|
|
|
ABSTRACT |
Despite increasing use of whole body vibration during exercise an understanding of the exact role of vibration and the supporting physiological mechanisms is still limited. An important aspect of exercise analysis is the utilisation of oxygen, however, there have been limited studies considering tissue oxygenation parameters, particularly during dynamic whole body vibration (WBV) exercise. The aim of this study was to determine the effect of adding WBV during heel raise exercises and assessing changes in tissue oxygenation parameters of the lateral gastrocnemius using Near Infra Red Spectroscopy (NIRS). Twenty healthy subjects completed ten alternating sets of 15 heel raises (vibration vs. no vibration). Synchronous oxygenation and motion data were captured prior to exercise to determine baseline levels, for the duration of the exercise and 20 sec post exercise for the recovery period. Both vibration and no vibration conditions elicited a characteristic increase in deoxyhaemoglobin and decreases in oxyhaemoglobin, total haemoglobin, tissue oxygenation index and normalised tissue haemoglobin index which are indicative of local tissue hypoxia. However, the addition of vibration elicited significantly lower (p < 0. 001) depletions in oxyhaemoglobin, total haemoglobin, normalised tissue haemoglobin index but no significant differences in deoxyhaemoglobin. These findings suggest that addition of vibration to exercise does not increase the cost of the exercise for the lateral gastrocnemius muscle, but does decrease the reduction in local muscle oxygenation parameters, potentially resulting from increased blood flow to the calf or a vasospastic response in the feet. However, further studies are needed to establish the mechanisms underlying these findings. |
Key words:
Vibration, NIRS, oxygenation, gastrocnemius, Heel raise
|
Key
Points
- Whole body vibration affects tissue oxygenation of the lateral gastrocnemius.
- The underlying mechanism could be either increased blood flow or a vasospastic response in the feet.
- The local metabolic cost of heel raise activity on the lateral gastrocnemius does not appear to be increased by whole body vibration.
|
Recent years have seen an increasing popularity of whole body vibration (WBV) as an exercise modality. As a consequence there is an increased need for greater understanding of the effects of the addition of vibration to exercise. The effects of WBV on the musculoskeletal system have been the subject of much research in the last decade and have been summarised in recent reviews (Cochrane, 2011; Rittweger, 2010; Rauch, 2009). Studies on the effects of vibration are essentially split into two categories, those on occupational vibration and those on vibration as an exercise modality. Typically occupational vibration is much higher frequency (>100 Hz) with exposure over longer durations e.g. hours each day (Griffin, 1990). Vibration for exercise purposes is typically much shorter in duration e.g. minutes on a few days per week, with much lower frequencies and amplitudes (Dolny and Reyes, 2008). To date there have been few studies investigating the effect of WBV on tissue blood flow and oxygenation parameters. Nakamura et al. (1996) was one of the first researchers to report that vibration exercise has different blood flow responses to occupational vibration, in that blood flow is increased to the digits of the hand. Laser Doppler studies have shown that application of both local vibration (Maloney-Hinds et al., 2008) and WBV during isometric weight-bearing exercise (Lohman III et al. 2008) significantly increased skin blood flow without subsequent vasoconstriction during the recovery period. Kerschan-Schindl et al., 2001 reported a 100% increase of blood flow in the popliteal artery (from 6.5 to 13cm s-1), corresponding to Lythgo et al., 2009 who found an increased mean blood cell velocity in the femoral artery following WBV. However, previously Hazell et al., 2008 reported no difference in the femoral artery from WBV in addition to Button et al. (2007) who found local vibration did not affect blood flow. Cardinale et al., 2007 investigated the effects of vibration during a static squat on vastus lateralis and gastrocnemius oxygenation, however no statistically significant results were found. More recently Coza et al., 2011 investigated gastrocnemius muscle oxygenation during heel raise exercise in arteriolar occluded (AO) conditions with respect to performance and recovery, both of which are dependent on blood flow. Near Infra-Red Spectroscopy (NIRS) has been shown to provide valid, non-invasive measurements regarding tissue oxygenation parameters (Boushel et al., 2001; McCully and Hamaoka, 2000; Suzuki et al., 1999) and provides information on combined arteriolar, capillary and venular haemoglobin concentrations (Quaresima et al., 2001). The signal obtained is therefore dependent on both oxygen delivery and rate of use. The aim of this study was therefore to assess the acute effects of WBV vibration during dynamic exercise on NIRS-derived muscle oxygenation parameters.
