|
|
|
ABSTRACT |
The purpose of this study was to compare three different intervals for a between sets rest period during a common isokinetic knee extension strength-testing protocol of twenty older Brazilian men (66.30 ± 3.92 yrs). The volunteers underwent unilateral knee extension (Biodex System 3) testing to determine their individual isokinetic peak torque at 60, 90, and 120° ·s-1. The contraction speeds and the rest periods between sets (30, 60 and 90 s) were randomly performed in three different days with a minimum rest period of 48 hours. Significant differences between and within sets were analyzed using a One Way Analysis of Variance (ANOVA) with repeated measures. Although, at angular velocity of 60°·s-1 produced a higher peak torque, there were no significant differences in peak torque among any of the rest periods. Likewise, there were no significant differences between mean peak torque among all resting periods (30, 60 and 90s) at angular velocities of 90 and 120°·s-1. The results showed that during a common isokinetic strength testing protocol a between set rest period of at least 30 s is sufficient for recovery before the next test set in older men. |
Key words:
Aging, muscle strength, muscle fatigue, isokinetic test
|
Key
Points
- Muscle fiber cross sectional area (CSA ) decreased 38% following spinal cord injury (SCI).
- Early intervention with functional electric stimulation cycle ergometry (FES-CE) prevented further loss of CSA in SCI patients and increased power output.
- Muscle myosin heavy chain (MHC) and myonuclear density were unaffected by SCI or FES-CE
|
The functional consequence is of Spinal Cord Injury (SCI) are associated with changes in the physiologic, histologic, and morphologic properties of the affected skeletal muscle. Changes include muscle atrophy, decreased muscle fiber cross sectional area (CSAf), decreased force of contraction in response to electrical stimulation, decreased oxidative enzymes, decreased fatigue resistance and a transformation of Type I, slow-twitch muscle fiber to Type IIx fast-twitch muscle fibers (Baldi et al., 1998; Burnham et al., 1997; Castro et al.,1999). It has been reported that CSAf decreases more than 60% by 24 weeks post-injury (Castro et al., 1999) with a 21% decrease in lower extremity lean body mass 36 weeks post injury (Baldi et al. 1998). Transition from Type I to Type IIx muscle fibers appears to begin 24 weeks post-injury with the initial changes being from Type IIa to IIx (Castro et al. 1999) with a complete transition occurring one to two years post injury (Burnham et al. 1997). Remodeling of muscle to faster isoforms has also been demonstrated in a number of other disuse models such as spinal contusion (Hutchinson et al., 2001) and short-term space flight (Day et al., 1995). The shift to faster muscle isoforms may have some effect to conserve power output by shifting the force-velocity curve (Faulkner et al., 1995), however, when normalized to fiber area (N·cm-2), force production is reportedly not affected by fiber type (Brooks and Faulkner, 1988). Therefore, decreased force and power following SCI appears primarily to be due to decreased CSAf , while a shift towards Type II isoforms would decrease fatigue resistance of muscle. One modality used to try to attenuate or reverse changes in skeletal muscle as a result of SCI is electrical stimulation, however to date the affects have been modest. In chronic SCI survivors, more than 2 years post-injury, exposure to prolonged low frequency electrical stimulation resulted in a reversal of Type II muscle fibers towards Type I muscle fibers and no change in CSAf (Martin et al., 1992). Other researchers reported increased fatigue resistance and an improvement in oxidative capacity (Rochester et al., 1995a; 1995b) with similar protocols. Other more modest low frequency stimulation protocols documented an increase in fatigue resistance, no change in MHC isoform content, but in situ hybridization documented that mRNA for the Type I MHC isoforms were up regulated and the Type IIx MHC isoforms were down regulated (Harridge et al., 2002). Protocols utilizing functional electrical stimulation cycle ergometry (FES-CE) to train chronic SCI survivors have been documented to alter the effects of SCI on muscle morphology and histology. An FES-CE protocol of one year duration reported a significant shift in MHC composition from an overwhelming predominance of Type IIb to a predominance of Type IIa fibers with no discernable change in Type I MHC isoforms (Mohr et al., 1997). Others have reported an increase in muscle mass (Mohr et al., 1997; Scremin et al., 1999) and a modest increase in CSAf (Chilinbeck et al., 1999). Significant improvement in fatigue resistance (Gerrits et al., 2000) and power output (Faghri et al., 1992; Hooker et al., 1992) have also been reported in similar studies using FES-CE training. One study trained acute SCI (14- 15 weeks post- SCI) using FES-CE, reported prevention of the loss of lean body mass