|
|
|
ABSTRACT |
Previous studies reported faster pulmonary oxygen uptake kinetics at the onset of exercise in untrained youth compared with adults. Whether or not these differences are identical for trained groups have not been examined. The purpose of this study was to compare ̇VO2 kinetics of youth and adult cyclists at moderate and heavy-intensity exercise. Thirteen adult (age: 23.2 ± 4.8 years; ̇VO2peak 68.4 ± 6.8 mL·min-1.kg-1) and thirteen youth cyclists (age: 14.3 ± 1.5 years; ̇VO2peak 61.7 ± 4.3 mL·min-1.kg-1) completed a series of 6-min square wave exercises at moderate and heavy-intensity exercise at 90 rev·min-1. A two-way repeated-measure ANOVA was conducted to identify differences between groups and intensities. The time constant, time delay and the mean response time were not significantly different between youth and adult cyclists (p > 0.05). We found significant differences between intensities, with a faster time constant during moderate than heavy-intensity exercise in youth (24.1 ± 7.0 s vs. 31.8 ± 5.6 s; p = 0.004) and adults (22.7 ± 5.6 s vs. 28.6 ± 5.7 s; p < 0.001). The present data suggest that the effect of training history in adult cyclists compensate for the superior primary response of the oxygen uptake kinetics typically seen in youth compared to adults. Furthermore, the ̇VO2 response is dependent of work rate intensity in trained youth and adult cyclists. |
Key words:
Adolescents, endurance training, exercise physiology, physical performance, trained athletes
|
Key
Points
- No differences in the primary response between youth and adult cyclists at moderate and heavy-intensity exercise
- This suggests that adaptations to long-term training have a high potential as an influencing factor on the response of oxygen uptake kinetics
- Oxygen uptake kinetics are influenced by work rate in both youth and adult cyclists with a slower response at higher work rates.
|
The peak oxygen uptake (̇VO2peak) and oxygen uptake kinetics are key parameters of aerobic fitness (Whipp et al., 1981) and have been typically used to determine adaptations through endurance training. The ̇VO2peak represents the maximum rate to resynthesize ATP aerobically, whereas oxygen uptake kinetics provide an additional non-invasive insight into the integrated capacity of oxygen utilization and transport to the contracting myocytes at the onset of exercise (Poole and Jones, 2012). Furthermore, a close coupling between muscle PCr breakdown and oxygen uptake kinetics was observed at the on- and offset of exercise in children (Barker et al., 2008) and adults (Rossiter et al., 2002). Recent studies have shown that there are no differences in ̇VO2peak between untrained children and adults when a ratio standard (mL·min-1.kg-1) was used (Fawkner et al., 2002; Leclair et al., 2013). However, faster oxygen uptake kinetics have been reported in children and therefore it was suggested to consider both ̇VO2peak and oxygen uptake kinetics to determine aerobic fitness (McNarry and Jones, 2014; McNarry and Barker, 2018). Recent studies showed a faster time constant (𝜏) during moderate-intensity exercise in untrained children compared with adults (Armstrong and Barker, 2009; Fawkner and Armstrong, 2003; Fawkner et al., 2002; Leclair et al., 2013). Moreover, a faster 𝜏 with no or a significantly lower ̇VO2 slow component (SC) was found during high-intensity exercise in children compared to adults (Armon et al., 1991; Williams et al., 2001). In addition, longitudinal studies reported an increased 𝜏 and SC over a 2-year period in children and adolescents at heavy-intensity exercise (Breese et al., 2010; Fawkner and Armstrong, 2004). It is evident that training affects the parameters of oxygen uptake kinetics. In adults, cross-sectional studies showed a faster 𝜏 in trained compared to untrained subjects irrespective of exercise intensity and type of exercise (Caputo et al., 2003; Koppo et al., 2004). In addition, a six week (Jones and Carter, 2000) and eight week (Billat, 2000) endurance training in untrained adults, reduced the amplitude of the SC at the same