This study was carried out in accordance with University Ethics Guidelines and the ethical standards of the Declaration of Helsinki. All participants gave informed consent and received familiarisation of the procedure before data collection. Twenty physically active subjects (14 male, 6 female, age 29 ± 10. 4 years, height 1.75 ± 0.09m, weight 76.2 ± 17.15 kg, BMI 24.8 ± 4.3), with no recent history of lower limb musculoskeletal disorders were selected for inclusion in the study.
Study designAll heel raise exercises were performed on a Power Plate® pro6 (Power Plate International Ltd) whole body vibrating platform (40Hz 1.9mm vertical displacement), with either no vibration (NVIB) or vibration (VIB) being utilised in ten alternating sets of 15 heel raises. The initial set for each subject was randomised (i.e. VIB or NVIB). The exercises were completed using a metronome operating at 1Hz to ensure all exercises were completed at the same pace. The subjects were instructed to move at a pace of 0.5Hz i.e. one second up on to toes to maximum heel raise and one second down to complete flat foot and to ensure each repetition was a full heel raise i.e. as far up onto their toes as possible. Subjects were also instructed to keep a slight bend on their knees to prevent excessive transmission to their heads. During straight leg heel raise activity although the soleus muscle contributes to the movement, the prime activity comes from the gastrocnemius which is mechanically better positioned to generate full power while the knee is extended compared to when flexed (Baechle and Earle, 2008; Palastanga et al., 2004).
Data collection and processingTissue oxygenation parameters were obtained using a NIRO 300 (Hamamatsu Photonics, Japan), the emitter and recording sensor were placed on the right lateral gastrocnemius with the central distance between the emitter and detector 1/3 of the distance between the head of the fibula and the calcaneus. A constant distance of 4cm was maintained between the emitter and the detector. Analogue output to a USB AD board allowed synchronous oxygenation and motion data capture. One retro-reflective marker was placed on the right lateral malleolus and tracked for 60 seconds at 20Hz to determine baseline activity, ankle motion and recovery period (Oqus3, Qualysis SB, Sweden). Marker motion was tracked and all synchronous data exported in c3d format for subsequent analysis in Visual3D (C-Motion). Motion data was filtered (6Hz, 4th order low pass Butterworth filter), maximal and minimal vertical displacements were defined from which vertical ankle displacements were derived as well as total exercise time. Voltage calibration was used to convert oxygenation signals to appropriate values; these data were then smoothed using a 0.2Hz 4th order lowpass Butterworth filter. All signals were baseline corrected relative to the first 5 seconds of data prior to initiation of the exercise. Maximal or minimum values during the exercise period were used to determine absolute concentration changes for deoxyhaemoglobin (∆HHb), oxyhaemoglobin (∆O2Hb), total haemoglobin (∆cHb), and tissue oxygenation index (TOI, the ratio of oxygenated to total tissue haemoglobin) and normalised tissue haemoglobin index (nTHI, the relative concentration of total haemoglobin).
Statistical analysisMean values of the five VIB and five NVIB repetitions were determined for each participant and group mean data are presented as means ± SEs. Data were checked for normality (Shapiro-Wilk test) and between-conditions analysed using a Paired-Samples T- Test where distribution was normal and Wilcoxon signed rank test with non-normal data distributions. Cross correlation analysis was completed to identify any relationship between movement and the cyclical changes observed in some of the oxygenation parameters. Gender differences were assessed using one way ANOVA. The minimal level of significance was set at p < 0.05. PASW Statistics 18 software (IBM Corporation, USA) was used for statistical analysis.