after 3 month of training and hypertrophy of leg muscles after 6 months of training (Baldi et al., 1998). These data then support the potential for electrical stimulation to reverse the plasticity observed in skeletal muscle after SCI and thereby improve the strength and endurance properties of that muscle for potentially functional purposes. The magnitude of the strength and endurance changes in response to electrical stimulation of chronic SCI survivors have been relatively modest despite the utilization of varied stimulation protocols and parameters under isometric contraction, contraction against no load or FES-CE conditions. Previous work in our laboratory (Baldi et al., 1998) demonstrated attenuation of loss in lean body mass muscle mass when FES-CE training was initiated within weeks of SCI. However, conservation of muscular power or changes to CSAf or fiber type in response to early intervention with FES-CE is unknown. Therefore, the primary purpose of this study was to determine if intervening 4 to 6 weeks post injury using FES-CE can attenuate the change in muscle fiber cross sectional area and MHC composition that is otherwise observed in response to SCI. It was hypothesized that 13 weeks of FES-CE training starting 4 to 6 weeks post injury would increase average cyclic power output and attenuate decreases in CSAf seen post SCI.
SubjectsA total of ten SCI subjects participated in this study and were recruited from the acute spinal cord injury (SCI) in-patient rehabilitation center. Subject demographic information can be found in Table 1. Criteria for selection were cervical or thoracic motor complete SCI (American Spinal Injury Association [ASIA] A or B) individuals 17-50 years of age (Maynard et al., 1997). All subjects were clinically stable before entering the study and continued their normal rehabilitation programs throughout the period of the study. Subjects were required to be able to sit upright for greater than thirty minutes before they were allowed to start training. Exclusion criteria include the presence of pressure ulcers, recurrent urinary tract infection, and transportation problems, prescribed medications that directly affect bone and muscle metabolism, lower extremity fracture at the time of the SCI, prolonged bleeding times, full dose anticoagulation, a history of clotting abnormalities, or known bleeding diathesis. All prospective subjects were given a thorough neurological and physiological exam including range of motion of lower limbs, resting electrocardiograph, and X-rays of lower extremities before participating in the study. Subjects were randomly assigned to either SCI-control (IC) or SCI- Functional Electric Stimulation Cycle Ergometry (FES-CE) (IE) groups. Non-injured age and gender-matched individuals were recruited from the faculty and student population of the Ohio State University to serve as uninjured controls (UIC). Following explanation of the procedures; purposes, benefits and risks of the study each subject provided written informed consent. This study was approved by the Institutional Review Board.
Equipment and training protocolThe FES-cycle ergometer training system is composed of a Stimaster Clinical Ergometry system (Electrologic of America, Inc., Dayton, OH), which was connected to an electrically braked cycle ergometer (Therapeutic Alliance, Inc., Fairborn, OH). As described previously (Baldi et al. 1998) training sessions were held 30 minutes·day-1, 3 days·week-1 for 13 weeks. Before each FES-CE training session 12 reusable electrodes were placed over appropriate motor points on the quadriceps, hamstring, and gluteal muscle groups. Each training session began with a one-minute warm- up, during which stimulation was delivered to all muscles at 50% of the threshold value (the minimum required to produce a palpable, tetanic contraction). Pedaling motion was assisted by an able-bodied operator throughout the warm-up and for the first 10-30 seconds of full stimulation, until the subject’s legs created enough power to maintain the cycling motion independently. All subjects began at 2 watts with the goal of completing 30 minutes of continuous cycling. As subject’s legs fatigued, and after delivered stimulation increased to the maximum (140 mA), pedaling cadence would slow. The stimulator is programmed to terminate the stimulation once cadence decreased to 35 rpm. Post ride, each subject went through a two-minute passive pedaling cool-down. Upon completion of 3 consecutive 30-minute training sessions, subjects’ resistance was increased by 6.1 watts. Subjects that were unable to ride at 2.4 watts for any length of time were trained to increase their quadriceps strength by performing electrically stimulated knee extensions. The leg was stimulated for 5 seconds with a 30 seconds rest before the next leg extension. When the subject was able to perform 30 repetitions of 45° knee extension with 1 kg, they were placed on the cycle ergometer and followed the training regimen as described above.