absolute treadmill speed. Furthermore, within highly trained athletes, those with a higher ̇VO2peak showed a faster 𝜏 and a reduced SC (Ingham et al., 2007; Powers et al., 1985). Therefore, it can be assumed that a high training status as a result of a higher accumulated training volume (i.e. years of training), is associated with a faster 𝜏 and reduced amplitude of the SC (Poole and Jones, 2012). Whilst the effect of endurance training volume on parameters of oxygen uptake kinetics is well investigated in adults, relatively less is known about the responses in children and youth. Whilst early studies indicated no effect of training on oxygen uptake kinetics in prepubertal children (Cleuziou et al., 2002; Obert et al., 2000), more recent studies reported a significant effect of training. It has been shown, that trained children and adolescents showed faster adjustments in the oxidative response at the onset of exercise than their untrained counterparts irrespective of sex (Marwood et al., 2010; McNarry and Jones, 2014; McNarry et al., 2011a; McNarry et al., 2011b; Unnithan et al., 2015; Winlove et al., 2010). According to the results examining youth and adults, it appears that training status (i.e. endurance training history) is a potential factor to positively affect oxygen uptake kinetics and therefore exercise tolerance in both, youth and adults. Whilst the majority of studies compared oxygen uptake kinetics between untrained children and adults, comparisons of the ̇VO2 responses between endurance trained youth and adults have not been examined. Additionally, an influence of work rate on the ̇VO2 response is reported in adults (Poole and Jones, 2012). Although some results indicate, that the ̇VO2 response is independent of work rate in children no studies specifically investigated the influence of work rate on the ̇VO2 response in children (McNarry, 2019). The main purpose of the study was to compare oxygen uptake kinetics between endurance trained youth and adult cyclists. The second purpose was to determine differences in the oxygen uptake response at different exercise intensities within groups. We hypothesized, that the primary response would be faster in adult cyclists due to the higher training status and would be affected by exercise intensity within both groups. During heavy-intensity exercise, we expected a larger SC in adult than in youth cyclists.
ParticipantsNine adult male and four female (n = 13; mean ± SD; age: 23.2 ± 4.8 years; stature: 1.76 ± 0.09 m; body mass: 68.1 ± 10.0 kg) and ten youth male and three female (n = 13; mean ± SD; age: 14.3 ± 1.5 years; stature: 1.65 ± 0.12 m; body mass: 54.2 ± 11.7 kg) members of the Austrian Cycling Federation volunteered to participate in this study. The youth cyclists had a training history of 3-4 years and trained for 10 to 12 h per week at the time of the study. Adults training history was at minimum 10 years with 15 to 20 h of training per week. Before entering the study, all participants and the guardians of the youth cyclists were informed of the experimental procedures. The youth cyclists provided assent to participate and their guardians, as well as the adult cyclists, gave written informed consent. The study was conducted in accordance with the ethical principles of the Declaration of Helsinki and was approved by the institutional review board. Participants were instructed to arrive at the laboratory in a rested and fully hydrated state and to refrain from strenuous exercise in the 24 h prior each test.
Study designParticipants visited the laboratory on two occasions separated by 3-7 days. All participants performed a ramp-exercise test on a Cyclus-2 cycle ergometer (RBM Electronics, Leipzig, Germany) during the first visit. At the second visit participants performed two square-wave exercise transitions each at moderate and heavy-intensity exercise to obtain a 95% confidence interval in the primary response 𝜏 of ± 5 s. During all trials pulmonary ̇VO2 was measured continuously and laboratory temperature was held constant at 22° with a relative humidity of ~42%.
