LVW) and the LVW to body weight ratio (LVW·BW-1) increased in The EX rats by 15% (p < 0.05) and 24 % (p < 0.05), respectively, compared with the SED rats. The LVW and LVW·BW-1 increased in the AC rats by 30 % (p < 0.01) and 23 % (p < 0.01), respectively (Table 1).
Myocardial Gene Expression Regarding Hypertrophy Signaling ()The ascending aortic constriction surgery induced the myocardial expressions of alpha skeletal actin and natriuretic peptides, while SERCA2a mRNA level was decreased by 29% (p < 0.05). In contrast, the voluntary exercise training induced isozyme shift of MyHC from beta subtype to alpha subtype. SERCA2a mRNA level was increased by 50% by the exercise training. Neither ANP nor BNP was elevated in the EX group.
Myocardial Caveolin Content ()The amount of caveolin-3 expression in the EX group was approximately 11% higher than that in the control group (p < 0.05). In contrast, the amount of caveolin-3 expression of the AC group was approximately 13% lower than that in the SED group (p < 0.05). That is, caveolin-3 is up-regulated in physiological cardiac hypertrophy, but down-regulated in pathological cardiac hypertrophy. Caveolin-1 and caveolin-2 protein levels did not differ among the groups.
Left ventricular hypertrophy (LVH) induced by pathological hemodynamic overload can eventually result in maladaptive congestive heart failure (CHF). LVH is a strong predictor of subsequent cardiovascular events (Devereux et al., 1995). In contrast, the athletes’ heart, or LVH without pathological change, results from properly designed endurance exercise training (Barry, 1986). Elucidating the underlying molecular mechanisms that distinguish adaptive hypertrophy from pathological hypertrophy is important for the development of therapies to prevent CHF. We previously showed that calcineurin was activated in the voluntary exercise-induced physiological LVH but not in the decompensated pressure-overload hypertrophy (Eto et al., 2000). The LVW/BW, LV end-diastolic dimension, and velocity-time integral of aortic ejection flow increased, without an increase in cardiomyocytes width as reported in literature (Schaible et al., 1987, Moore et al., 1993). Other than calcineurin, many molecules participating in signal transduction of cardiac hypertrophy have been reported with controversies in their roles. Those controversies may come from the diversity of cardiac hypertrophy itself. We believe that the presence of adaptive physiological hypertrophy has different signal transduction from the pathological hypertrophy. In order to further clarify the characteristics of the voluntary exercise-induced physiological LVH, we advanced our investigation into several other molecules participating in signal transduction of cardiac hypertrophy including caveolin. The roles of caveolin in cardiac hypertrophy process are also controversial (Woodman et al., 2002, Galbiati et al., 2000, Minetti et al., 1998). The current study is the first report that shows the involvement of caveolin in physiological adaptive hypertrophy. Although roles of caveolin-3 in physiological cardiac hypertrophy are unknown, association of caveolin-3 with Na+/Ca2+ exchanger (Bossoyt et al., 2002) may somehow explain it. Since we classified the voluntary exercise as physiological response, the forced exercise would be a more pure counterpart. However, the forced running in rats have not successfully attained enough degrees of running distance on resultant hypertrophy corresponding to those of the current voluntary running model. Improvement in experimental design of forced running is in our future plan. The exercise rats voluntarily ran 1.6 ± 1.1km/day. The variation of the exercise amount among the animals was quite large. Some rats seemed to like physical exercise and our running wheel, while other did not. Despite this wide variation in the exercise amount, ventricular hypertrophy response was relatively steady. Effects of physical exercise may have on upper threshold, above which no more hypertrophic stimulus occurres. If this is the care, the dose-response relationship may not be linear. Other possible explanations in dude negative effects of sedentary circumstance on cardiac growth, which may be released in the voluntary exercise group.
The voluntary exercise-induced LVH differed in signal transduction from the decompensated pressure-overload LVH. Caveolin-3 was induced in the voluntary exercise-induced LVH, while it was decreased in the decompensated pressure-overload LVH.
|
AUTHOR BIOGRAPHY |
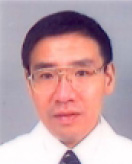 |
Teruhiko Aoyagi |
Employment: Japanese Red Cross Medical Center |
Degree: M.D. |
Research interests: Cardiology |
E-mail: aoyagi@ve.catv.ne.jp |
|
 |
Yoshihiro Ishikawa |
Employment: |
Degree: |
Research interests: |
E-mail: |
|
 |
Hitosh Oshikawa |
Employment: |
Degree: |
Research interests: |
E-mail: |
|
 |
Koichiro Kinugawa |
Employment: |
Degree: |
Research interests: |
E-mail: |
|
 |
Ikuo Yokoyama |
Employment: |
Degree: |
Research interests: |
E-mail: |
|
 |
Ryozo Nagai |
Employment: |
Degree: |
Research interests: |
E-mail: |
|
|
|
REFERENCES |
 Aoyagi T., Yonekura K., Eto Y., Matsumoto A., Yokoyama I., Sugiura S., Momomura S., Hirata Y., Baker D.L., Periasamy M. (1999) The sarcoplasmic reticulum Ca-ATPase (SERCA2) gene promoter activity is in response to severe left ventricular pressure-overload hypertrophy in rat hearts. Journal of Molecular and Cellular Cardiology 31, 919-926.