Functional changesFunctional changes in power output of the IE group were determined by changes in average weekly power output during FES-CE training. Average weekly power output was determined from the average power output of each ride as calculated by the Stimaster Clinical Ergometry system and the number of training sessions per week.
Tissue CollectionNeedle biopsies of the vastus lateralis (VL) muscle were taken initially 4-6 weeks post-SCI and after 13 weeks of training. Initial biopsies in SCI subjects varied between 4 and 6 weeks post-injury when subjects could sit upright for 30 minutes without orthostatic hypotension. Biopsies were taken from the non-injured control subjects 13 weeks apart. Biopsies for all subjects were taken from the non-dominant leg with a 25-gauge biopsy needle. Tissue was rapidly coated with Tissue-Tek OCT compound (Fisher 15-183-13), placed in a microcentrifuge tube, rapidly frozen in liquid nitrogen and stored at -80 °C for subsequent analysis.
Muscle histology and morphologyTen-micrometer serial cross-sections were cut from the muscle biopsy sample using a Reichert Histostat Microtome cryostat (-20° C), placed onto coverslips and stained using hematoxylin and eosin for identification of nuclear density and muscle fiber cross sectional area (CSAf) (Luna 1968). Slides were viewed under a Leitz Weitcher microscope equipped with a top mounted video camera. Images were sent to a Pentium 1 computer with Bioquant Classic 95 software package (R&M Biometrics, Inc.) for analysis. Fiber CSA was determined by calculating the CSA of 100 fibers using computer software (Bioquant 95, R&B Biometrics, Inc.). The muscle fiber cross-sections were captured and traced on a video monitor using a hand held mouse. The software was calibrated to determine the area in µm2. Average fiber CSA was determined for each muscle from the mean of 100 fibers quantified for each muscle. To reduce experimental bias in the selection of fibers for measurement, all of the fibers on randomly selected slides were quantified. Quantification of CSAf was practiced until a coefficient of variance of less than 5% was repeatedly achieved (See Figure 1). Myonuclear density was defined as the number of myonuclei·mm-2 of CSA and was determined by counting the number of nuclei in a set area and dividing the number by the area in square millimeters (Allen et al., 1997). Five counts were taken from different regions of each muscle section and the average of these values was used to determine nuclear density. To reduce experimental bias in the selection of nuclei for measurement, the slide was randomly selected for performing myonuclei counting. Myosin heavy chain (MHC) composition was determined using sodium dodecyl sulfate polyacrylamide gel electrophoresis (SDS-PAGE) as described previously (Talmadge and Roy, 1993). The SDS-PAGE gels were stained with rapid coomassie blue and scanned with a Pharmacia LKB Ultrascan laser scanning densitometer for quantification of MHC composition (See Figure 2).
Statistical analysesA one-way ANOVA with repeated measures was used to determine changes in functional power output for the SCI exercise group. A two-way ANOVA was used for statistical analysis of nuclear density, fiber CSA, and changes in MHC composition. Level of significance was set a priori at p < 0.05. The Student-Newman- Keul method was used to determine specifically where the significant difference occurred.
Functional changesAt the onset of training, subjects average power output was 2.4 ± 0.88 watts (mean ± SEM). Average weekly power output increased in the IE group by week four. Power output continued to increase with training and reached a maximum of 24.5 ± 3.2 watts (Figure 3).
