|
|
|
ABSTRACT |
To explore the effects of 8-week polarized training (POL), high-intensity interval training (HIIT), and threshold training (THR) interventions on the cardiorespiratory fitness (CRF) of untrained healthy young adults. This study recruited 36 young adults and randomly assigned them to POL, HIIT, THR, or control (CG) groups to undergo an 8-week training intervention. The training impulse applied to all three intervention groups was identical. The training intensity was divided into Zone 1, 2, and 3 (Z1, Z2 and Z3) on the basis of the ventilatory thresholds (VT). The weekly training intensity distribution for POL was 75% of Z1 and 25% of Z3; HIIT was 100% of Z3 and THR was 50% of Z1 and 50% of Z2. Each group underwent Bruce protocol testing and supramaximal testing before, during, and after the intervention; relevant CRF parameters were assessed. 8 weeks of POL and HIIT significantly increased VT2 (p < 0.05); 8 weeks of POL, HIIT, THR and significantly increased VO2max and TTE (p < 0.05). The effect size of POL in relation to VO2max and TTE improvements was greater than that of HIIT and THR (g = 2.67 vs. 1.26 and 1.49; g = 2.75 vs. 2.05 and 1.60). Aerobic training models with different intensity distributions have different time effects on improving CRF. Relative to HIIT and THR, POL improved more variables of CRF. Therefore, POL is a feasible aerobic training method for improving CRF. |
Key words:
Ventilation threshold, Aerobic metabolism adaptation, Training intensity distribution
|
Key
Points
- The effect of POL on improving VO (12.9%) and time to exhaustion (12.0%) was greater than that of HIIT and THR.
- THR mainly comprises moderate-intensity exercises (Zones 1 and 2) and only improves aerobic metabolism (VT).
- Both POL and HIIT encompass Z3 exercises; this indicates that high-intensity training is conducive to enhancing the energy supply for aerobic metabolism and delaying complete reliance on anaerobic metabolism.
|
Improving cardiorespiratory fitness (CRF) is closely associated with improvements in personal health conditions. Numerous studies have ascertained that in both adults and adolescents (Raghuveer et al., 2020), low CRF is a risk factor for death (Ross et al., 2016), diabetes (Zaccardi et al., 2015), cancer (Schmid and Leitzmann, 2015), and other health-related diseases. Therefore, an individual must improve and maintain their CRF to maintain their health condition. The physiological benefits of exercise training stimuli are determined by the intensity and amount of training stimulation (Gormley et al., 2008). However, the optimal training combination for enhancing cardiopulmonary functions and health has yet to be determined at the time of writing. Studies have reported on the dose - response effect of exercise intensity and training amount in relation to CRF (Ross et al., 2015). Studies have indicated that aerobic training intensity is a key factor influences CRF (Laursen, 2010). When determining the exercise intensity domain of each personnel, the first and second ventilatory thresholds (VT1 and VT2) are to define three exercise intensity zones (Z1 [below VT1], Z2 [between VT1 and VT2], and Z3 [above VT2]; Lucia et al., 1999). Foster et al. (2001) proposed the application of training impulse (TRIMP; i.e., the product of training intensity and training duration) to quantify training load and design aerobic exercise with varying levels of intensity on the basis of exercise intensity zones. Studies have revealed that most people performed high-intensity interval training (HIIT), moderate-intensity continuous training (MICT), or threshold training (THR) in Z3, Z2, or Z1 - Z2, respectively, and attained satisfactory results (Macpherson et al., 2011; Muñoz et al., 2014). Studies have suggested incorporating a combination of high-volume low-intensity training and low-volume high-intensity training into programs for endurance athletes who require high training loads. This training method is called polarized training (POL). POL is conducive to improving and maintaining CRF, and it can reduce the risk of overtraining. For the training intensity distribution of POL, 75-80%, 0-10%, and 15-20% of training are spent in Z1, Z2, and Z3, respectively (Carnes and Mahoney, 2018; Muñoz et al., 2014; Pérez et al., 2018). Studies have verified that POL can improve the CRF and other exercise-related attributes of professional and recreational endurance runners and provide superior effects to those of other training interventions (Carnes and Mahoney, 2018; Muñoz et al., 2014; Stöggl and Sperlich, 2014). However, some studies did fail to find a CRF improvement after POL intervention (Festa et al., 2020; Pérez et al., 2018; Treff et al., 2017), scholars have indicated that the effects of POL training on maximal oxygen intake (VO2max) and time to exhaustion (TTE) are not significantly greater than those of HIIT (Stöggl and Sperlich, 2014) or THR (Festa et al., 2020; Pérez et al., 2018). The inconsistency in literature findings may be related to the limitations of the experiment adopted in each study, such as the ceiling effect. For example, if participants only comprise professional or recreational athletes (Festa et al., 2020; Muñoz et al., 2014; Neal et al., 2013; Pérez et al., 2018; Stöggl and Sperlich, 2014) with existing high training loads and excellent physical conditions, the effect of POL on CRF and other relevant variables may be nonsignificant. The above-mentioned conflicting results regarding the effectiveness and definition of POL intensity distribution for endurance athletes are subject to ongoing discussions. (Burnley et al., 2022a; Burnley et al., 2022b; Foster et al., 2022a; Foster et al., 2022b). Furthermore, most of the studies that observed the effects of different training intensity distributions have conducted experiments that focused on the distribution ratio and have not considered experiments in which TRIMP was kept as a constant (Carnes and Mahoney, 2018; Neal et al., 2013; Stöggl and Sperlich, 2014). Other studies have employed the number of sessions, distance covered, and duration of training to quantify training volume (Treff et al., 2017); however, how varying intensity affects TRIMP has been ignored. The aforementioned factors could have resulted in POL and other training methods being unsuitable for comparison. Therefore, the present study designed training programs with different intensity distributions but identical TRIMP. Additionally, supramaximal testing was conducted to verify the effect of POL, HIIT, and THR on CRF.