Laboratory ramp-exercise testParticipants performed the ramp-exercise test at a cadence of 90-100 rev·min-1 until exhaustion to determine maximal measures of power output (Pmax) and ̇VO2peak. Participants used their own bicycle which was mounted on a Cyclus-2 ergometer. After a 3-minute standardized warm-up at 60 W the work rate was increased continuously by 20 W·min-1 until exhaustion. Pulmonary ventilation and gas exchange were measured continuously throughout the test via breath-by-breath open circuit spirometry (MetaMax 3B, Cortex Biophysik, Leipzig, Germany). The gas analyzer was calibrated before each test with gases of known concentrations (4.99 Vol% CO2, 15.99 Vol% O2, Cortex Biophysik, Leipzig, Germany). Volume and flow were calibrated with a 3-L syringe (Type M 9474-C; Cortex Biophysik, Leipzig, Germany). The participants wore a facemask and breathed through a low-resistance impeller turbine. Achievement of ̇VO2peak was assumed as the highest 10-s average value attained before volitional exhaustion. In addition, the ventilatory threshold (VT) was determined as sub-maximal performance correlate. For determination of VT the criteria of an increase of the ventilatory equivalent of O2 (̇VE/̇VO2), without a concomitant increase of the ventilatory equivalent of CO2 (̇VE/̇VCO2) and the first loss of linearity in pulmonary ventilation (̇VE) and carbon dioxide ventilation (̇VCO2) were used (Beaver et al., 1986).
Square-wave exercise trialsThe trials were conducted at a cadence of 90 rev·min-1 for all intensities. The work rate for moderate-intensity exercise was set at 90% of VT and the work rate for heavy-intensity exercise was set at the difference of 50% between VT and Pmax (∆50%). After a 5-min warm-up period (i.e. cycling at 40 W), each trial started with 3-min baseline cycling at a power output of 60 W followed by 6 min cycling at moderate intensity separated by 10 min cycling at 60 W before 6 min cycling at heavy intensity. The protocol was repeated after a passive rest of 1 h (Nimmerichter et al., 2020). During all trials gas exchange was measured as described above.
Oxygen uptake kineticsThe ̇VO2 breath-by-breath data were examined to exclude errant breaths from sighing, swallowing, coughing, etc., and breaths lying more than four standard deviations from the local mean of 5 data points were removed. The filtered data were linearly interpolated to provide second-by-second values for each participant. In addition, the identical transitions from each participant were ensemble-averaged and subsequently time aligned to the onset of the exercise (Whipp and Rossiter, 2005). The cardio-dynamic or phase I response was visually identified and the first 15-20 s of the transition from baseline to the target work rate were excluded from further analyses (Hebestreit et al., 1998). A single-exponential equation was used to model the kinetics of the primary (phase II) response of ̇VO2:
̇VO2 (t) = ̇VO2baseline + Amp (1- e-(t-TD)/𝜏)
where ̇VO2 (t), ̇VO2baseline, Amp, TD and 𝜏 represent the ̇VO2 at any given time (t), the ̇VO2 at the last 60 s of baseline exercise, the amplitude from baseline to its asymptote, the time delay and the time constant of the response, respectively.
According to the methods of Rossiter et al. (2002) a purpose-designed software (LabView 6.1, National Instruments, Newbury, UK) was used to identify the optimal fitting window of the primary parameter response. Beginning from the initial 60 s of exercise, the fitting window was increased iteratively by 5-s to end-exercise. For ∆50% trials, each fitting window was plotted against time of the estimated 𝜏 and through visual inspection the onset of the SC was determined as the point at which the estimated 𝜏 progressively increased following an initial plateau. The parameter estimates were then resolved by least-squares non-linear regression (Graph Pad Prism 8.1, San Diego). The amplitude of the SC was calculated as the difference between the mean of the final 30 s at end-exercise and the asymptote of the fundamental response. The ̇VO2 gain of the primary response and of the end-exercise, was calculated by dividing the respective amplitudes by the incremental work rates above baseline (∆̇VO2/∆WR). In addition, mean response time (MRT) was calculated as 𝜏 + TD.
Statistical analysesStatistical analyses were performed using SPSS statistics 23 (IBM Corporation, Armonk, NY). Descriptive data are summarized as mean ± standard deviation (SD). After testing the assumption of normality using the Shapiro-Wilks’ test, a two-way repeated-measure ANOVA with Fisher’s LSD post-hoc test compared differences between groups (youth vs. adults) and intensities (moderate vs. heavy). In addition, an unpaired t-test was conducted to compare data obtained from the laboratory ramp-exercise test as well as the SC of the heavy-intensity exercise trials. The level of significance was set at p < 0.05.