Muscle morphologyThere was no difference in CSAf between SCI groups at time of initial biopsy. However, compared with uninjured control subjects, SCI resulted in a 36 % decrease in CSAf in both injured groups (Figure 4; p = 0.009). There was a continued decrease (72%) from initial biopsy after 13 weeks in the IC subjects (p < 0.001). In contrast, FES-CE training increased CSAf 63% in IE subjects following 13 weeks of training (3376 ± 625 vs. 5518 ± 1205 µm2). However, the difference was not significant (p = 0.172). After 13 weeks of FES-CE training the CSAf of the IE groups was 171% greater than the CSAf in the IC group (p = 0.05). There was no significant difference in nuclear density in SCI subjects compared to UIC at baseline. In addition FES-CE had no effect on nuclear density (Figure 5). Similarly, MHC composition of the muscle was unaffected by SCI and FES-CE (Table 2).
There is a paucity of data related to understanding the training consequences of functional electric stimulation cycle ergometry (FES-CE) on the maintenance of skeletal muscle form, structure, and function after acute spinal cord injury (SCI). To date, the effect of FES-CE in acute SCI subjects has been limited to measures of lean body mass and not focused on potential alterations to the morphological and physiological characteristics of skeletal muscle. Therefore, the primary aim of the present study was to determine the affect of FES-CE on muscle fiber cross-sectional area (CSAf), as well as to characterize changes in myosin heavy chain (MHC) composition, myonuclear density, and power output on a bicycle ergometer in acute SCI participants. Previous studies utilizing FES-CE training on chronic SCI survivors have reported increases in power output, defined as force input of a particular skeletal muscle or muscle group multiplied by velocity, that range from 5.1-17.1 watts following 8 -52 weeks of FES-CE training (Chilinbeck et al., 1999; Faghri et al., 1992; Hooker et al., 1992; Mohr et al., 1997; Scremin et al., 1999). In contrast, data from the present study demonstrate that the weekly power output averaged 24.5 ± 3.2 watts, which was achieved with 13 weeks of FES-CE training. Thus, the increase in power output found in the present study is substantially greater than seen in previous studies using chronic SCI subjects, suggesting that early intervention utilizing FES-CE training more effectively conserves power output when compared with other electrical stimulation training protocols using chronic SCI patients. A potentially salient adaptation known to result in an increase in power output is an increase in force development and, force production of a given muscle is directly related to its cross-sectional area (CSA). Previous work in chronic SCI subjects indicated the increases in power output were due to increases in either whole muscle CSA (Mohr et al., 1997; Scremin et al., 1999) or CSAf (Chilinbeck et al., 1999). In the present study, there was a 3 times greater increase in power output than reported by Mohr et al. (1997) suggesting that the more substantial attenuation of muscle atrophy observed in this study as a result of the acute intervention, contributed to diminishing the loss of power output (Figure 3). In the present study, the CSAf in SCI subjects decreased by 36% by 4-6 weeks post-injury, and 72% at 17-19 weeks post-injury relative to uninjured control subjects. These data are in close agreement with those of Castro et al. (1999) who reported decreases in CSAf of 37% and 62% 6 and 24 weeks post-injury, respectively. Previous reports state that the CSAf of chronic SCI subjects was 53% to 68% less than the CSAf of uninjured control subjects (Castro et al., 1999; Martin et al., 1992). Therefore, the present data demonstrate a similar progressive loss in CSAf and underscores the observation that the majority of changes in CSAf occur within the first 24 weeks following a spinal cord injury event. The CSAf in the SCI exercise group (IE) of the present study, increased by 63% above pre-training levels after 13 weeks of FES-CE training and was 171% greater than the SCI control group at the same time point post-SCI. Previously, Scremin et al. (1999) and Mohr et al. (1997) reported increases of 31% and 12% in vastus lateralis and thigh CSA respectively; with 1 year of FES-CE training in chronic SCI patients while Chilinbeck et al. (1999) reported a 23% increase in CSAf with 8 weeks of training. However, the increases in both CSAf and whole muscle CSA seen in training chronic SCI subjects are relative to the pre-training CSA, but when the training effect are compared with the CSAf of the uninjured control subjects, there is still an approximate 55% deficit. Thus, the training-induced increases in CSAf in the present study are greater in magnitude than seen in chronic SCI training studies (68% vs. 23%), and occurred following a much shorter training period. Also the CSAf post training in the acute SCI group was only 24% less than the uninjured control group CSAf