Participant characteristicsThis study recruited 36 untrained young adults. After the study procedure was explained, the participants signed informed consent forms. The participants were required to (1) maintain their regular lifestyle and dietary habits and (2) not to perform additional exercise training. The experiment was implemented with the approval of the Fu Jen Catholic University Institutional Review Board (No. C107083).
Study designThe present study was an 8-week, parallel-group, randomized controlled trial designed to compare the CRF improvements resulting from various training intensity distributions with identical TRIMP (180 TRIMP/week) (Figure 1). The 36 participants were randomly assigned to the control group (CG), POL, HIIT, or THR group (Table 1). Each participant, except for the control group, completed three training sessions weekly. Their CRF was evaluated before (pre-test), during (mid-test), and after (post-test) the intervention. Training intensity was adjusted individually after week 5.
CRF testingThe participants were instructed to refrain from overeating 2 h before exercise testing and to familiarize themselves with the procedures of the treadmill test. The same familiarization testing procedures and time of day (± 2 h) were applied for all tests and participants. The participants’ VO2max, first ventilation threshold (VT1), and second ventilation threshold (VT2) during maximal exercise testing were analyzed (Metalyzer 3B, Cortex, Leipzig, Germany). The Bruce protocol was used to assess CRF. The initial slope and speed of the treadmill were 10% and 2.7 km/h, respectively; every 3 min, the slope and speed were increased by 2% and 1.4 km/h, respectively. The participants ran continuously until exhaustion. The Borg rating of perceived exertion (RPE) scale was used to measure exertion. The participants wore heart rate monitors (H10, Polar Electro Oy, Kempele, Finland) during the testing process. VO2max (average of 15 s) was defined as a plateau in VO2 with a deviation ≤ 2.0 mL/kg-1/min-1. However, if a VO2 plateau was not observed, then at least two of the following conditions must be met to determine VO2max: (1) respiratory exchange ratio (RER) ≥ 1.10; (2) ± 10 bpm of age-predicted maximum heart rate (HRmax = 208-0.7 × age); and (3) RPE ≥ 18. VT1 and VT2 were used as cut-points for personalizing training intensities. To determine VT1 and VT2, 10-s averages of oxygen intake were obtained during the CRF test. Two independent observers, who were experienced in exercise physiology, blindly identified VT1 and VT2. In case of disagreement, the opinion of a third researcher was sought to reach a consensus. VT1 was identified based on the criteria of an increase in VE/VO2 with VE increasing non-linearly in addition to the nonconcurrent increase in VE/VCO2. VT2 was identified based on the concurrent increase in VE/VO2 and VE/VCO2.
Supramaximal testingTo verify the accuracy of the CRF data, this study employed supramaximal testing after the participants had completed the CRF test and rested for 20-60 min (Nolan et al., 2014). During the rest period, participants who perceived themselves to be fully rested were allowed to start the supramaximal test. The test included a warm-up phase with 2 min of running at a 50% workload and 1 min of running at a 70% workload; for the supramaximal testing, a 105% workload (computed from the final stage of CRF testing) was applied, and a participant continued until exhaustion (Astorino et al., 2009). The criteria for exhaustion and method of data acquisition were identical to those used for the CRF test.
Maximal aerobic speed testThe initial speed and slope for maximal aerobic speed testing was 8 km/h and 1%, respectively. The speed for the test was progressively increased by 1 km/h every 2 min while the slope was maintained at 1%, and testing was conducted until a participant was exhausted (Machado et al., 2013). The indicators used to determine exercise exhaustion were (1) failure to keep up with an applied speed, (2) having a HRmax that was within (208-0.7 × age) ± 10, and (3) having an RPE of >18. The participants’ relative running speed and heart rate (HR) for each intensity zone during the intervention were determined.