No significant differences were observed in range of motion (NVIB: 9.7 ± 0.4 cm, VIB: 9.2 ± 0.02 cm) or in the time taken to complete each set of exercises (NVIB 29.4 ± 0.2 sec, VIB: 29.3 ± 0.3 sec). Peak changes in NIRS muscle oxygenation parameters during heel raise exercises between NVIB and VIB conditions are shown in Table 1. Aggregate time series data representing increasing ∆ HHb and decreasing ∆ O2Hb profiles are shown in Figures 1a and 1b respectively which together are characteristic of tissue hypoxia. NVIB and VIB conditions produced very similar ∆ HHb profiles with no significant difference in absolute concentration changes. However, the ∆ O2Hb profiles showed a higher O2Hb depletion during exercise in the NVIB condition. In the VIB condition O2Hb depletion was significantly reduced relative to NVIB (p < 0.001). Aggregate time series data representing ∆ cHb and nTHI profiles are shown in Figures 2 and 3">3 respectively. During exercise in both NVIB and VIB conditions the time series data show similar patterns with an initial rapid decrease in response to the onset of exercise, a slow but linear recovery during the exercise, followed by a rapid increase in both ∆cHb and nTHI ending in levels above the pre-exercise baseline. There were no significant differences in the slow recovery component slopes during the exercise protocol; however, the decrease in blood volume (as indicated by ∆cHb and ∆nTHI) during the VIB condition was less than half of that seen during the NVIB condition (p < 0.001). Aggregate time series data representing TOI profiles are shown in Figure 4. Similar profiles were observed in both NVIB and VIB conditions with an initial linear decrease in tissue oxygenation from the onset of exercise and a corresponding linear increase following cessation of exercise. A higher level of tissue desaturation was observed in the NVIB condition compared to the VIB condition, however the absolute changes from baseline to minimal TOI values did not reach significance (p = 0.151) nor did the slopes of the initial linear rate of change in TOI (p = 0.158). When investigating gender difference ANOVA only highlighted differences in ∆ HHb with females producing significantly less HHb during both NVIB and VIB conditions compared to male participants (p < 0.05). All oxygenation parameter time series profiles exhibited cyclical changes during the exercise protocol. These data were compared to the ankle motion time series using cross correlation analysis. This showed the cyclic nature of ankle motion and changes oxygenation parameters had the highest level of correlation (r = 0.473) with a lag difference of only 0.6 s.
The results obtained indicate that there are significant differences in tissue oxygenation resulting from the addition of whole body vibration to heel raise exercise. Whilst NIRS does not precisely measure blood flow, changes in haemoglobin levels point towards blood volume in the area assessed (Boushel et al., 2001; Bhambhani et al., 2000; Mileva et al., 2006) and changes can be an indication of oxygen delivery and utilisation in non-occluded conditions (McNeil et al., 2006). The changes in cHb observed in this study suggest an initial decrease in blood volume/flow, likely to be a consequence of 'start up costs' of exercise, followed by an increase in blood volume/flow. The differences observed in cHb, nTHI and O2Hb suggest that the addition of vibration during exercise reduces the depletion of these measures. Since the nTHI is based on an assessment of an unknown path length, the actual tissue volume assessed is not known. Therefore the units obtained are arbitrary units, however the relative level of changes are an indication of changes in blood volume or blood flow, with potential to discriminate between arterial (decreased nTHI) or venous (increased nTHI) occlusions (Myers et al., 2009). During exercise decreased levels of available oxygenated haemoglobin in conjunction with increased levels of deoxygenated haemoglobin are indicative of local muscular hypoxia; therefore any intervention which alters these parameters has potential to influence local muscle hypoxia. The pattern of change observed in nTHI is very similar to that reported by Coza et al., 2011 when investigating the effect of vibration in arterially occluded and non occluded blood flow during vibration exercise, the changes in nTHI were attributed to increased blood flow. These results also reflect previous research which has shown the rate of blood flow in the popliteal artery doubles during vibration exercise (Kerschan-Schindl et al., 2001). The increase in blood flow