|
|
|
ABSTRACT |
The onset of fatigue disrupts the functioning of the autonomic nervous system (ANS), potentially elevating the risk of life-threatening incidents and impairing daily performance. Previous studies mainly focused on physical fatigue (PF) and mental fatigue (MF) effects on the ANS, with limited knowledge concerning the influence of physical-mental fatigue (PMF) on ANS functionality. This study aimed to assess the immediate impact of PMF on ANS function and to compare its effects with those of PF and MF on ANS function. Thirty-six physically active college students (17 females) without burnout performed 60-min cycling exercises, AX-Continuous Performance Task (AX-CPT), and cycling combined with AX-CPT to induce PF, MF, and PMF respectively. Subjective fatigue levels were measured using the Rating of Perceived Exertion scale and the Visual Analog Scale-Fatigue. Heart rate variability was measured before and after each protocol to assess cardiac autonomic function. The proposed tasks successfully induced PF, MF, and PMF, demonstrated by significant changes in subjective fatigue levels. Compared with baseline, PMF decreased the root mean square of successive differences (RMSSD) between normal heartbeats (P < 0.001, d = 0.50), the standard deviation of normal-to-normal RR intervals (SDNN) (P < 0.01, d = 0.33), and the normalized high-frequency (nHF) power (P < 0.001, d = 0.32) while increased the normalized low-frequency (nLF) power (P < 0.001, d = 0.35) and the nLF/nHF ratio (P < 0.001, d = 0.40). Compared with MF, PMF significantly decreased RMSSD (P < 0.001, η2 = 0.431), SDNN (P < 0.001, η2 = 0.327), nLF (P < 0.01, η2 = 0.201), and nHF (P < 0.001, η2 = 0.377) but not the nLF/nHF ratio. There were no significant differences in ∆HRV (i.e., ∆RMSSD, ∆SDNN, ∆nLF/nHF, ∆nLF, and ∆nHF), heart rate, and training impulse between PF- and PMF-inducing protocols. Cognitive performance (i.e., accuracy) in AX-CPT during the PMF-inducing protocol was significantly lower than that during the MF-inducing protocol (P < 0.001, η2 = 0.101). PF and PMF increased sympathetic activity and decreased parasympathetic activity, while MF enhanced parasympathetic activity. |
Key words:
Physical fatigue, mental fatigue, physical-mental fatigue, heart rate variability, cardiac autonomic activity
|
Key
Points
- The study examines how combined physical and mental fatigue (PMF) affects the autonomic nervous system, contrasting its impact with physical (PF) or mental fatigue (MF) alone.
- PMF significantly reduced parasympathetic and increased sympathetic nervous system markers.
- PMF had a greater impact on autonomic function than MF alone, similar to PF.
- Cognitive abilities were more adversely affected by PMF than by MF alone.
|
Fatigue is a phenomenon that dysregulates physiological systems, including the autonomic nervous system (ANS) (Tanaka et al., 2011; Yoshiuchi et al., 2004). Heart rate variability (HRV), reflecting ANS activity, serves as a potential biomarker for fatigue (Escorihuela et al., 2020; Matuz et al., 2022; Ni et al., 2022). Compared to individuals with lower levels of physical activity, fatigue may exhibit distinctive HRV characteristics in physically active individuals, attributed to their unique physiological adaptations and response patterns to physical stress (Hegde et al., 2018). Physically active individuals present a prime model for studying these changes as their HRV responses are indicative of their ANS's adaptability to stress and resilience to fatigue (Marcora et al., 2009). Understanding these responses in a physically active population can provide insights into optimizing training regimens and recovery strategies (Seiler et al., 2007). In addition, Fatigue-induced alterations in ANS activity increase the risk of life-threatening events and decrease performance in daily activities (Marcora et al., 2009; Okutucu et al., 2011). Fatigue affects the balance between the sympathetic nervous system (SNS) and the parasympathetic nervous system (PNS) (Castillo-Aguilar et al., 2021; Hebisz et al., 2020; Matuz et al., 2021; Mizuno et al., 2011) by altering the activation of brain regions that