|
|
|
ABSTRACT |
We investigated effects of creatine (Cr) supplementation (CrS) on exercise-induced muscle damage. Untrained males and females (N = 27) ages 18-25, with no CrS history in the past 4 months, were randomly assigned to CrS (creatine and carbohydrate) (n = 9), placebo (P) (carbohydrate only) (n = 9), or control (C) (no supplements) groups (n = 9). Participants followed a 5-day Cr loading protocol of 40 g·day-1, divided for 5 days prior to exercise, reduced to 10 g g·day-1 for 5 days following exercise. Testing consisted of 5 maximal isometric contractions at 90 arm flexion with the preferred arm on a CYBEX NORM dynamometer, assessed prior to, immediately following, and 24, 48, 72, and 96 hours post muscle-damaging procedures. Damage was induced to the elbow flexor muscles using 6 sets of 10 eccentric contractions at 75 °/sec, 90 °/sec and 120 °/sec. Participants were asked to rate their muscle soreness on a scale of 1-10. Data was analyzed using repeated-measures ANOVA, with an alpha of 0.05. No significant differences were found between muscle force loss and rate of recovery or muscle soreness between groups over the 96 hr recovery period (p > 0.05). Across all 3 experimental groups an initial decrease in force was observed, followed by a gradual recovery. Significant differences were found between baseline and all others times (p = 0.031,0 .022, 0.012, 0.001 respectively), and between the 48 hour and 96 hour time periods (p = 0.034). A weak negative correlation between subjectively rated muscle soreness and mean peak isometric force loss (R2 = 0.0374 at 96 hours), suggested that muscle soreness and muscle force loss may not be directly related. In conclusion, 5 days of Cr loading, followed by a Cr maintenance protocol did not reduce indices of muscle damage or speed recovery of upper body muscles following eccentrically induced muscle damage. |
Key words:
Creatine loading, muscle damage, muscle force, muscle soreness.
|
Key
Points
- Creatine supplementation has been suggested as a means to diminish exercise induced muscle damage and speed post-damage muscle recovery but previous results have been contradictory
- Creatine supplementation followed by a creatine maintenance period failed to alter the degree of post-eccentric exercise muscle force loss or rate of recovery or muscle soreness in untrained young adult males or females
- These findings suggest that in accordance with other studies, arm muscles may not benefit from creatine supplementation as a prophylactic for exercise induced muscle damage or an enhancer of post-damage muscle recovery.
- Hence athletes may not benefit from creatine supplementation in order to diminish effects of overtraining on upper arm muscle function
|
Creatine supplementation (CrS) has a well established role in increasing maximal work output during short, high-intensity exercises (Balsom et al., 1995; Casey and Greenhaff, 2000). This is due to the ability of creatine to act as a rapid energy source in muscles, which supports rapid ATP resynthesis, and allows for the continuation of muscular work at a high intensity for a longer period of time (Koh, 2008; Rawson et al., 2001). CrS has also been reported to reduce indices of skeletal muscle damage (Bassit et al., 2010; Cooke et al., 2009) following exercise, although this result has not been consistently observed (Rawson et al., 2001). Unaccustomed bouts of high-force eccentric exercise have been well documented as a causative factor in muscle damage (Bassit et al., 2010; Clarkson and Sayers, 1999; Cooke et al., 2009; Koh, 2008; Proske and Allen, 2005; Rawson et al., 2001). Skeletal muscle damage is characterized by misaligned Z-discs and a disruption of the cell and sarcolemmal membrane (Clarkson and Hubal, 2002; Clarkson and Sayer, 1999; Ebbeling and Clarkson, 1989; Koh, 2008; Sayers and Clarkson, 2001). Damage is also associated with increased intracellular calcium ion concentrations, which can activate damaging calciumdependant degradation pathways (Clarkson and Sayers, 1999; Cooke et al., 2009; Proske and Allen, 2005). These factors, along with the resultant inflammatory response combine to decrease the force generating capacity of injured muscle, as well as increase the perception of muscle soreness (Warren et al., 1999). Thus any dietary supplements or exercise strategies that could potentially reduce or limit the deleterious effects of eccentrically induced muscle damage could be of value to athletes. Supplementation with Cr has been shown to play an important role