potentially explains the lower levels of depletion in oxygenated haemoglobin found in this study. Influx of blood to exercising muscles brings additional haemoglobin and, in the absence of occlusion, will influence the recorded levels of oxygenated haemoglobin. This increase in blood flow and volume will also influence tissue saturation and therefore TOI (Quaresima and Ferrari, 2009) which may explain the lack of a significant difference observed between the exercise conditions. The similar ∆ HHb profiles observed during exercise with and without vibration suggest that the mechanical and metabolic costs of each protocol were essentially the same. The only parameter noted to have a significant difference between male and female participants was ∆ HHb. It could be suggested that female subjects were subjected to less mechanical work (due to lower body masses), or that gender differences in subcutaneous adipose tissue thickness affected NIRS signals Cooper et al., 2010. However, further work in this area is required to determine the relative impact of subcutaneous adipose tissue thickness on each of the NIRS signals. It should also be noted that the female sample group was small (n = 6); therefore caution must be taken when drawing gender specific conclusions. Interestingly the inverse relationship between changes in the levels of nTHI and position of the ankle suggests that systematic drops in haemoglobin occur at the point in time when the ankle is at its highest point i.e. at the peak of muscle contraction. This decrease could either be indicative of maximum use of haemoglobin at the point when the muscle is working hardest, or reduced blood flow due to the muscle working isometrically for brief period at the point of maximum heel raise. It should also be considered that a potential consequence of vibration exercise is a shift in the type of fibres being utilised to perform the exercise from type II fibres to type I fibres. Potentially this could have resulted in greater increases in HHb levels which were not observed. However, it should be noted that the precise relationships between the metabolic and microvascular heterogeneities of muscle fibre types has not yet been fully elucidated (Koga et al., 2007). In order to address these questions the exercise protocol could be repeated whilst recording electromyographic activity to provide a greater insight into the possibility of muscle fibre activity. Previous studies have investigated the role of the gastrocnemius contraction as a muscle pump in relation to blood flow and venous return. The validity of muscular contractions influencing venous return remains a controversial issue with many authors reporting no influence and suggestions that increased blood flow is more likely to be a result of increased vasodilatation. (Clifford et al., 2005; 2007; Hamann et al., 2003; 2004; Nagami, 1990). It should also be considered that a more distal response, such as vasospasm in the feet, could result in a resistance to blood flow from the calves. Recently Thompson et al., 2010 reported a case of occupational exposure resulting in vasoconstriction in the feet but not the hands; diagnostic testing indicated normal ankle brachial indices but reduced digital plethysmographic waveforms in the toes when exposed to cold. However, it should be noted that the case study was based on a miner with 18 years of exposure. While this potential mechanism has not been fully investigated and to the author's knowledge never investigated in response to WBV exercise, this type of local response could explain the results obtained. A final explanation to consider is that of a global response to vibration. Previous investigations of the extremities have indicated that local vibration to the hand has resulted in changes in circulatory disturbances of the foot (Egan et al., 1996; Sakakibara, 1994). These changes have been attributed to a central sympathetic vasoconstrictor reflex elicited by vibration (Egan, 1996). These findings partially confirm the earlier work of Greenstein and Kester, 1992 who investigated the effect of unilateral hand vibration with and without a nerve block. They found that in the majority of cases a bilateral response suggestive of a sympathetic vasoconstrictor reflex in the absence of a digital nerve block was obtained. When the nerve block was administered the response was absent and vasodilatation was noted. However, it should also be noted that in some subjects vasodilatation was noted without the nerve block. The authors concluded that acute vibration may elicit both a vasoconstrictor reflex and an active local vasodilatation, with