8-week Training InterventionControl group
The participants in the control group did not receive any form of training intervention throughout the experiment period. The participants were required to maintain their regular lifestyles and avoid engaging in additional activities and exercises.
HIIT groupThe training intensity distribution of the HIIT only comprised Z3 (Figure 2). Three sessions per week were conducted, with each training session lasted 20 min and comprised five sets of 2-min Z3 exercises (90% VO2max) separated by 2-min active rest (≥VT2).
POL groupThe training intensity distribution of the POL was 75% and 25% of training time spent in Z1 and Z3 (Figure 2), respectively. Three sessions per week were conducted, each training session lasted 40 min and comprised 30 min of Z1 (95% VT1) and 10 min of Z3 (five sets of 1-min Z3 exercises [90% VO2max] separated by 1-min active rest [≥ VT2]).
THR groupThe training intensity distribution of the THR was 50% of training time spent in Z1 and the other 50% spent in Z2 (Figure 2). Three sessions per week were conducted, each training session lasted 40 min and comprised 20 min of Z1 (95% VT1) and 20 min of Z2 (50% - 95% VT2).
Exercise intensity monitoringPrior to each training session, each participant wore a heart rate monitor (OH1, Polar Electro Oy, Kempele, Finland). The applied training speed was adjusted for each participant to reach the targeted HR zone. If a participant’s HR deviated from the target zone corresponding to their target exercise intensity (Z1, Z2 or Z3), the instructor made minor speed adjustments (≤0.5 km/h) to maintain their HR within the target zone. Additionally, if the participant’s RPE or average HR (5-10 bpm) decreased over 2 consecutive weeks of training (Zapata-Lamana et al., 2018), the initial speed was increased by 0.5 km/h for the subsequent training session to maintain the training load.
Statistical analysisThe demographic characteristics and testing data were presented as mean (M) and standard deviations (SD). The percentage of changes in the CRF variables (%∆) was calculated using the following equation: ([post-test - pre-test]/pre-test) × 100%. The results were referred to the third researcher in the case of conflicting results. SPSS 28.0 (SPSS, Chicago, IL, USA) was employed for statistical analysis. A mixed-design two-way analysis of variance with Bonferroni post hoc analysis was used to test for differences (groups [CG, HIIT, POL, and THR] × weeks [weeks 0, 4, and 8]). An independent Student’s t-test was performed to compare percentage of maximal heart rate (%HRmax) in Z1 and Z3 at week 8 among groups. Statistical significance was set at α = .05. Finally, the corrected effect size (ES) and Hedges’ g (g) values were used to measure the effect of the interventions. The following threshold for ES were employed: <0.2 = trivial, >0.2 = small, >0.6 = moderate, >1.2 = large, >2.0 = very large, and >4.0 = extremely large (Hopkins et al., 2009).
Actual intensity of various training distributionsThree 8-week aerobic training programs that had different training intensity distributions but shared the same TRIMP were implemented. In particular, the %HRmax differences between the POL and THR in Z1 were nonsignificant (67.1% ± 4.1% vs. 65.6% ± 3.9%, t = 0.809, p = .431). The %HRmax differences between the HIIT and POL in Z3 were nonsignificant (92.5% ± 2.2% vs. 91.8% ± 1.2%, t = 0.904, p = .380). Details of the %HRmax of the 3 training intervenetion are presented in Figure 3.
Variables of CRFPost hoc comparison revealed that the VO2max for POL, HIIT, and THR on the posttest was significantly higher after the intervention than before (p = 0.012, 0.001 and 0.005). The VO2max for POL was significantly higher after the intervention than during the intervention (p = 0.006), and the value during the intervention was significantly higher than that before (p = 0.004). The HRmax for POL, HIIT, and THR was significantly lower during the intervention than before (p = 0.030, 0.006 and 0.044). VO2 at VT1 was significantly higher during the intervention than before it in the THR group (p = 0.015). VO2 at VT2 for HIIT and POL was significantly higher after the intervention than during (p = 0.041 and 0.026) or before (p = 0.036 and < 0.001). Furthermore, VO2 at VT2 for POL was significantly higher during the intervention than before (p = 0.037). TTE was significantly higher after and during the intervention than before in all three groups (p < 0.05). In the POL and THR, TTE was also significantly higher after the intervention than during (p = 0.002 and 0.017). VO2max, VT1, VT2, and TTE did not significantly differ among the groups, and all of these CRF variables were more favorable than those of the CG group after the intervention (p < 0.05). In addition, post hoc comparison revealed that the VEmax was significantly higher after the intervention than before in the HIIT and POL groups (p = 0.034 and 0.017). In addition, RERmax was significantly lower during the intervention than before in POL, HIIT, and THR groups (p = 0.004, 0.009 and 0.006); in the THR and HIIT groups, RERmax was significantly lower after the intervention than before (p = 0.011 and < 0.001; Figure 4). All participants, except for the CG, exhibited significant improvements in VO2max, but only POL produced a large ES after 4 weeks of training (g = 1.56). After 8 weeks of training, HIIT and THR also increased VO2max with a large ESs (g = 1.26 and 1.49), and POL substantially increased VO2max, with an extremely large ES (g = 2.67). In addition, after 4 weeks of training, POL improved TTE with an extremely large ES (g = 2.20). Finally, after 8 weeks, HIIT and POL improved TTE with extremely large ESs (g = 2.05 and 2.75), and THR improved TTE with a large ES (g = 1.60; Table 2).