in muscle damage and recovery. One mechanism by which Cr may affect muscle damage is though cell membrane stabilization. The molecular structure of phosphocreatine (PCr), allows it to bind to the phospholipid heads of the cell membrane and stabilize the membrane, reducing membrane fluidity and thus potentially decrease the amount of protein and cellular component loss associated with muscle damage (Saks and Strumia, 1993). Injections of PCr have also been reported to increase muscle force recovery in patients with muscle atrophy (Satolli and Marchesi, 1989). Olsen et al., 2006 demonstrated that CrS may influence post-exercise muscle recovery by enhancing muscle satellite cell proliferation. Since satellite cells are myogenic stem cells that fuse with damaged myocytes to effect repair (Lepper et al., 2011; Olsen et al., 2006; Shortreed, 2008), increased satellite cell proliferation could result in an increase recovery in muscle fiber integrity. Rawson et al., 2001 investigated effects of CrS following eccentric contractions of the biceps brachii. No significant differences in indices of muscle damage or recovery between the CrS group and a placebo (P) group were seen. The researchers suggested that the eccentric damage induced by their protocol may have been too severe for the creatine repair mechanisms to overcome. However more recent studies of CrS following eccentrically-induced muscle damage have reported CrS to not only reduced the initial amount of muscle damage, but also increase the rate of muscle recovery (Bassit et al., 2010; Cooke et al., 2009). Cooke et al., 2009 using a protocol similar to that of Rawson et al., 2001 found that the CrS group demonstrated significantly less post-exercise muscle damage (as evidenced by plasma CK and LDH levels) as well as a significantly more rapid recovery of muscle force. However, Cooke et al., 2009 investigated the knee extensor muscles where as Rawson et al., 2001 used the biceps brachii. Additionally Cooke et al., 2009 included a Cr maintenance protocol, by which subjects continued to ingest Cr post muscle damage in order to maintain elevated Cr levels within the muscle during the post-exercise recovery period, whereas Rawson et al., 2001 did not include such a maintenance protocol. Willoughby and Rosene, 2003 have suggested that continued supplementation of creatine after a bout of unaccustomed exercise may help to direct gene transcription and upregulate protein synthesis. Thus continued post-exercise CrS may help enhance muscle recovery rate. The purpose of this investigation was to determine if CrS, with a post-exercise CrS supplementation protocol, will reduce indices of muscle damage and increases the rate of force recovery of the elbow flexor muscles following eccentrically-induced muscle damage. It was hypothesized that the CrS group will demonstrate a smaller initial loss of muscle force, as well as a more rapid rate of muscle force recovery, following a bout of unaccustomed high-force eccentric contractions. Also, the perception of muscle soreness will be reduced in the CrS group.
SubjectsAll aspects of this study were reviewed and approved by the Wilfrid Laurier University Human Ethics Research Committee. A total of 27 male (n = 15) and female (n = 12) participants between the ages of 18-24 completed the experimental protocol. Subjects were recruited from the Wilfrid Laurier University community. All participants had refrained from upper body strength training and diet supplementation (creatine or protein) for at least 4 months prior to commencement of the study. Participants were instructed to maintain a typical activity levels through the duration of the study. All participants were screened for health issues precluding physical activity participation using the PAR-Q questionnaire. Participants were also asked to complete an additional health questionnaire that screened out any persons with diabetes or renal dysfunctions that may be at risk due to the increased sugar intake required of participants in the placebo group. All participants provided written informed consent to participate in this study.
EquipmentTesting was completed using the CMSi (Stoughton, MA, USA) Cybex Humac Norm dynamometer at Wilfrid Laurier University (Waterloo, ON, Canada). The equipment set up was individualized to account for size variation in each participant prior to testing. Participants were given several practice trials to ensure they felt comfortable with the equipment, and to eliminate any practice effects in data collection.