|
 Barry J.M. (1986) Structural features of the athlete heart as defined by echocardiography. Journal of American College of Cardiology 7, 190-203.
|
 Bossuyt J., Taylor B.E., James-Kracke M., Hale C.C. (2002) Evidence for cardiac sodium-calcium exchanger association with caveolin-3. FEBS Letter 511, 113-117.
|
 Caselli A., Taddei M.L., Manao G., Camici G., Ramponi G. (2001) Tyrosine-phosphorylated caveolin is a physiological substrate of the low M(r) protein-tyrosine phosphatase. Journal of Biological Chemistry 276, 18849-18854.
|
 Devereux R.B. (1995) Left ventricular geometry, pathophysiology, and prognosis. Journal of American College of Cardiology 25, 885-887.
|
 Eto Y., Yonekura K., Sonoda M., Arai N., Sata M., Sugiura S., Takenaka K., Gualberto A., Hixon M.L., Wagner M.W., Aoyagi T. (2000) Calcineurin is activated in rat hearts with physiological left ventricular hypertrophy induced by voluntary exercise training. Circulation 101, 2134-2137.
|
 Galbiati F., Volonte D., Chu J.B., Maomi L., Fine S.W., Fu M., Bermudez J., Pedemonte M., Weidenheim K.M., Pestell R.G., Minetti C., Lisanti M.P. (2000) Transgenic overexpression of caveolin-3 in skeletal muscle fiber induces a Duchenne-like muscular dystrophy phenotype. Proceedings of National Academy of Science 97, 9689-9694.
|
 Lisanti M.P., Scherer P.E., Tang Z., Sargiacomo M. (1994) Caveolae, caveolin and caveolin-rich membrane domains: a signaling hypothesis. Trends in Cellular Biology 4, 231-235.
|
 Minetti C., Sotgia F., Bruno C., Scartezzini P., Broda P., Bado M., Masetti E., Mazzocco P., Egeo A., Donati M.A. (1998) Mutations in the caveolin-3 gene cause autosomal dominant limb-girdle muscular dystrophy. Nature Genetics 18, 365-368.
|
 Moore R.L., Musch T.I., Yelamarty R.V. (1993) Chronic exercise alters contractility and morphology of isolated rat cardiac myocytes. American Journal of Physiology 264, C1180-C1189.
|
 Oka N., Asai K., Kudej R.K., Edwards J.G., Toya Y., Schwencke C., Vatner D.E., Vatner S.F., Ishikawa Y. (1997) Downregulation of caveolin by chronic beta-adrenergic receptor stimulation in mice. American Journal of Physiology 273, C1957-1962.
|
 Oka N., Kikuchi T., Nakaura H., Shimada H., Kato S., Imaizumi T. (2001) Adenovirus-mediated overexpression of caveolin-3 alters hypertrophic response to phenylephrine in rat cardiomyocytes (abstract). Japanese Circulation Journal 64, 583-.
|
 Okamoto T., Schlegel A., Scherer P.E., Lisanti M.P. (1998) Caveolins, a family of scaffolding proteins for organizing “preassembled signaling complexes ”at the plasma membrane. Journal of Biological Chemistry 273, 5419-5422.
|
 Schaible T., Malhotra A., Ciambrone G. () Combined effects of hypertension and chronic running program on rat heart. Journal of Applied Physiology 63, 322-327.
|
 Song K.S., Scherer P.E., Tang Z.L., Okamoto T., Li S., Chafel M., Chu C., Kohtz D.S., Lisanti M.P. (1996) Expression of caveolin-3 in skeletal, cardiac, and smooth muscle cells. Caveolin-3 is a component of the sarcolemma and co-fractionates with dystrophin and dystrophin-associated glycoproteins. J Biol Chem 271, 15160-15165.
|
 Toya Y., Iwatsubo K., Onda T., Kimura K., Umemura, S, Ishikawa Y. (2001) Accumulation of molecules involved in alpha-1 adrenergic signal within caveolae. (abstract) Japanese Circulation Journal 65, 42-.
|
 Woodman S.E., Park D.S., Cohen A.W., Cheung M.W., Chandra M., Shirani J., Tang B., Jelicks L.A., Kitsis R.N., Christ G.J., Factor S.M., Tanowitz H.B., Lisanti M.P. (2002) Caveolin-3 knock-out mice develop a progressive cardiomyopathy and show hyperactivation of the p42/44 MAPK cascade. Journal of Biological Chemistry 277, 38988-38997.
|
 Yonekura K., Eto Y., Yokoyama I., Matsumoto A., Sugiura S., Momomura S., Kirimoto T., Hayashi Y., Omata M., Aoyagi T. (2000) Inhibition of carnitine synthesis modulates protein contents of the cardiac sarcoplasmic reticulum Ca-ATPase and hexokinase type I in rat hearts with myocardial infarction. Basic Research in Cardiology 95, 343-348.
|
|
|
|
|
|
|