The present study tested the effects of training intensity distribution on CRF, obtaining the following findings: (1) 4 weeks of THR intervention significantly improved VT1, and 8 weeks of HIIT and POL interventions significantly improved VT2; (2) 8 weeks of POL, HIIT, and THR interventions significantly improved VO2max and TTE; and (3) 8 weeks of POL produced more favorable effects (ESs) on CRF than did HIIT or THR. These results support the hypothesis that 8 weeks of POL, HIIT, and THR interventions at an equalized TRIMP can improve the CRF of healthy young adults. Furthermore, the intervention time required to achieve a significant CRF improvement differed among the three groups. The TRIMP of each training distribution was the same and based on the ACSM’s guidelines, which recommend 20-40 min of exercise to improve CRF in the general population (American College of Sports Medicine, 2021). The overall training load is crucial to CRF improvement. Studies have reported that differences in the overall training loads of training distributions can confound effects on CRF or exercise performance (Stöggl and Sperlich, 2014; Zapata-Lamana et al., 2018) and limit the subsequent interpretation of results. The present study tested various training distributions with the same TRIMP and revealed nonsignificant differences in the Z1 %HRmax achieved through POL and THR and nonsignificant differences in the Z3 %HRmax achieved through POL and HIIT at week 8. Therefore, the same overall training load resulted in a similar physical load for the various training distributions. The physical fitness level of participants may confound the results of others studies of POL for CRF improvement. Pérez et al. (2018) recruited ultramarathon runners as participants and discovered that the VO2max did not significantly increase after 12 weeks of POL training (55.8 vs. 54.9 mL/kg/min). Treff et al. (2017) recruited national team rowers as participants and discovered that the VO2max did not significantly increase after 11 weeks of POL training (68 vs. 68 mL/kg/min; ∆0.6%). Festa et al. (2020) compared the pre- and post-intervention performance of leisure runners after 8 weeks of POL training and could not identify any improvements in VO2max (53.0 vs. 53.6 mL/kg/min). The aforementioned studies indicate that a ceiling effect could influence the results of POL training for participants with high physical fitness. However, 12 weeks of POL intervention was shown to significantly increase the VO2peak of women with overweight or obesity (Zapata-Lamana et al., 2018). In the present study, 8 weeks of POL intervention significantly improved the VO2max of untrained young adults (+12.9%). In summary, differences in fitness level may be a key influencing factor for intervention effects. Therefore, researchers must practice caution when interpreting the effect of a POL intervention and implementing it in a practical setting. Given the potential ceiling effect, indicators other than VO2max (e.g., VT1 and VT2) should be and examined to clarify the effects of POL on CRF. In the present study, 8 weeks of HIIT or POL significantly increased VT2. However, this effect was not achieved by THR, which could be attributed to the lack of high-intensity (Z3) training in THR; by contrast, POL and HIIT incorporate Z3 training. In a previous study, the increase in VT2 achieved through HIIT was greater than that through MICT (Schaun et al., 2018), indicating that high-intensity training helps to improve aerobic metabolism efficiency and delay anaerobic metabolism. Another study reported that VT2 increases are related to the capacity for performing long-duration, high-intensity aerobic exercises (Bunc et al., 1987). In the present study, POL (at the same TRIMP) increased VT2 while requiring shorter high-intensity training relative to HIIT. This finding provides the untrained population (or even athletes) with an aerobic training model that can reduce the risk of overuse posed by excessive training intensity. The present study revealed that HRmax was significantly lower during all three interventions than before. The underlying mechanism of HRmax alteration remains unclear (Whyte et al., 2008; Zavorsky, 2000), but Zavorsky (2000) suggested numerous explanations, including plasma volume expansion, enhanced baroreflex function, changes to the sinoatrial node, and a reduced number and density of ß-adrenergic receptors. Kenney et al. (2019) suggested that HRmax changes occur because aerobic endurance training increases the time required for ventricular refilling, which increases stroke volume, thereby reducing HRmax. However, the causal relationship between aerobic training and HRmax remains unclear. Regarding the effect of aerobic training on the variables of CRF, the results revealed 4 weeks of POL, HIIT, and THR interventions significantly reduced the RERmax. In particular, the RERmax of the POL and HIIT were significantly lower than CG. Evidence suggests that fat oxidation provides energy during short, intense exercise sessions (Essén-Gustavsson and Tesch, 1990; McCartney et al., 1986). This could be the cause of the decrease in RERmax. Messonnier et al. (2005) reported that 4 weeks of aerobic training significantly reduced the RERmax of healthy sedentary adults, enhanced fat metabolism adaptations, increased oxidizing enzyme activity and O2 intake, and increased TTE. After 8 weeks of POL, HIIT, or THR intervention, TTE significantly increased and was more