The results of the ramp-exercise test and calculated target work rates for the square-wave trials are presented as absolute and normalized to body mass values in Table 1. There was a significant difference in all measures between youth and adult cyclists except the target work rate for 90% VT which was not significantly different between groups (p = 0.408) when normalized to body mass. The parameter estimates of the transitions to moderate- and heavy-intensity exercise for both groups are provided in Table 2. ANOVA revealed significant main effects of intensity for baseline ̇VO2 (F1; 24 = 8.9, p = 0.006), amplitude (F1; 24 = 319.5, p < 0.001), 𝜏 (F1; 24 = 29.9, p < 0.001), MRT (F1; 24 = 11.1, p = 0.003), end-exercise ̇VO2 (F1; 24 = 303.2, p < 0.001) and end-exercise ̇VO2 gain (F1; 24 = 4.8, p = 0.039). No significant main effect of intensity was found for TD (F1; 24 = 2.8, p = 0.106) and phase II ̇VO2 gain (F1; 24 = 0.9, p = 0.356). Significant group differences were found for baseline ̇VO2 (F1; 24 = 13.4, p = 0.001), phase II ̇VO2 gain (F1; 24 = 39.7, p < 0.001), end-exercise ̇VO2 (F1; 24 = 11.6, p = 0.002), end-exercise ̇VO2 gain (F1; 24 = 16.1, p = 0.001) and SC (p < 0.001). No significant differences were found for amplitude (F1; 24 = 3.0, p = 0.058), 𝜏 (F1; 24 = 1.3, p = 0.263), TD (F1; 24 = 2.5, p = 0.124) and MRT (F1; 24 = 0.03, p = 0.870). In addition, no significant interactions between intensity and group were found (p = 0.294 to p = 0.717). The mean ̇VO2 response for both intensities is illustrated in Figure 1.
The main finding of this study was that for moderate- and heavy-intensity exercise the phase II of the primary response is not significantly different between trained youth and adult cyclists. This is in contrast to our hypothesis that the primary response would be faster in adults and to studies comparing children and adults. Additionally, in both groups a SC was observed, with a significantly higher SC amplitude in adult cyclists compared to youth cyclists which is in turn comparable to results from studies comparing youth and adults. However, in the present study adult cyclists with a longer training history and higher training volume, showed significantly higher maximal and sub-maximal physiological characteristics for both, absolute and normalized values than youth cyclists. The differences in endurance performance in the present study are not surprising. It is well documented that prolonged endurance training over several years results in increased endurance performance. Therefore, the higher endurance performance in adult cyclists can be mainly explained by the differences in training history and consequently a higher accumulated training volume. We observed no significant differences in the phase II at the primary response of oxygen uptake kinetics at both intensities. Nevertheless, the ̇VO2 gain in the present study was significantly different between groups, with a higher ̇VO2 gain in youth, which is comparable to studies where untrained children showed a significantly higher ̇VO2 gain than adults (Armon et al., 1991; Fawkner and Armstrong, 2003; Williams et al., 2001). By comparing youth and adults, the higher ̇VO2 gain in youth is associated with a higher capacity of oxidative phosphorylation and/or a limited ability to generate ATP through anaerobic metabolism (Armstrong and Barker, 2009). Irrespective of age the positive influence of training status on oxygen uptake kinetics has been shown in recent studies (Koppo et al., 2004; Marwood et al., 2010; Winlove et al., 2010). Furthermore, studies investigating oxygen uptake kinetics within trained groups showed that those with a higher ̇VO2peak displayed a faster 𝜏 (Ingham et al., 2007; Powers et al., 1985). The results of the present study regarding 𝜏 are in contrast to the mentioned studies, thus an influence of age is most likely present. However, in the present study the differences in the ̇VO2 gain for the primary response are in line with previous studies, where youth or club level athletes showed a significantly higher ̇VO2 gain than