both mechanisms competing against each other. In 2002 Schweigert conducted a systemic review to establish if competing hypothesis regarding the underlying mechanisms for circulatory disturbances of the extremities in response to vibration could be developed in to an established theory. Unfortunately the studies were found to have significant validity flaws such as lack of independent variables, selection and survivor bias, the assessment of confounding exposures and the lack of blinding of investigators. The ultimate conclusions were that there is some evidence for lower limb vascular symptoms (cold induced vasospasm) to be associated with Hand Arm Vibration Syndrome (HAVS) but not in workers exposed to vibration without HAVS. The effect on local vascular structures has also been investigated by assessing pulse wave velocity and blood pressure at the ankles as an estimate of peripheral arterial stiffness. While it is difficult to distinguish between peripheral and central arterial stiffness, it was suggested that by obtaining measurements at the ankles peripheral influence would be greater (Otsuki et al., 2008). Results obtained indicated that arterial stiffness reduced approximately 20 minutes after WBV exercise and lasted for up to 40 mins. The authors proposed that this may reflect vasodilatation via vascular endothelial function. This is supported by evidence that vibration during cycling resulted in an increase in the release of vascular endothelial growth factor (Suhr et al., 2007) which could result from increased shear stress in blood vessels, as mathematically modelled by Yue et al. (2007a; 2007b). The potential limiting factors of this study include: the small number of female participants; the fact only one muscle was monitored for changes in oxygenation; the lack of additional information such as EMG of the triceps surae, vascular changes in the feet and global effects of the sympathetic nervous system (such as sweating and or physiological changes in the hands). Once these areas have been addressed, in particular the effect of vibration on the vasculature of the feet, recommendations for exercise prescription and/or contraindications can be made. All of these areas present an opportunity for further research on vibration exercise.
The results obtained indicate that the addition of vibration to heel raise exercise does not increase the metabolic cost of completing the exercise for the lateral gastrocnemius muscle. However, the addition of vibration during exercise does decrease the reduction in local muscle oxygenation parameters potentially indicating less reduction in tissue blood volume and/or increased blood flow, this pattern of responses is indicative of reducing exercise induced tissue hypoxia. Nonetheless, it is important not to over interpret these results. To date it has not been fully established if the observed changes are a direct result of increased blood flow to the leg, or a consequence of a vasospastic response in the feet creating a blood pooling effect in the legs. Further studies should be undertaken to investigate these potential explanations before conclusions are formed and exercise/rehabilitation recommendations being issued.
ACKNOWLEDGEMENTS |
This project was partly funded by Power Plate International Ltd and produced within the Power Plate Research Institute at the University of Greenwich at Medway. The NIRS equipment was provided by the Department of Medical Physics and Bioengineering, University College London |
|
AUTHOR BIOGRAPHY |
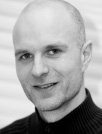 |
Daniel Robbins |
Employment: PhD Candidate University of Greenwich |
Degree: MSc |
Research interests: Vibration exercise; Neuromusculoskeletal biomechanics; neuromuscular physiology; Rehabilitation. |
E-mail: Daniel.W.Robbins@greenwich.ac.uk |
|
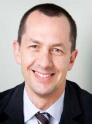 |
Clare Elwell |
Employment: Professor of Medical Physics |
Degree: PhD |
Research interests: Near infrared spectroscopy instrumentation and application |
E-mail: celwell@medphys.ucl.ac.uk |
|
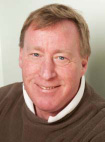 |
Alfonso Jimenez |
Employment: Professor University of Greenwich |
Degree: PhD |
Research interests: Clinical and performance exercise physiology |
E-mail: A.Jimenez@greenwich.ac.uk |
|
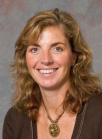 |
Mark Goss-Sampson |
Employment: Principle Lecturer University of Greenwich |
Degree: PhD |
Research interests: Sensorimotor control and biomechanics of human movement. |
E-mail: |
|
|
|
REFERENCES |
 Baechle T.R., Earle R.W. (2008) Essentials of strength training and conditioning. Human Kinetics , -.