favorable than that of the CG group. In particular, the ES of HIIT and POL in increasing TTE were greater than that of THR (g = 2.05 [HIIT] and 2.75 [POL] vs. 1.60 [THR]). The present study surmised that the changes in RERmax induced by 4 weeks of aerobic training could reflect the changes to the substrates of energy metabolism. In particular, the present study proposes that these improvements can be enhanced by incorporating high-intensity training (e.g., POL and HIIT) into the training program design. After 4 weeks of HIIT training, VEmax significantly improved, and this improvement maintained throughout the intervention. By contrast, the VO2max of the POL group improved only by the end of the intervention; this could be caused by the group’s high percentage of Z1 training (75%) and smaller amount of Z3 training stimulus. Accordingly, the present study inferred that the lack of Z3 training for the THR group resulted in its VEmax improving only in the first 4 weeks (weeks 1–4) and returning to baseline by the end of the intervention. During high-intensity exercise, lactate and H+ levels increase, stimulating the respiratory control center to increase VE and accelerate CO2 expiration. In the final 4 weeks (weeks 5-8), only the POL group exhibited a significant increase in VCO2max, with a 3.9% increase in VEmax (moderate ES). Regular exercise enhances the sensitivity of VCO2 chemoreceptors (Yamashiro et al., 2021). One study revealed an increase in VE during the supramaximal state of sprint interval training (SIT), during which the maximal reductions in muscle and blood acidity were achieved, resulting in increased VCO2 (Wood et al., 2016). In the present study, the Z3 work–rest ratio for POL was similar to that applied for the aforementioned SIT intervention. Accordingly, we speculated that POL considerably improved ventilation efficiency in the final 4 weeks because of the adaptations from Z3, which increased the ventilatory CO2 and reduced its acidity, resulting in a longer TTE (g = 2.75). The improvements in the CRF of untrained young adults were similar among three exercise intervention groups. Other studies did not identify an optimal training distribution for improving CRF. Therefore, the present study examined ES to clarify the effects of the training distributions. The ESs of the improvements in VO2max (g = 2.67) and TTE (g = 2.75) in the POL group were greater than those in the HIIT (g = 1.26 [VO2max] and 2.05 [TTE]) and THR (g = 1.49 [VO2max] and 1.60 [TTE]) groups after 8 weeks of training. This result is similar to that of Carnes and Mahoney (2018), who revealed POL intervention had an ES (Cohen’s d = 0.85) that was greater than CrossFit endurance training. Similarly, Zapata-Lamana et al. (2018) reported that the ES of POL was greater than HIIT and MICT (g = 2.6 [POL] vs. 2.1 [HIIT] vs. 1.5 [MICT]). In the ongoing debate about the effectiveness of polarized training, Burnley et al. (2022a) emphasized the importance of Z2, which is supported by our finding that training in THR significantly impacted VT1 and improved aerobic capacity. However, we intentionally excluded Z2 training from POL. Our findings suggest that incorporating both Z1 and Z3 components is crucial for CRF improvements, as the absence of Z3 may limit its enhancement. As noted by Foster et al. (2022a), a study (Treff et al., 2017) that "clamped" the Z1 training volume at 93% showed little difference in improved performance, underscoring the importance of incorporating Z3 training. Laursen (2010) has suggested the possible explanation was that the molecular signaling pathways for the low-intensity (Z1) and high-intensity (Z3) components of endurance training induce the same metabolic adaptation, that is, CRF improvement. However, both pathways involve the activation of a key aerobic adaptation factor: PGC-1α. The POL intervention in the present study incorporated both intensity levels, and this could have resulted in POL achieving a greater ES than HIIT or THR on VO2max. In addition, relative to implementing only SIT or endurance exercises, a combination of SIT and endurance exercises induces greater PGC-1α mRNA expression (Skovgaard et al., 2016). Studies have reported that high-volume low-intensity training (Z1) improves CRF by increasing cardiac output and plasma volume, inducing adaptations pertaining to capillary and mitochondrial biogenesis and enhancing the efficiency of key metabolic processes (Midgley et al., 2006; Romijn et al., 1993; Stöggl and Sperlich, 2014). Other studies have proposed that short-term high-intensity training (Z3) activate PGC-1α expression, thereby promoting mitochondrial synthesis, which leads to adaptations of the cardiovascular (central) and musculoskeletal (peripheral) systems (MacInnis & Gibala, 2017; Torma et al., 2019) and enhances the oxygen utilization efficiency of peripheral tissues (Macpherson et al., 2011). Additionally, Z1 training helps to stabilize hormone levels, prevent overuse, and compensate for the strenuous training load of Z3 (Hill et al., 2008). In summary, POL, which comprises both Z1 and Z3 training, creates an optimal environment for mitochondrial biogenesis and cardiovascular adaptation and ultimately improves CRF. This result suggests that individuals with poor CRF can benefit from POL training with a low high-intensity training load (Z3) and achieve CRF improvements that are similar to those achieved through HIIT while reducing the risks of poor recovery and overuse.