adults or elite athletes (Ingham et al., 2007; Williams et al., 2001). In addition, we observed a SC in youth cyclists which was significantly smaller compared to adult cyclists, whereas the end-exercise ̇VO2 gain was higher in youth cyclists. The differences in SC are most likely due to the significantly greater absolute and relative work rate performed by adult cyclists during heavy-intensity exercise. Longitudinal and cross-sectional studies showed a slowing of 𝜏 and an increasing SC at heavy-intensity exercise with age (Breese et al., 2010; Fawkner and Armstrong, 2004; Williams et al., 2001). The mechanisms related to these changes with age are poorly understood, but it was suggested that children compared to adults, display a higher potential for oxidative metabolism (McNarry and Barker, 2018). Leclair et al. (2013) found a faster ̇VO2 𝜏 and a faster oxygen extraction (deoxy-hemoglobin) as measured by near-infrared spectroscopy in children compared to adults. It appears, that the enhanced oxidative metabolism in children includes a faster oxygen delivery and a higher oxygen extraction in the exercising muscles (Leclair et al., 2013; McNarry and Jones, 2014) and this in turn results in a reduced PCr breakdown at the onset of exercise. The results in the present study are comparable to studies evaluating groups at different training status and contrary to studies evaluating the effect of age differences. Based on the findings of recent studies we assume that the results in the present study are more related to long-term training rather than age-related adaptations. In the present study the ̇VO2 response is work rate dependent, with a significantly slower 𝜏 at heavy than in moderate-intensity exercise in trained youth and adults. This results are in line with recent studies, where untrained and trained adults displayed a slowing of the 𝜏 with increasing work rate (Koppo et al., 2004). The novel finding in the present study is, that in trained youth the slowing of the 𝜏 with increasing work rate is also present. This indicates, that in trained youth the ̇VO2 response displays a similar behavior, as it was reported in adults.
LimitationsThe present study reveals novel insight in oxygen uptake kinetics of trained youth and adult cyclists. However, some limitations should be addressed. An untrained control group of youth would have been beneficial to clearly identify age-related differences. Additionally, a group of adult cyclists with a comparable training status to our group of youth cyclists would have been beneficial to examine the effect of training state. As a result of the limited number of available subjects in these categories the small sample size should be considered as a limitation. Although we applied the commonly used delta concept to ensure appropriate intensity for heavy-intensity exercise, it should be noted that the differences found should be interpreted with caution due to the suggestion that the delta concept is less secure than the use of critical power to investigate this intensity domain (Armstrong and Barker, 2009).
The results of the current study are contrary to the existing knowledge about differences in the primary response of oxygen uptake kinetics between children and/or youth and adults. Our results showed that at moderate and heavy-intensity exercise trained youth and adult cyclists displayed a similar behavior in the primary response of oxygen uptake kinetics. However, irrespective of work rate and training status, recent studies and the findings of the present study showed that the ̇VO2 gain of the primary response and end-exercise ̇VO2 gain in youth is higher than in adults, whereas adults showed a higher SC than youth cyclists. We assume that long-term training adaptations influence oxygen uptake kinetics and can be a potent mediator to reduce the age-related slowing of oxygen uptake kinetics.
ACKNOWLEDGEMENTS |