versus approximately a 55% deficit seen in chronic SCI studies. Collectively, these observations again strongly support early intervention using FES-CE training as more effective in increasing CSAf following SCI. Following SCI muscle decreased power associated with decreases in whole muscle CSA and CSAf , may be partially compensated for through a shift of MHC isoforms from slow to fast (Castro et al., 1999; Burnham et al., 1997). Mohr et al. (1997) reported that in chronic SCI subjects, the MHC composition of the vastus lateralis muscle adapted to 5%, 33%, and 62% for Type I, Type IIa, and Type IIx MHC, respectively. However, in spite of the in spite of the large decrease in power MHC composition in SCI subjects was not different than control at either 6 or 19 weeks post-injury. Similarly, others (Castro et al., 1999) have reported no change in muscle MHC up to six months post-SCI. Therefore, changes in MHC in SCI subjects appears to occur at a slower time course than in other disuse models, such as spaceflight (Day et al., 1995) and further, decreased muscular power in SCI subjects appears to be largely due to decreased CSAf. The present study also showed no changes in MHC composition due to FES-CE training, whereas, studies utilizing FES-CE training with chronic SCI subjects reported changes in MHC isoforms from Type IIx to Type IIa, but no changes in Type I MHC composition (Mohr et al., 1997). One possible reason for no training effect of FES-CE on MHC in the present study was that no shift in MHC had occurred pre- training, therefore no stimulus for a reversal adaptation was present. Another possibility is that while the training stimulus was not great enough to cause shifts in MHC isoform profile, in situ hybridization could have potentially documented an up-regulation of the Type I MHC and down-regulation of Type IIx MHC isoform mRNA as noted by Harridge, et al in their electrical stimulation study involving chronic SCI survivors. The precise stimulus parameters needed to maximally elicit adaptation of MHC isoforms using electrical stimulation are not currently known. One potential cause for lack of a training effect in response to FES with chronic SCI subjects compared to that observed in this study utilizing acute SCI subjects could be a change in the adaptability of the muscle. Muscle adaptations are related to the number of myonuclei present within fibers (Allen et al., 1997). To date no data exist that report alterations in myonuclear density in either acute or chronic SCI patients. Previous studies in non-SCI subjects reported increases in the number of myonuclei with increases in CSAf (Hikida et al., 2000). In the present study, with acute SCI subjects, the loss of CSAf had no effect on myonuclear density. Therefore our data suggest that sufficient myonuclei are retained in acute SCI survivors to facilitate the increase in CSAf and a subsequent increase in power output, with FES-CE training. Thus, our findings on myonuclear density suggest that the large leg muscles of acute SCI patients retain their potential to adapt to functional demands. Although these data demonstrate an enhanced response to FES training in acute SCI subjects relative to chronic SCI subjects, the CSAf had already decreased by 36% at our baseline assessment of 4-6 weeks post-SCI. These data would then suggest that to maximally conserve the cross-sectional area of the muscle, training should start immediately post-injury. However, the time required to recover from surgical stabilization, secure hemodynamic stability, and recover from spinal shock sufficiently to observe muscle contractions in response to stimulation realistically precludes the initiation of a FES-CE training program sooner than our baseline 4-6 weeks post-injury. A potential limitation of this study is the sample size and therefore the applicability of the study to the general SCI population. The small sample size (n = 5 per group) occurred despite recruitment of individuals with SCI from a large Midwestern rehabilitation program with approximately 100 admissions for SCI rehabilitation per year. The recruitment pool was primarily limited by the inclusion criteria that potential subjects must have a motor complete injury and therefore fall within an ASIA A or B (American Spinal Injury Association) injury severity category due to the concern that the active exercise through volitional movement during the concurrent rehabilitation of patients with ASIA C or D type injuries would confound our outcome variables (Maynard et al., 1997). The second largest category of subjects excluded from the study involved injury related issues such as lower extremity fractures precluding FES-CE training, peripheral nerve injuries resulting in an inadequate response to electrical stimulation and thromboembolic disease requiring full dose anticoagulation, thereby precluding a muscle biopsy. Finally, the third significant barrier to subject recruitment involved transportation difficulties to training sessions.