Experimental designParticipants were randomly assigned into one of three groups containing 9 subjects each. Groups consisted of a creatine supplementation group (CrS), a placebo supplement group (P), and control (no supplement) (C) group. Randomization was achieved by having participants chose containers of supplement that had been numbered and recorded by a third party. Control subjects received no containers. In order to avoid detection of the supplement, the experimental supplement consisted of a mixture of creatine monohydrate (20g) and a carbohydrate supplement (20g) in order to blend consistency and taste. For the 5 days leading up to testing commencement, individuals in the CrS groups ingested powdered creatine monohydrate mixed in equal proportions with a carbohydrate supplement (maltodextrin). Supplementation was performed at a rate of 40 g of creatine·day-1, which was divided into two equal servings taken once in the morning (between 8 am and 10 am) and once in the evening (between 5 pm and 7 pm). Participants in the placebo group followed the same supplementation protocol consisting of 40 g of maltodextrin only, ingested twice per day. Both experimental groups were instructed to dilute their given supplement in 500mL of water. Subjects in the control group were given no supplementation for the 5 days prior to the commencement of testing. This creatine loading protocol has been documented to be an effective means of enhancing creatine stores within the muscle (Bassit et al., 2010). The experimental design is summarized in Figure 1. Subjects attended 6 testing sessions over an 11-day period. On day 1 of the experiment subjects read and signed all the necessary forms required for participation in the study. Participants were then given the opportunity to familiarize themselves with the procedure and equipment to eliminate any potential practice effects present in the study. They were then assigned to one of the 3 experimental groups. If the subject was assigned to either the CrS or P group they were given instruction on how to properly ingest their supplement. C subjects did not receive a supplement. All subjects were then asked to return to the laboratory 5 days later. On day 7 participants returned to the laboratory to perform their baseline testing. The measure of peak isometric elbow flexor force was taken with the arm locked at 90° elbow flexion relative to their anatomical zero. Peak force output of the elbow flexor was calculated based on the average of 5 maximal isometric contractions held for 4 seconds each. All testing was performed on the dominant arm of the subject. After having given each subject several minutes to rest, they then performed a muscle-damaging protocol that consisted of maximal force eccentric contractions. Subjects performed 60 maximal eccentric contractions that were divided into 6 sets of 10 repetitions, with a 45 second rest period between repetitions. The velocity of eccentric contractions was varied between sets (2 at 75°/sec, 2 at 90°/sec, and 2 at 120°/sec). This protocol has been used in previous studies and has been shown to be an effective means of inducing skeletal muscle damage (Cooke et al., 2009). The researchers also provided verbal encouragement to the subjects to help maintain maximal effort throughout the protocol. Finally on day 7 after being given adequate rest, participants performed their first post-test, which was performed in the same manner as the baseline testing. Subjects were then asked to return to the laboratory on each of the subsequent 4 days (days 8-11) in order to perform post-exercise testing, which consisted of 5 isometric contractions held for 4 seconds each. Subjects in the CrS and P group continued to ingest the supplement for all 4 days during the post-exercise testing. Supplementation during post-exercise testing consisted of 10 g·day-1 divided into two equal doses as explained above. This supplementation protocol has been used in previous studies in order to maintain elevated creatine levels in muscle (Cooke et al., 2009). The peak elbow flexor isometric force at times 0, 24, 48, 72, and 96 hours post exercise-induced muscle damage was compared to the baseline value to indicate the amount of muscle force attenuation that was initially induced, as well as the recovery of muscle force. The average peak torque generated across their 5 contractions was represented as a percentage of their baseline peak torque output. Previous literature has demonstrated that post-damage muscle force recovery is an accurate and non-invasive measure of muscle damage and recovery in human subjects (Cooke et al., 2009; Rawson et al., 2001; Warren et al., 1999) and has often been used as an indicator of the effectiveness of intervention strategies in mitigating muscle damage and enhancing muscle recovery (eg. Dawson et al., 2011). Ratings of perceived muscle soreness were also taken immediately following damage induction, as well as all 4 post-testing days. Perceived soreness was indicated on a scale ranking 1-10, 1 corresponding to “not sore at all” and 10 representing “extremely sore". This measure are a subjective indication of delayed onset muscle soreness (DOMS), which has also been used in previous human studies to quantify muscle damage and repair (Warren et al., 1999).