contribute to sympathetic-parasympathetic balance (e.g., the dorsal anterior cingulate cortex) (Critchley et al., 2003; Tanaka et al., 2015). Thus, assessing the effect of different fatigue types on ANS activity is essential to improve autonomic function, reduce fatigue-related morbidity and mortality, as well as optimizing recovery strategies. Fatigue is classified as physical fatigue (PF), mental fatigue (MF), and physical-mental fatigue (PMF) (Kume et al., 2017), which are induced by different factors and exercise loads, including prolonged physical activity, demanding cognitive tasks, and insufficient sleep (Dutheil et al., 2012; Maynard et al., 2021; Russell et al., 2020; Schmitt et al., 2013). Furthermore, different types of fatigue may affect ANS activity differently (András et al., 2021; Dobbs et al., 2020; Qin et al., 2021). For instance, compared with the control (absence of fatigue), MF changes PNS parameters by increasing the root mean square of successive differences (RMSSD) between normal heartbeats (Matuz et al., 2021; Qin et al., 2021), while PF has the opposite effect (Dobbs et al., 2020; Hebisz et al., 2020). However, previous studies focused primarily on the effects of PF and MF on the ANS (Castillo-Aguilar et al., 2021; Dobbs et al., 2020; Hebisz et al., 2020; Matuz et al., 2021; Mizuno et al., 2011), and little is known about the effects of PMF on ANS function. Although MF and PF affect the ANS by stimulating or inhibiting PNS activity, respectively, the activation of the PNS and SNS is not linearly correlated. Therefore, the effects of PMF on the regulation of ANS activity need to be further explored. This study evaluated the effects of PMF on ANS function and compared the effects of PF, MF, and PMF on ANS activity. Thus, models of these types of fatigue in healthy younger adults were constructed. ANS activity was assessed by measuring HRV before and after the induction of fatigue. We hypothesized (1) that the induction of PF, MF, and PMF resulted in a corresponding increase in subjectively fatigue levels respectively (e.g., physical fatigue without mental fatigue, mental fatigue without physical fatigue, and physical-mental fatigue) and (2) that different types of fatigue have a different effect on ANS activity. Specifically, PMF and PF leads to high level of sympathetic activity while MF leads to high level of parasympathetic activity.
ParticipantsForty-five physically active individuals from Beijing Sport University voluntarily participated in the study. Participants were included if they engaged in moderate to vigorous physical activity exceeding six hours weekly (Gianola et al., 2017), and their physical activity habits were assessed using the International Physical Activity Questionnaire (IPAQ) (Craig et al., 2003). The average weekly physical activity was 14.47 ± 3.23 h, spanning 4.27 ± 1.46 days, with a mean MET value of 4919.06 ± 3021.50 per week. Average sedentary and sleep durations were 6.13 ± 1.93 hours and 7.45 ± 0.78 h, respectively. All participants gave written informed consent. The exclusion criteria were subjects with injuries that affected the implementation of fatigue-inducing protocols and subjects with burnout. Nine participants were excluded, and 36 (17 females) were included in the study (Table 1). This study was approved by the Human Subjects Review Committee of Beijing Sport University (2021004H), and all procedures were conducted according to the Declaration of Helsinki.
Experimental protocolsParticipants underwent four separate visits, each comprising a specific fatigue-inducing protocol (PF, MF, or PMF) followed by a peak power output assessment. The order of protocols, depicted in Figure 1, was interspersed with a minimum seven-day interval to facilitate complete recovery. This study was conducted from December 2020 through June 2021. To gauge subjective physical and mental fatigue levels, the Rating of Perceived Exertion (RPE) scale and the Visual Analog Scale-Fatigue (VAS-F) were employed. Prior to each session, participants were advised to avoid strenuous physical activities and to refrain from consuming alcohol or caffeine for 24 hours.