ConclusionsIn conclusion these data indicate that early intervention after SCI with FES-CE attenuates the changes in both the CSAf and the loss of power output more effectively than that seen with chronic SCI and the maintenance of myonuclei suggests a preservation of the muscle’s potential to adapt to functional demands. In view of the fact that the medical and neurologic consequences of the acute SCI precludes earlier intervention with FES than the 4-6 weeks after injury seen in this study, the rehabilitation and performance implications of these observations suggest that further development of training and stimulation protocols that enhance muscle strength and endurance for functional purposes such are needed. Future studies may therefore consider utilizing a combination of isotonic extension against resistance with FES-CE. Additionally, modifying the FEC-CE protocol once weekly to incorporate “sprint ”training with repeated short bouts of high relative resistance followed by a rest period could be explored as mechanism to elicit a greater training effect.
AcknowledgementsFunding was provided National Institute on Disability and Rehabilitation Research # H133A980056.
|
AUTHOR BIOGRAPHY |
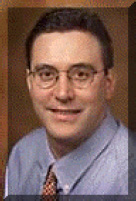 |
Timothy J. Demchak |
Employment: Department of Athletic Training, Indiana State University. |
Degree: PhD. |
Research interests: Therapeutic Modalities, Post-Injury Muscle Regeneration. |
E-mail: tdemchak@indstate.edu |
|
 |
Jon K. Linderman |
Employment: Department of Health and Sports Science, University of Dayton. |
Degree: PhD. |
Research interests: Muscle Physiology. |
E-mail: jonlinderman@udayton.edu |
|
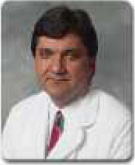 |
W. Jerry Mysiw |
Employment: College of Medicine and Public Health, The Ohio State University. |
Degree: MD. |
Research interests: Head Injury, Functional Electrical Stimulation, Osteoporosis |
E-mail: |
|
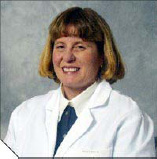 |
Rebecca Jackson |
Employment: College of Medicine and Public Health, The Ohio State University. |
Degree: MD. |
Research interests: Osteoporosis; Metabolic bone disease |
E-mail: |
|
 |
Jihong Suun |
Employment: Department of Physical Medicine & Rehabilitation., The Ohio State University, Columbus. |
Degree: MD. |
Research interests: Osteoporosis; Metabolic bone disease |
E-mail: |
|
 |
Steven T. Devor |
Employment: School of Physical Activity and Educational Services, The Ohio State University. |
Degree: PhD. |
Research interests: skeletal muscle physiology |
E-mail: |
|
|
|
REFERENCES |
 Allen D.L., Linderman J.K., Roy R.R., Grindeland R.E., Mukku V., Edgerton V.R. (1997) Growth hormone/IGF-I and/or resistive exercise maintains myonuclear number in hindlimb unweighted muscles. Journal of Applied Physiology 83, 1857-1861.
|
 Baldi J.C., Jackson R.D., Moraille R., Mysiw W.J. (1998) Muscle atrophy is prevented in patients with acute spinal cord injury using functional electric stimulation. Spinal Cord 36, 463-469.
|
 Brooks S.V., Faulkner J.A. (1988) Contractile properties of skeletal muscles from young, adult and aged mice. Journal of Physiology 404, 71-82.
|
 Burnham R., Martin T., Stein R., Bell G., MacLean I., Steadward R. (1997) Skeletal muscle fiber type transformation following spinal cord injury. Spinal Cord 35, 86-91.
|
 Castro M.J., Apple D.F., Staron R.S., Campos G.E., Dudley G.A. (1999) Influence of complete spinal cord injury on skeletal muscle within 6 mo of injury. Journal of Applied Physiology 86, 350-358.
|
 Chilinbeck P.D., Jeon J., Weiss C., Bell G., Burnham R. (1999) Histochemical changes in muscle of individuals with spinal cord injury following functional electrical stimulated exercise training. Spinal Cord 37, 264-268.
|
 Day M.K., Allen D.L., Mohajerani L., Greenisen M.C., Roy R.R., Edgerton V.R. (1995) Adaptations of human skeletal muscle fibers to spaceflight. Journal of Gravitational Physiology 2, 47-50.