With TRIMP being a fixed constant, the effects of different training intensity distributions were compared. The effect of POL on CRF is similar to that of HIIT and THR; however, POL requires a lower high-intensity training load. In the present study, confounding factors (e.g., fitness levels and training impulse) were accounted for. Additionally, supramaximal testing was performed to verify the accuracy of CRF testing and to clarify the benefits of aerobic training with different training intensity distributions. Results indicated that the different training intensity distributions have different time effects on CRF improvement. The findings suggest that POL is effective aerobic training for improving CRF in untrained healthy young adults. Furthermore, POL can help individuals to maintain their training volume, recover from fatigue, and avoid overuse.
ACKNOWLEDGEMENTS |
We thank all participants for devoting their time to this study. This work was supported by Chinese Culture University and National Science and Technology Council [grant numbers 1082410H034047, 2019]. The experiments comply with the current laws of the country in which they were performed. The authors have no conflict of interest to declare. The datasets generated and analyzed during the current study are not publicly available, but are available from the corresponding author who was an organizer of the study. |
|
AUTHOR BIOGRAPHY |
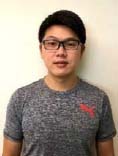 |
Tsung-Lin Chiang |
Employment: Graduate Institute of Sport Coaching Science, Chinese Culture University, Taipei City, Taiwan (R.O.C.) |
Degree: PhD |
Research interests: Strength and conditioning, measurement and evaluation, aerobic capacity performance, metabolic energy |
E-mail: asd7251270@gmail.com |
|
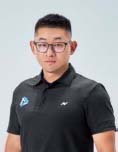 |
Chu Chen |
Employment: Lab of Progression and Perfection Sport Science, Taipei, Taiwan (R.O.C.) |
Degree: Ph.D. candidate |
Research interests: Strength and conditioning, athletic performance, speed and agility |
E-mail: alvinc5939@gmail.com |
|
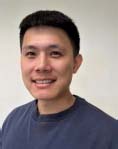 |
Yu-Chin Lin |
Employment: Graduate Institute of Sport Coaching Science, Chinese Culture University, Taipei City, Taiwan (R.O.C.) |
Degree: PhD |
Research interests: Examining the influence of various acute and chronic stressors on biomechanical and neuromuscular function. |
E-mail: yujimlin@gmail.com |
|
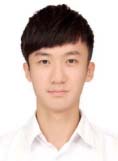 |
Shih-Hsuan Chan |
Employment: Graduate Institute of Sport Coaching Science, Chinese Culture University, Taipei City, Taiwan (R.O.C.) |
Degree: MS |
Research interests: Strength and conditioning, sport performance, muscle hypertrophy, muscular adaptations |
E-mail: csh010404@gmail.com |
|
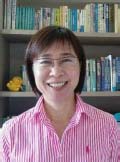 |
Huey-June Wu |
Employment: Graduate Institute of Sport Coaching Science, Chinese Culture University, Taipei City, Taiwan (R.O.C.) |
Degree: PhD |
Research interests: Sports training, sports performance, exercise physiology |
E-mail: wuhc0123@gmail.com |
|
|
|
REFERENCES |
 American College of Sports Medicine. (2021) ACSM's guidelines for exercise testing and prescription. 11th edition. Philadelphia: American College of Sports Medicine.