Statistical analysesAll data was analyzed using SPSS version 19 for Windows. Raw data was first normalized and expressed as a percentage of the individuals’ baseline (pre-muscle damage) values for each of the subsequent days of testing. Data was collapsed across groups, and a repeated measure analysis of variance (ANOVA) was conducted in order to find a main effect of time and ensure damage was induced in all subjects. All data was considered statistically significant at an alpha level of P ≤ 0.05. A split-plot ANOVA was then conducted to identify any potential interaction effects between experimental group and muscle force over time. A Tukey’s Post Hoc test was performed for any values that achieved statistical significance. A repeated measures ANOVA was used to analyze ratings of perceived muscle soreness of experimental groups over time. Pearson correlations were utilized to compare perceived soreness ratings to the percentage of muscle force loss at each post-testing time. Since muscle force loss is indicative to muscle damage, an increased loss of force production in the muscle could correlate with DOMS perceived by the subject.
Subject characteristicsTable 1 depicts the characteristics of all 27 subjects whose data was reported in this study, in their randomly assigned groups.
Isometric muscle force measuresResults for peak isometric elbow flexor force, collapsed across groups, are depicted in Figure 2. All subjects experienced a significant main effect of time (p < 0. 01), indicating a significant decrease in isometric force following a bout of eccentric exercise. These results confirm that muscle damage (as indicated by loss of muscle force) was induced by our protocol. The greatest deficit in force production was experienced at time 0 post-damage. This 0 time result is indicative of fatigue as well as actual muscle damage (Proske and Allen, 2005). Isometric muscle force gradually recovered over the 4 post testing days: however, even at 96 hours post-damage isometric muscle force was still significantly (p < 0.05) decreased from baseline elbow flexor torque values. Results of peak isometric muscle torque across groups are depicted in Figure 3. There was no significant difference (p > 0.05) between any of the experimental groups (CrS, P, or control) in recovery of elbow flexor muscles strength following a bout of high-force eccentric contractions.
Soreness measuresResults of perceived soreness measures are depicted in Figure 4. A significant main effect of time was reported (p < 0.01), however there was interaction effect between experimental groups (p = 0.289). The greatest perception of muscle soreness was experienced at 48 hours post-injury. Pearson correlations between perceived muscle soreness and muscle force loss for each of the post-test times ranged between -0. 193 and 0.138 and non-significant (p > 0.05) at each of the post-test times, indicating that perception of muscle soreness, rated on a 10-point scale, is not directly or temporally associated with muscle force loss.