The authors would like to thank the athletes of the Austrian Cycling Federation for participating in this study. The experiments comply with the current laws of the country in which they were performed. The authors have no conflict of interest to declare. The datasets generated during and/or analyzed during the current study are not publicly available, but are available from the corresponding author who was an organizer of the study. |
|
AUTHOR BIOGRAPHY |
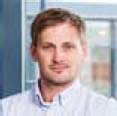 |
Bernhard Prinz |
Employment: Research Assistant, Training and Sports Sciences, University of Applied Sciences, Wiener Neustadt, AUT. |
Degree: PhD, MSc |
Research interests: Exercise physiology, cycling performance, oxygen uptake kinetics |
E-mail: bernhard.prinz@fhwn.ac.at |
|
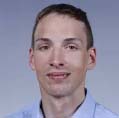 |
Manfred Zöger |
Employment: PhD student, Research Assistant, Training and Sports Sciences, University of Applied Sciences, Wiener Neustadt, AUT. |
Degree: MSc |
Research interests: Exercise physiology, cycling performance, oxygen uptake kinetics |
E-mail: manfred.zoeger2@fhwn.ac.at |
|
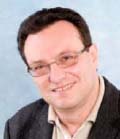 |
Harald Tschan |
Employment: Professor of Sport and Exercise Sciences, Centre for Sport Science and University Sports, University of Vienna, Wien, AUT. |
Degree: Mag. Dr. |
Research interests: Exercise science, strength and conditioning, human performance |
E-mail: harald.tschan@univie.ac.at |
|
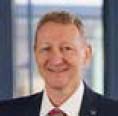 |
Alfred Nimmerichter |
Employment: Professor (Associate) of Sport and Exercise Sciences, Head of Faculty, Training and Sports Sciences, University of Applied Sciences, Wiener Neustadt, AUT. |
Degree: PhD |
Research interests: Exercise physiology, cycling performance, oxidative metabolism |
E-mail: alfred.nimmerichter@fhwn.ac.at |
|
|
|
REFERENCES |
 Armon Y., Cooper D.M., Flores R., Zanconato S., Barstow T.J. (1991) Oxygen uptake dynamics during high-intensity exercise in children and adults. Journal of Applied Physiology 70, 841-848. Crossref
|
 Armstrong N., Barker A.R. (2009) Oxygen uptake kinetics in children and adolescents: a review. Pediatric Exercise Science 21, 130-147. Crossref
|
 Barker A.R., Welsman J.R., Fulford J., Welford D., Williams C.A., Armstrong N. (2008) Muscle phosphocreatine and pulmonary oxygen uptake kinetics in children at the onset and offset of moderate intensity exercise. European Journal of Applied Physiology 102, 727-738. Crossref
|
 Beaver W.L., Wasserman K., Whipp B.J. (1986) A new method for detecting anaerobic threshold by gas exchange. Journal of Applied Physiology 60, 2020-2027. Crossref
|
 Billat V.L. (2000) VO2 slow component and performance in endurance sports. British Journal of Sports Medicine 34, 83-85. Crossref
|
 Breese B.C., Williams C.A., Barker A.R., Welsman J.R., Fawkner S.G., Armstrong N. (2010) Longitudinal change in the oxygen uptake kinetic response to heavy-intensity exercise in 14- to 16-years-old boys. Pediatric Exercise Science 22, 314-325. Crossref
|
 Caputo F., Mello M.T., Denadai B.S. (2003) Oxygen uptake kinetics and time to exhaustion in cycling and running: a comparison between trained and untrained subjects. Archives of Physiology and Biochemistry 111, 461-466. Crossref
|
 Cleuziou C., Lecoq A.M., Candau R., Courteix D., Guenon P., Obert P. (2002) Kinetics of oxygen uptake at the onset of moderate and heavy exercise in trained and untrained prepubertal children. Science and Sports 17, 291-296. Crossref
|
 Fawkner S., Armstrong N. (2003) Oxygen uptake kinetic response to exercise in children. Sports Medicine 33, 651-669. Crossref
|
 Fawkner S.G., Armstrong N. (2004) Longitudinal changes in the kinetic response to heavy-intensity exercise in children. Journal of Applied Physiology 97, 460-466. Crossref
|
 Fawkner S.G., Armstrong N., Potter C.R., Welsman J.R. (2002) Oxygen uptake kinetics in children and adults after the onset of moderate-intensity exercise. Journal of Sports Sciences 20, 319-326. Crossref
|
 Hebestreit H., Kriemler S., Hughson R.L., Bar-Or O. (1998) Kinetics of oxygen uptake at the onset of exercise in boys and men. Journal of Applied Physiology 85, 1833-1841. Crossref
|