|
 Astorino T. A., White A. C., Dalleck L. C. (2009) Supramaximal testing to confirm attainment of VO in sedentary men and women. International Journal of Sports Medicine 30, 279-284. Crossref
|
 Bunc V., Heller J., Leso J., Sprynarová S., Zdanowicz R. (1987) Ventilatory threshold in various groups of highly trained athletes. International Journal of Sports Medicine 8, 275-280. Crossref
|
 Burnley M., Bearden S. E., Jones A. M. (2022a) Polarized training is not optimal for endurance athletes. Medicine and Science in Sports and Exercise 54, 1032-1034. Crossref
|
 Burnley M., Bearden S. E., Jones A. M. (2022b) Polarized training is not optimal for endurance athletes: response to foster and colleagues. Medicine and Science in Sports and Exercise 54, 1038-1040. Crossref
|
 Carnes A. J., Mahoney S. E. (2018) Polarized vs. high intensity multimodal training in recreational runners Submission. International Journal of Sports Physiology and Performance 14, 105-112. Crossref
|
 Essén-Gustavsson B., Tesch P. A. (1990) Glycogen and triglyceride utilization in relation to muscle metabolic characteristics in men performing heavy-resistance exercise. European Journal of Applied Physiology and Occupational Physiology 61, 5-10. Crossref
|
 Festa L., Tarperi C., Skroce K., La Torre A., Schena F. (2020) Effects of different training intensity distribution in recreational runners. Frontiers in Sports and Active Living 1, 70. Crossref
|
 Foster C., Casado A., Esteve-Lanao J., Haugen T., Seiler S. (2022a) Polarized training is optimal for endurance athletes. Medicine and Science in Sports and Exercise 54, 1028-1031. Crossref
|
 Foster C., Casado A., Esteve-Lanao J., Haugen T., Seiler S. (2022b) Polarized training is optimal for endurance athletes: response to burnley, bearden, and jones. Medicine and Science in Sports and Exercise 54, 1035-1037. Crossref
|
 Foster C., Florhaug J. A., Franklin J., Gottschall L., Hrovatin L. A., Parker S., Doleshal P., Dodge C. (2001) A new approach to monitoring exercise training. The Journal of Strength and Conditioning Research 15, 109-115. Crossref
|
 Gormley S. E., Swain D. P., High R., Spina R. J., Dowling E. A., Kotipalli U. S., Gandrakota R. (2008) Effect of intensity of aerobic training on VO. Medicine and Science in Sports and Exercise 40, 1336-1343. Crossref
|
 Hill E. E., Zack E., Battaglini C., Viru M., Viru A., Hackney A. C. (2008) Exercise and circulating cortisol levels: the intensity threshold effect. Journal of Endocrinological Investigation 31, 587-591. Crossref
|
 Hopkins W., Marshall S., Batterham A., Hanin J. (2009) Progressive statistics for studies in sports medicine and exercise science. Medicine and Science in Sports and Exercise 41, 3. Crossref
|
 Kenney, W. L., Wilmore, J. H. and Costill, D. L. (2019) Physiology of sport and exercise. 7th edition. Champaign: Human Kinetics.
|
 Laursen P. B. (2010) Training for intense exercise performance: high-intensity or high-volume training?. Scandinavian Journal of Medicine and Science in Sports 20, 1-10. Crossref
|
 Lucia A., Hoyos J., Carvajal A., Chicharro J. L. (1999) Heart rate response to professional road cycling: the Tour de France. International Journal of Sports Medicine 20, 167-172. Crossref
|
 Machado F. A., Kravchychyn A. C. P., Peserico C. S., da Silva D. F., Mezzaroba P. V. (2013) Effect of stage duration on maximal heart rate and post-exercise blood lactate concentration during incremental treadmill tests. Journal of Science and Medicine in Sport 16, 276-280. Crossref
|
 MacInnis M. J., Gibala M. J. (2017) Physiological adaptations to interval training and the role of exercise intensity. The Journal of Physiology 595, 2915-2930. Crossref
|
 Macpherson R. E., Hazell T. J., Olver T. D., Paterson D. H., Lemon P. W. (2011) Run sprint interval training improves aerobic performance but not maximal cardiac output. Medicine and Science in Sports and Exercise 43, 115-122. Crossref
|
 McCartney N., Spriet L. L., Heigenhauser G. J., Kowalchuk J. M., Sutton J. R., Jones N. L. (1986) Muscle power and metabolism in maximal intermittent exercise. Journal of Applied Physiology 60, 1164-1169. Crossref
|
 Messonnier L., Denis C., Prieur F., Lacour J. R. (2005) Are the effects of training on fat metabolism involved in the improvement of performance during high-intensity exercise?. European Journal of Applied Physiology 94, 434-441. Crossref
|
 Midgley A. W., McNaughton L. R., Carroll S. (2006) Verification phase as a useful tool in the determination of the maximal oxygen uptake of distance runners. Applied Physiology, Nutrition, and Metabolism 31, 541-548. Crossref
|
 Muñoz I., Seiler S., Bautista J., España J., Larumbe E., Esteve-Lanao J. (2014) Does polarized training improve performance in recreational runners?. International Journal of Sports Physiology and Performance 9, 265-272. Crossref
|