Creatine supplementation failed to significantly influence indices elbow flexor muscle damage or rate of muscle recovery following eccentric muscle contractions. Following a bout of unaccustomed eccentric exercises with the elbow flexor muscles no significant differences were seen between muscle force loss and rate of recovery or muscle soreness between control, placebo or creatine supplemented groups over the 96 hr recovery period. These results suggest that creatine supplementation even with the inclusion of a creatine maintenance protocol, did not significantly reduce indices of muscle damage or rates of recovery in the elbow flexors following damaging exercise. It was also demonstrated that measures of perceived soreness did not significantly differ between groups. Additionally muscle soreness measures did not correlate with muscle force loss, and therefore may be indicative of unrelated post-damage physiological processes. Following repeated bouts of eccentric contractions, all participants showed a significant decrease in peak isometric force, followed but a progressive recovery of force over the subsequent 4 days (Figure 3). These results are in accordance with previous studies (Brown et al., 1996; Cooke et al., 2009; Rawson et al., 2001), demonstrating that the protocol used was successful in inducing muscle damage. A study by Rawson et al., 2001 found similar results to those of the present study and proposed that the eccentric exercise employed in their protocol may have been too extensive for CrS to have an influence on damage or recovery rates. They suggested that the damage induced by repeated eccentric contractions may have overwhelmed any protective mechanisms of creatine suggested by other research (eg. Olsen et al., 2006; Saks and Strumia, 1993; Satolli and Marchesi, 1989). However more recent research involving CrS have demonstrated significant reductions in muscle damage indices in subjects supplemented with creatine (Bassit et al., 2010; Cooke et al., 2009). Specifically Cooke et al., 2009 used an eccentric exercise protocol very similar to the protocol used by Rawson et al., 2001 and found that the CrS group had a significantly more rapid recovery as well as demonstrated attenuated indices of muscle damage when compared to a placebo supplementation group. Nevertheless, two fundamental differences existed between the experimental design of the present study and that of those that demonstrated positive effects of creatine supplementation on post-exercise muscle recovery which may account for these differing results. The present study and that of Rawson et al. , 2001 used the biceps brachii muscle, whereas Cooke et al., 2009 used the knee extensor muscles. Further, Cooke et al., 2009 included a creatine maintenance protocol in order to retain elevated creatine levels in the muscle post-damage, whereas Rawson et al., 2001 did not. The present study included a post-exercise CrS protocol to determine if the differing results of these studies could be explained by differing CrS protocols. Previous work by Willoughby and Rosene, 2003 had suggested elevated creatine content during the days following muscle damage may help to alter gene transcription and upregulate protein synthesis in the myofibril. However, since the present study used the elbow flexors while including a maintenance protocol and found no significant differences between CrS groups and other unsupplemented groups, we propose that conflicting results being reported by Rawson et al., 2001 and Cooke et al., 2009 may be primarily due to differences in the muscle groups being tested. The knee extensor muscles represent significantly more mass than the elbow flexors. Brault and Terjung (2003) investigated Cr uptake in muscles tissue and concluded that a greater amounts of creatine uptake occurs in muscle fibers with lower creatine content, and consequently a larger capacity to store creatine. The knee extensor muscles, with a larger mass and more muscle fibers would potentially have a greater total capacity to store and exploit ingested Cr, and thus may have a greater potential to respond to CrS related protective effects. Additionally, the knee extensor muscles differ from the elbow flexor muscles in muscle fiber type composition. Previous literature has determined that the knee extensor muscles (specifically the vastus lateralis) typically contain approximately 55% type I muscle fibers (Froese and Houston, 1985), whereas the elbow flexors (specifically the biceps brachii) contains approximately 40% type I muscle fibers (Klein et al., 2003). Casey and Greenhaff, 2000 reported that PCr utilization during muscle contractions can be up to 33% higher in fast twitch (Type IIx) muscle fiber relative to slow twitch. Thus it may be possible that the elbow flexor muscles, with a larger proportion of type II muscle fibers, may transiently turn over their stored supply of PCr during the eccentric contractions to a greater extent, and for at least a few potentially critical minutes following exercise have less PCr available to provide energy for potential recovery related effects such as satellite cell activation. The knee extensor muscles, by comparison, could have a larger Cr storage capacity and may turn over relatively less stored Cr during the eccentric contractions leaving a greater potential for PCr generated therapeutic effects. Differences in the utilization of Cr by different fiber types may be of particular importance immediately following eccentric exercise. Since PCr is better conserved in type I muscle fibers (Casey and Greenhaff, 2000), and PCr has been shown to have a membrane stabilizing effect in cardiac muscle tissue (Saks and Strumia, 1993), it is also possible that elevated levels of PCr in skeletal muscle fibers immediately following eccentric damage may help to stabilize the cell membrane and reduce the loss of protein and other cellular components. This may in part account for the more rapid increase in muscle force recovery in studies that investigated CrS in the quadriceps muscles (Bassit et al., 2010; Cooke et al., 2009), than the current study and others (Rawson et al., 2001) that used the biceps brachii muscle. Future research is needed in order to further delineate the mechanism by which CrS may affect specific muscle fiber types or muscle groups. There were also no significant differences in changes in perceived muscle soreness between the groups over the muscle damage and recovery time course (Figure 4). It was also noted that when collapsed across the groups and time, there were no significant positive correlations between muscle force or muscle soreness over the course of the post-exercise recovery period (Table 1). This suggests that changes in post-exercise muscle soreness and muscle force may be indicative of different aspects of muscle damage that are not temporally correlated. Nevertheless, neither of these indices of muscle damage or recovery indicated any influence of CrS. These results suggest that, as also reported by Rawson et al., 2001, CrS may not aid recovery from damaging muscular exercise, particularly in smaller upper body musculature. Hence despite other performance related benefits (McNaughton et al., 1998), competitors in sports with a high proportion of upper body muscular demand such as kayakers, and others may not benefit from CrS to enhance recovery of muscle force or ameliorate muscle soreness after intense damaging arm muscle training.