|
 Bhambhani Y., Tuchak C., Burnham R., Jeon J., Maikala R. (2000) Quadriceps muscle deoxygenation during functional electrical stimulation in adults with spinal cord injury. Spinal Cord 38, 630-638.
|
 Boushel R., Langberh H., Olesen J., Gonzales-Alonzo J., Burlow J., Kjaer (2001) Monitoring tissue oxygenation availability with near infrared spectroscopy (NIRS) in health and disease. Scandinavian Journal of Medicine and Science in Sports 11, 213-222.
|
 Button C, Anderson N, Bradford C, Cotter J.D., Ainslie P.N. (2007) The effect of multidirectional mechanical vibration on peripheral circulation of humans. Clinical Physiology and Functional Imaging 27, 211-216.
|
 Cardinale M., Ferrari M., Quaresima V. (2007) Gastrocnemius medialis and vastus lateralis oxygenation during whole-body vibration exercise. Medicine and Science in Sports and Exercercise 39, 694-700.
|
 Clifford P.S. (2007) Skeletal muscle vasodilatation at the onset of exercise. Journal of Physiology 583, 825-833.
|
 Clifford P.S., Hamann J.J., Valic Z., Buckwalter J.B. (2005) Counterpoint: The muscle pump is not an important determinant of muscle blood flow during exercise. Journal of Applied Physiology 99, 372-374.
|
 Cochrane D.J. (2011) Vibration exercise: the potential benefits. International Journal of Sports Medicine 32, 75-99.
|
 Cooper C.E., Penfold S.P., Elwell C.C., Angus C. (2010) Comparison of Local Adipose Tissue Content and SRS-Derived NIRS Muscle Oxygenation Measurements in 90 Individuals. Advances in Experimental Medicine and Biology 66, 177-181.
|
 Coza A., Nigg B.M., Dunn J. (2011) Effects of Vibrations on Gastrocnemius Medialis Tissue Oxygenation. Medicine & Science in Sports & Exercise 43, 509-515.
|
 Egan C.E., Espie B.H., McGrann S., McKenna K.M., Allen J.A. (1996) Acute effects of vibration on peripheral blood flow in healthy subjects. Occupational and Environmental Medicine 53, 663-669.
|
 Griffin M.J. (1990) Handbook of Human Vibration. Elsevier Ltd.
|
 Hamann J.J., Buckwalter J.B., Clifford P.S. (2004) Vasodilation is obligatory for contraction-induced hyperemia in canine skeletal muscle. Journal of Physiology (London) 557, 1013-1020.
|
 Hamann J.J., Valic Z., Buckwalter J.B., Clifford P.S. (2003) Muscle pump does not enhance blood flow in exercising skeletal muscle. Journal of Applied Physiology 94, 6-10.
|
 Hazell T.J., Thomas G.W.R., De Guire J.R., Lemon P.W.R. (2008) Vertical whole-body vibration does not increase cardiovascular stress to static semi-squat exercise. European Journal of Applied Physiology 104, 903-908.
|
 Kerschan-Schindl K., Grampp S., Henk C., Resch H., Preisinger E., Fialka-Moser V., Imhof H. (2001) Whole-body vibration exercise leads to alterations in muscle blood volume. Clinical Physiology 21, 377-382.
|
 Koga S., Poole D.C., Ferreira L.F., Whipp B.J., Kondo N., Saitoh T., Ohmae E., Barstow T.J. (2007) Spatial heterogeneity of quadriceps muscle deoxygenation kinetics during cycle exercise. Journal of Applied Physiology 103, 2049-2056.
|
 Lohman III E.B., Petrofsky J.S., Maloney-Hinds M., Betts-Schwab H., Thorpe D. (2008) The effect of whole body vibration on lower extremity skin blood flow in normal subjects. Medical Science Monitor 13, CR71-76.