 Ingham S.A., Carter H., Whyte G.P., Doust J.H. (2007) Comparison of the oxygen uptake kinetics of club and olympic champion rowers. Medicine and Science in Sports and Exercise 39, 865-871. Crossref
|
 Jones A.M., Carter H. (2000) The effect of endurance training on parameters of aerobic fitness. Sports Medicine 29, 373-386. Crossref
|
 Koppo K., Bouckaert J., Jones A.M. (2004) Effects of training status and exercise intensity on phase II VO2 kinetics. Medicine and Science in Sports and Exercise 36, 225-232. Crossref
|
 Leclair E., Berthoin S., Borel B., Thevenet D., Carter H., Baquet G., Mucci P. (2013) Faster pulmonary oxygen uptake kinetics in children vs adults due to enhancements in oxygen delivery and extraction. Scandinavian Journal of Medicine and Science in Sports 23, 705-712. Crossref
|
 Marwood S., Roche D., Rowland T., Garrard M., Unnithan V.B. (2010) Faster pulmonary oxygen uptake kinetics in trained versus untrained male adolescents. Medicine and Science in Sports and Exercise 42, 127-134. Crossref
|
 McNarry M., Jones A. (2014) The influence of training status on the aerobic and anaerobic responses to exercise in children: a review. European Journal of Sport Science 14, 57-68. Crossref
|
 McNarry M.A. (2019) Oxygen Uptake Kinetics in Youth: Characteristics, Interpretation, and Application. Pediatric Exercise Science 31, 175-183. Crossref
|
 McNarry M.A., Welsman J.R., Jones A.M. (2011a) The influence of training and maturity status on girls’ responses to short-term, high-intensity upper- and lower-body exercise. Applied Physiology, Nutrition, and Metabolism 36, 344-352. Crossref
|
 McNarry M.A., Welsman J.R., Jones A.M. (2011b) Influence of training status and exercise modality on pulmonary O2 uptake kinetics in pubertal girls. European Journal of Applied Physiology 111, 621-631. Crossref
|
 McNarry, M.A. and Barker, A.R. (2018) Aerobic and anaerobic metabolism. In: Elite Youth Cycling. Ed: Nimmerichter, A. Oxon: Routledge. 49-69. Crossref
|
 Nimmerichter A., Breese B.C., Prinz B., Zoeger M., Rumpl C., Williams C.A. (2020) Test-retest reliability of pulmonary oxygen uptake and muscle deoxygenation during moderate- and heavy-intensity cycling in youth elite-cyclists. Journal of Sports Sciences 38, 2462-2470. Crossref
|
 Obert P., Cleuziou C., Candau R., Courteix D., Lecoq A.M., Guenon P. (2000) The slow component of O2 uptake kinetics during high-intensity exercise in trained and untrained prepubertal children. International Journal of Sports Medicine 21, 31-36. Crossref
|
 Poole D.C., Jones A.M. (2012) Oxygen uptake kinetics. Comprehensive Physiology 2, 933-996. Crossref
|
 Powers S.K., Dodd S., Beadle R.E. (1985) Oxygen uptake kinetics in trained athletes differing in VO2max. European Journal of Applied Physiology and Occupational Physiology 54, 306-308. Crossref
|
 Rossiter H.B., Ward S.A., Kowalchuk J.M., Howe F.A., Griffiths J.R., Whipp B.J. (2002) Dynamic asymmetry of phosphocreatine concentration and O(2) uptake between the on- and off-transients of moderate- and high-intensity exercise in humans. Journal of Physiology 541, 991-1002. Crossref
|
 Unnithan V.B., Roche D.M., Garrard M., Holloway K., Marwood S. (2015) Oxygen uptake kinetics in trained adolescent females. European Journal of Applied Physiology 115, 213-220. Crossref
|
 Whipp B.J., Davis J.A., Torres F., Wasserman K. (1981) A test to determine parameters of aerobic function during exercise. Journal of Applied Physiology Respiratory, Environmental and Exercise Physiology 50, 217-221. Crossref
|
 Whipp, B.J. and Rossiter, H.B. (2005) The kinetics of oxygen uptake: physiological inferences from the parameters. In: Oxygen uptake
kinetics in sport, exercise and medicine. Eds: Jones, A.M. and Poole, D.C. Oxon: Routledge. 62-94.
|
 Williams C.A., Carter H., Jones A.M., Doust J.H. (2001) Oxygen uptake kinetics during treadmill running in boys and men. Journal of Applied Physiology 90, 1700-1706. Crossref
|
 Winlove M.A., Jones A.M., Welsman J.R. (2010) Influence of training status and exercise modality on pulmonary O(2) uptake kinetics in pre-pubertal girls. European Journal of Applied Physiology 108, 1169-1179. Crossref
|
|
|
|
|
|
|