 Neal C. M., Hunter A. M., Brennan L., O'Sullivan A., Hamilton D. L., DeVito G., Galloway S. D. (2013) Six weeks of a polarized training-intensity distribution leads to greater physiological and performance adaptations than a threshold model in trained cyclists. Journal of Applied Physiology 114, 461-47. Crossref
|
 Nolan P. B., Beaven M. L., Dalleck L. (2014) Comparison of intensities and rest periods for VO verification testing procedures. International Journal of Sports Medicine 35, 1024-1029. Crossref
|
 Pérez A., Ramos-Campo D. J., Freitas T. T., Rubio-Arias J. Á., Marín-Cascales E., Alcaraz P. E. (2018) Effect of two different intensity distribution training programmes on aerobic and body composition variables in ultra-endurance runners. European Journal of Sport Science 19, 1-9. Crossref
|
 Raghuveer G., Hartz J., Lubans D. R., Takken T., Wiltz J. L., Mietus-Snyder M., Mietus-Snyder M., Perak A. M., Baker-Smith C., Pietris N., Edwards N. M. (2020) Cardiorespiratory fitness in youth: an important marker of health: a scientific statement from the American heart association. Circulation 142, 101-118. Crossref
|
 Romijn J. A., Coyle E. F., Sidossis L. S., Gastaldelli A., Horowitz J. F., Endert E., Wolfe R. R. (1993) Regulation of endogenous fat and carbohydrate metabolism in relation to exercise intensity and duration. American Journal of Physiology-Endocrinology and Metabolism 265, 380-391. Crossref
|
 Ross R., Blair S. N., Arena R., Church T. S., Després J. P., Franklin B. A., Haskell W. L., Kaminsky L. A., Levine B. D., Lavie C. J., Wisløff U. (2016) Importance of assessing cardiorespiratory fitness in clinical practice: a case for fitness as a clinical vital sign: a scientific statement from the American Heart Association. Circulation 134, 653-699. Crossref
|
 Ross, R., de Lannoy, L. and Stotz, P. J. (2015) Separate effects of intensity
and amount of exercise on interindividual cardiorespiratory fitness response. Mayo Clinic Proceedings 90, 1506-1514. Crossref
|
 Schaun G. Z., Pinto S. S., Silva M. R., Dolinski D. B., Alberton C. L. (2018) Whole-body high-intensity interval training induce similar cardiorespiratory adaptations compared with traditional high-intensity interval training and moderate-intensity continuous training in healthy men. The Journal of Strength & Conditioning Research 32, 2730-2742. Crossref
|
 Schmid D., Leitzmann M. F. (2015) Cardiorespiratory fitness as predictor of cancer mortality: a systematic review and meta-analysis. Annals of Oncology 26, 272-278. Crossref
|
 Skovgaard C., Brandt N., Pilegaard H., Bangsbo J. (2016) Combined speed endurance and endurance exercise amplify the exercise-induced PGC-1α and PDK4 mRNA response in trained human muscle. Physiological Reports 4, e12864. Crossref
|
 Stöggl T., Sperlich B. (2014) Polarized training has greater impact on key endurance variables than threshold, high intensity, or high volume training. Frontiers in Physiology 5, 33. Crossref
|
 Torma F., Gombos Z., Jokai M., Takeda M., Mimura T., Radak Z. (2019) High intensity interval training and molecular adaptive response of skeletal muscle. Sports Medicine and Health Science 1, 24-32. Crossref
|
 Treff G., Winkert K., Sareban M., Steinacker J. M., Becker M., Sperlich B. (2017) Eleven-week preparation involving polarized intensity distribution is not superior to pyramidal distribution in national elite rowers. Frontiers in Physiology 8, 515. Crossref
|
 Whyte G. P., George K., Shave R., Middleton N., Nevill A. M. (2008) Training induced changes in maximum heart rate. International Journal of Sports Medicine 29, 129-133. Crossref
|
 Wood K. M., Olive B., LaValle K., Thompson H., Greer K., Astorino T. A. (2016) Dissimilar physiological and perceptual responses between sprint interval training and high-intensity interval training. The Journal of Strength and Conditioning Research 30, 244-250. Crossref
|
 Yamashiro S. M., Kato T., Matsumoto T. (2021) Altered chemosensitivity to CO2 during exercise. Physiological Reports 9, e14882. Crossref
|
 Zaccardi F., O'Donovan G., Webb D. R., Yates T., Kurl S., Khunti K., Davies M. J., Laukkanen J. A. (2015) Cardiorespiratory fitness and risk of type 2 diabetes mellitus: a 23-year cohort study and a meta-analysis of prospective studies. Atherosclerosis 243, 131-137. Crossref
|
 Zapata-Lamana R., Henríquez-Olguín C., Burgos C., Meneses-Valdés R., Cigarroa I., Soto C., Fernández-Elías V. E., García-Merino S., Ramirez-Campillo R., García-Hermoso A., Cerda-Kohler H. (2018) Effects of polarized training on cardiometabolic risk factors in young overweight and obese women: A randomized-controlled trial. Frontiers in physiology 9, 1287. Crossref
|
 Zavorsky G. S. (2000) Evidence and possible mechanisms of altered maximum heart rate with endurance training and tapering. Sports Medicine 29, 13-26. Crossref
|
|
|
|
|
|
|