Fatigue protocolsPF-inducing protocol: 60-min cycling exercise
The 60-min cycle ergometer exercise included three 20-min blocks with a 10-s interval between blocks, aimed at assessing to the subjective levels of both physical and mental fatigue (Figure 1). The load was 60% of the peak power and remained constant throughout the protocol. Before the experiment, the peak power output of each participant was measured using a cycle ergometer (Ergoselect 100, Ergoline GmbH, Bitz, Germany). The protocol included 1) a warm-up (cycling for 3 min at 30 W and 70 ± 5 rpm) and 2) initial loads of 50 W (males) and 30 W (females) with an increase at a rate of 20 W per 2 min until the test ended or the participant interrupted the test for any reason. The test was terminated when at least two of the following conditions were met: 1) HR ≥ 85% × maximum heart rate (measured using the formula: 208 - [age × 0.7]); (2) RPE ≥ 7; and (3) failure to maintain a speed of 70 ± 5 rpm for more than 10 s. The peak power output and the loads used to induce PF and PMF in both sexes are shown in Table 1.
MF-inducing protocol: 60 min AX-CPTMF was induced using the 60-min AX-Continuous Performance Task (AX-CPT), which was generated and implemented using E-prime 2.0 (Sharpsburg, USA). The task consisted of three 20-min blocks with a 10-s interval between blocks based on four uppercase letters (A, B, X, Y). The task was presented on a 23-inch monitor (Lenovo L12364A, 1920 × 1080 pixels, 60 Hz refresh rate). Before the experimental test, the participants performed a 3.5-min familiarization task (60 trials) consisting of 42 target pairs (70%, AX), six valid cue-invalid probe pairs (10%, AY), six invalid cue-valid probe pairs (10%, BX), and six invalid cue-invalid probe pairs (10%, BY). Each block of the experimental task (total of 340 trials) consisted of 238 AX (70%), 34 AY (10%), 34 BX (10%), and 34 BY (10%). The letters appeared on the screen randomly. One trial of AX-CPT is presented in Figure 2. Participants were instructed to press the target button (left arrow) as soon as the X probe appeared after observing the A cue; while observing any other letter pair, the participants pressed the right arrow button as soon as possible. Responses to probe stimuli were recorded with a time limit of 1500 ms.
PMF-inducing protocol: AX-CPT combined with cycling exercisePMF was induced by simultaneously performing AX-CPT and cycling exercises, the same protocols used to induce MF and PF, respectively.
Outcome measuresHRV and HR
HRV was assessed before and immediately after each task (Figure 1). Participants were asked to sit still for at least 5 min before the test. Then, HRV data were recorded continuously for 3 min in a sitting position using a cardiac chest band (Movesense OP174, Suunto, Vantaa, Finland) connected to an app (Firstbeat Sports Coach, Jyväskylä, Finland) running on a mobile device (iPad, Apple) for monitoring and recording HR throughout the experiment (Chen et al., 2020; Wu et al., 2020). Recordings were conducted in a quiet, temperature-controlled room. Participants were seated comfortably and asked to breathe naturally with their eyes closed.
Learning Burnout ScaleThis scale was developed by Lian et al. (2005) based on the Maslach Burnout Inventory and consists of 20 items that evaluate college student burnout in three domains: dejection (eight items), improper behavior (six items), and reduced personal accomplishment (six items). Items were rated on a Likert scale ranging from one (strongly disagree) to five (strongly agree). Learning burnout and its dimensions were measured as mean scores with a cut-off point of three.
RPE Borg CR-10Subjective PF was assessed using the Borg CR-10 on a scale from 0 to 10 (Borg, 2010) and was defined as an RPE score of at least 5 (Ribeiro et al., 2008). Participants were instructed to complete the RPE scale four times: at baseline and immediately after each block (Figure 1).
Visual Analog Scale-Fatigue (VAS-F)The level of MF was measured using the VAS-F (Veness et al., 2017), which is a 100-mm horizontal line with the marks ‘none’ (not fatigued at all) and ‘maximum’ (extremely fatigued) on the left and right, respectively. Participants were instructed to mark the line based on their subjective MF. The VAS score was the distance between the marked point and the left endpoint, and a VAS-F value of at least 50 mm was defined as the presence of MF (Dernis-Labous et al., 2003).