In summary, creatine supplementation had no effect on muscle force loss, muscle strength recovery or muscle soreness following eccentric exercise of the elbow flexor muscles, in young healthy subjects relative to placebo and control subjects. This suggests that supplementing creatine at a rate of 20/day for 5 days leading up to a muscle damage protocol does not reduce indices of muscle damage, or rates of subsequent muscle force recovery even with the inclusion of a creatine maintenance protocol (5 g·day-1) for the 4 days following damage. Thus, CrS does not reduce the amount of damage, or increase the rate of recovery following eccentrically-induced muscle damage specifically to the elbow flexor muscles.
ACKNOWLEDGEMENTS |
This study was supported by research funds from the Faculty of Science Student’s Association (FOSSA) of Wilfrid Laurier University and a Natural Sciences and Engineering Council of Canada (NSERC) Discovery Grant to PMT. |
|
AUTHOR BIOGRAPHY |
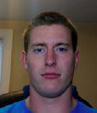 |
Neal B. McKinnon |
Employment: Wilfrid Laurier University |
Degree: BSc |
Research interests: Neuroscience, neuromuscular disorders and rehabilitation |
E-mail: mcki1220@mylaurier.ca |
|
 |
Mitchell T. Graham |
Employment: Wilfrid Laurier University |
Degree: BSc |
Research interests: Neuroscience, neuromuscular disorders and rehabilitation |
E-mail: grah1381@mylaurier.ca |
|
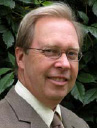 |
Peter M. Tiidus |
Employment: Professor of Kinesiology and Physical Education, Wilfrid Laurier University |
Degree: PhD |
Research interests: Muscle damage and repair mechanisms, therapeutic interventions in muscle damage |
E-mail: ptiidus@wlu.ca |
|
|
|
REFERENCES |
 Balsom P.D., Söderlund K., Sjödin B., Ekblom B. (1995) Skeletal muscle metabolism during short duration high-intensity exercise: influence of creatine supplementation. Acta Physiologica Scandinavica 1154, 303-310.
|
 Bassit R.A., Pinheiro C., Vitzel K.F., Sproesser A.J., Silveira L.R., Curi R. (2010) Effects of short-term creatine supplementation on markers of skeletal muscle damage after strenuous contractile activity. Journal of Applied Physioogy 1108, 945-955.
|
 Brault J.J., Terjung R.L. (2003) Creatine uptake and creatine transporter expression among rat skeletal muscle fiber types. American Journal of Physiology Cellular Physiology 284, C1481-C1489.
|
 Brown S.J., Child R.B., Donnelly A.E., Saxton J.M., Day S.H. (1996) Changes in human skeletal muscle contractile function following stimulated eccentric exercise. European Journal of Appied Physioogy 772, 515-521.
|
 Casey A., Greenhaff P.L. (2000) Does dietary creatine supplementation play a role in skeletal muscle metabolism and performance?. American Journal of Clinical Nutrition 72, 607S-617S.