|
 Faghri P.D., Glaser R.M., Figoni S.F. (1992) Functional electrical stimulation cycle ergometry exercise: training effects on cardiorespiratory responses of spinal cord injured subjects at rest and during submaximal exercise. Archives of Physical Medicine and Rehabilitation 73, 1085-1093.
|
 Faulkner J.A., Brooks S.V., Zerba E. (1995) Muscle atrophy and weakness with aging: contraction-induced injury as an underlying mechanism. The Journals of Gerontology: Biological Sciences and Medical Sciences 50A, 124-129.
|
 Gerrits H.L., Haan A., Sargeant A.J., Dallmeijer A., Hopman M.T. (2000) Altered contractile properties of the quadriceps muscle in people with spinal cord injury following functional electrical stimulated cycling training. Spinal Cord 38, 214-223.
|
 Harrridge S.D.S., Andersen J.L., Hartkopp A., Zhou S., Birring-Sorenson F., Sandri C., Kjaer M. (2002) Training by low-frequency stimulation of the tibialis anterior in spinal cord-injured men. Muscle and Nerve 25, 685-694.
|
 Hikida R.S., Staron R.S., Hagerman F.C., Walsh S., Kaiser E., Shell S., Hervey S. (2000) Effects of high-intensity resistance training on untrained older men. II. Muscle fiber characteristics and nucleo-cytplasmic relationships. Journal of Gerontology: Biological Sciences 35, B347-B354.
|
 Hooker S.P., Figoni S.F., Rodgers M.M., Glaser R.M., Mathews T., Suryaprasad A.G., Gupta S.C. (1992) Physiologic effects of electrical stimulation leg cycle exercise training in spinal cord injured persons. Archives of Physical Medicine and Rehabilitation 73, 470-476.
|
 Hutchinson K.J., Linderman J.K., Basso D.M. (2001) Skeletal muscle adaptations following spinal cord contusion injury in rat and the relationship to locomotor function: a time course study. Journal of Neurotrauma 18, 1075-1089.
|
 Luna L.G. (1968) Manual of histologic staining methods of the armed forces institute of pathology. Inc, New York. McGraw-Hill.
|
 Martin T.P., Stein R.B., Hoeppner P.H., Reid D.C. (1992) Influence of electrical stimulation on morphological and metabolic properties of paralyzed muscle. Journal of Applied Physiology 72, 1401-1406.
|
 Maynard F.M., Bracken M.B., Creasey G., Ditunno J.F., Donovan W.H., Ducker T.B., Garber S.L., Marino R.J., Stover S.L., Tator C.H., Waters R.L., Wilberger J.E., Young W. (1997) International standards for neurological and functional classification of spinal cord injury. Spinal Cord 35, 266-274.
|
 Mohr T., Anderson J.L., Biering-Sorensen F., Galbo H., Bangsbo J., Wagner A., Kjaer M. (1997) Long term adaptation to electrically induced cycle training in severe SCI individuals. Spinal Cord 35, 1-16.
|
 Rochester L., Chander C.S., Johnson M.A., Sutton R.A., Miller S. (1995a) Influence of electrical stimulation of the tibialis anterior muscle in paraplegiac subjects. 1. Contractile properties. Paraplegia 33, 437-449.
|
 Rochester L., Chander C.S., Johnson M.A., Sutton R.A., Miller S. (1995b) Influence of electrical stimulation of the tibialis anterior muscle in paraplegiac subjects. 2. Morphological and histochemical properties. Paraplegia 33, 514-522.
|
 Scremin A.M.E., Kurta L., Gentili A., Wiseman B., Perell K., Kunkel C., Scremin O.U. (1999) Increasing muscle mass in spinal cord injured persons with a functional electrical stimulation exercise program. Archives of Physical Medicine and Rehabilitation 80, 1531-1536.
|
 Talmadge R.J., Roy R.R. (1993) Electrophoretic separation of rat skeletal myosin heavy chain isoforms. Journal of Applied Physiology 75, 2337-2340.
|
|
|
|
|
|
|