|
 Lythgo N., Eser P., de Groot P., Galea M. (2009) Whole-body vibration dosage alters leg blood flow. Clinical Physiology and Functional Imaging 29, 53-59.
|
 Maloney-Hinds C., Petrofsky J.S., Zimmerman G. (2008) The effect of 30 Hz vs. 50 Hz passive vibration and duration of vibration on skin blood flow in the arm. Medical Science Monitor 14, CR112-116.
|
 McCully K.K., Hamaoka T. (2000) Near-infrared spectroscopy: what can it tell us about oxygen saturation in skeletal muscle?. Exercise Sport Science Reviews 28, 123-127.
|
 McNeil C.J., Murray B.J., Rice C.L. (2006) Differential changes in muscle oxygenation between voluntary and stimulated isometric fatigue of human dorsiflexors. Journal of Applied Physiology 100, 890-895.
|
 Mileva K.N., Naleem A.A., Biswas S.K., Marwood S., Bowtell J.L. (2006) Acute Effects of a Vibration-like Stimulus during Knee Extension Exercise. Medicine & Science in Sports & Exercise 38, 1317-1328.
|
 Myers D., McGraw1 M., George M., Mulier K., Beilman G. (2009) Tissue hemoglobin index; a non-invasive optical measure of total tissue haemoglobin. Critical Care 13, S2-.
|
 Nagami K. (1990) Excess venous return immediately after brief contraction of human calf muscles. Tokai Journal of Experimental and Clinical Medicine 15, 81-86.
|
 Nakamura H, Okazawa T, Nagase H, Yoshida M, Ariizumi M., Okada A. (1996) Change in digital blood flow with simultaneous reduction in plasma endothelin induced by hand-arm vibration. International Archives of Occupational and Environmental Health 68, 115-119.
|
 Otsuki T., Takanami Y., Aoi W., Kawai Y., Ichikawa H., Yoshikawa T. (2008) Arterial stiffness acutely decreases after whole-body vibration in humans. Acta Physiologica 194, 189-194.
|
 Palastanga N., Field D., Soames R. (2004) . Anatomy and human movement, structure and function. Butterwoth Heinemann.
|
 Quaresima V., Colierc W.N., van der Sluijsb M., Ferraria M. (2001) Nonuniform Quadriceps O2 Consumption Revealed by Near Infrared Multipoint Measurements. Biochemical and Biophysical Research Communications 285, 1034-1039.
|
 Quaresima V., Ferrari M. (2009) Muscle oxygenation by near-infrared-based tissue oximeters. Journal of Applied Physiology 107, 372-373.
|
 Rauch F. (2009) Vibration therapy. Developmental Medicine and Child Neurology 51, 166-168.
|
 Rittweger J. (2010) Vibration as an exercise modality: how it may work, and what its potential might be. European Journal of Applied Physiology 108, 877-904.
|
 Sakakibara H. (1994) Sympathetic responses to hand-arm vibration and symptoms of the foot. Nagoya Journal of Medical Science 57, 99-111.
|
 Schweigert M. (2002) The relationship between hand-arm vibration and lower extremity clinical manifestations: a review of the literature. International Archives of Occupational and Environmental Health 75, 179-185.
|
 Suhr F., Brixius K., de Marěes M., Bölck B., Kleinöder H., Achtzehn S., Bloch W., Mester J. (2007) Effects of short-term vibration and hypoxia during high-intensity cycling exercise on circulating levels of angiogenic regulators in humans. Journal of Applied Physiology 103, 474-483.
|
 Yue Z., Kleinöder H., de Marěes M., Speicher U., Wahl P., Mester J. (2007b) On the cardiovascular effects of whole-body vibration. II: Statistical analysis. Studies in Applied Mathematics 119, 111-125.
|
 Yue Z., Mester J. (2007a) On the cardiovascular effects of whole-body vibration. I. Longitudinal effects: hydrodynamic analysis. Studies in Applied Mathematics 119, 95-109.
|
|
|
|
|
|
|