|
 Clarkson P.M., Hubal MJ (2002) Exercise-induced muscle damage in humans. American Journal of Physical Medicine and Rehabilitation 881, S52-S52.
|
 Clarkson P.M., Sayers S.P. (1999) Etiology of exercise-induced muscle damage. Canadian Journal of Applied Physiology 224, 234-248.
|
 Cooke M.B., Rybalka E., Williams A.D., Cribb P.J., Hayes A. (2009) Creatine supplementation enhances muscle force recovery after eccentrically-induced muscle damage in healthy individuals. International Journal of Sports Nutrition 66, 13-.
|
 Dawson K.A., Dawson L., Tiidus P.M. (2011) Effectiveness of regular proactive massage therapy for novice recreational runners. Physical Therapy in Sport 12, 182-187.
|
 Ebbeling C.B., Clarkson P.M. (1989) Exercise-induced muscle damage and adapation. Sports Medicine 7, 207-234.
|
 Froese E.A., Houston M.E. (1985) Torque-velocity characteristics and muscle fiber type in human vastus lateralis. Journal of Applied Physiology 58, 309-314.
|
 Klein C.F., Marsh G.D., Petrella R.J., Rice C.L. (2003) Muscle fiber number in the biceps brachii muscle of young and old men. Muscle and Nerve 228, 62-68.
|
 Koh T.J., Tiidus P.M. (2008) Skeletal muscle damage and repair. Physiology and mechanisms of skeletal muscle damage. Champaign IL. Human Kinetics.
|
 Lepper C., Partridge T.A., Fan C.M. (2011) An absolute requirement for pax-7 positive satellite cells in acute injury-induced skeletal muscle regeneration. Development and Stem Cells 1138, 3639-3646.
|
 McNaughton L.R., Dalton B., Tarr J. (1998) The effects of creatine supplementation on high-intensity exercise performance in elite performers. European Journal of Applied Physiology and Occupational Physiology 778, 236-240.
|
 Olsen S., Aagaard P., Kadi F., Tufekovic G., Verney J., Olesen J.L., Suetta C., Kjaer M. (2006) Creatine supplementation augments the increase in satellite cell and myonuclei number in human skeletal muscle induced by strength training. Journal of Physiology 5572, 525-534.
|
 Proske U., Allen T.J. (2005) Damage to skeletal muscle from eccentric exercise. Exercise and Sport Sciences Reviews 333, 98-104.
|
 Rawson E.S., Gunn B., Clarkson P.M. (2001) The effects of creatine supplementation on exercise-induced muscle damage. Journal of Strength and Conditioning Research 115, 178-184.
|
 Saks V.A., Strumia E. (1993) Phosphocreatine: molecular and cellular aspects of the mechanism of cardio protection action. Current Therapy Research 553, 565-598.
|
 Satolli F., Marchesi G. (1989) Creatine phosphate in the rehabilitation of patients with muscle hyponotrophy of the lower extremity. Current Therapy Research 446, 67-73.
|
 Sayers S.P., Clarkson P.M. (2001) Force recovery after eccentric exercise in male and females. European Journal of Applied Physiology 884, 122-126.
|
 Shortreed K., Johnston A., Hawke T.J., Tiidus P.M. (2008) Skeletal muscle damage and repair. Satellite cells and muscle repair. Champaign IL. Human Kinetics.
|
 Smith L.L. (1992) Causes of delayed onset muscle soreness and the impact on athletic performance: A review. Journal of Applied Sport Science Research 6, 135-141.
|
 Tiidus P.M. (2008) Skeletal muscle damage and repair. Champaign IL. Human Kinetics.
|
 Warren G.L., Lowe D.A., Armstrong R.B. (1999) Measurement tools used in the study of eccentric contraction-induced injury. Sports Medicine 227, 43-59.
|
 Willoughby D.S., Rosene J.M. (2003) Effects of oral creatine and resistance training on myogenic regulatory factor expression. Medicne and Science in Sports and Exercise 335, 923-929.
|
|
|
|
|
|
|