Data processingAX-CPT performance
Cognitive performance in AX-CPT was recorded automatically by measuring the accuracy and reaction time for four probe pairs (AX, AY, BX, and BY). The average accuracy for these probe pairs every 5 min was measured as cognitive load and mental status during MF- and PMF-inducing tasks.
HRV and HRCardiac autonomic nervous function was evaluated using the time and frequency domains of HRV. HRV measurements were analyzed utilizing the Firstbeat system (https://sports.firstbeat.com/, Jyväskylä, Finland). The time domain consisted of the standard deviation of all RR intervals (SDRR) and the RMSSD in RR intervals. The frequency domain consisted of low-frequency spectral power (LF; 0.05-0.15 Hz), high-frequency spectral power (HF; 0.15-0.4 Hz), and the LF/HF ratio. The normalized units for LF and HF served as indices of autonomic regulation in the participants, with the following formulas:
nLF (nu) = LF (ms2) / total power (ms2) × 100
nHF (nu) = HF (ms2) / total power (ms2) × 100
To assess the effect of fatigue types on cardiac autonomic activity, RMSSD and nHF were utilized as primary outcomes of parasympathetic activity (Laborde et al., 2017), and SDNN served as a secondary outcome (Yılmaz et al., 2018). The LF is indicative of both sympathetic and parasympathetic activity, with a strong propensity towards sympathetic activity (Hayano and Yuda, 2021). Additionally, the nLF/nHF ratio reflects sympathovagal balance (Laborde et al., 2017). Physiological responses to fatigue-inducing protocols were evaluated by measuring HR. The effect of PF- and PMF-inducing protocols on internal and external load, respectively, was assessed by measuring training impulse (TRIMP) every 5 min using the formula:
TRIMP = t × ΔHR × 0.64e (b × ΔHR)
where t is training duration (min), ∆HR is (HR - HR rest) / (HR max - HR rest), e is the base of Napierian logarithms, and b is 1.67 for women and 1.92 for men (Sanders et al., 2017). HR max and HR were considered the maximal and average HR and were measured every 5 min.
Statistical analysisA power analysis was conducted using G*Power 3.1 (parameters: one-way repeated measure ANOVA, within factors, f = 0.25, P = 0.05, power = 0.90, number of groups: 3, number of measurement points: 4) yielding a sample size of 30 participants. For the present study, 36 participants were recruited and included in the final analyses. Data were expressed as means ± standard deviations (M ± SD). Statistical analyses were conducted using SPSS version 25.0 (SPSS Inc., Chicago, USA). The normality of the distribution of continuous variables was assessed using the Shapiro-Wilk test. The effects of PMF on ANS activity were examined using a paired-samples t-test. The dependent variables were the outcomes of HRV (RMSSD, SDNN, nLF/nHF, nLF, and nHF). This research undertook a stratified analysis to investigate the differential impacts of fatigue on autonomic nervous system activity across genders. The effects of PMF and PF on ANS activity were compared using two-way analysis of variance (ANOVA). The dependent variable encompassed the percentage changes in HRV parameters (∆RMSSD, ∆SDNN, ∆nLF, ∆nHF, and ∆nLF/nHF), with fatigue type and gender serving as independent variables. RPE was included as a covariate because fatigue might affect ANS activity. Similarly, VAS-F (subjective level of mental fatigue) was included as a covariate to analyze differences in the effects of MF- and PMF-inducing protocols. The effectiveness of the fatigue models (PF, MF, and PMF) was evaluated by one-way repeated-measures ANOVA. The dependent variables of the models were RPE and VAS-F. The effects of cycling exercises alone and AX-CPT combined with cycling on heart rate and TRIMP, and the effects of AX-CPT alone and AX-CPT combined with cycling on cognitive task performance (i.e., accuracy) were assessed using a two-way repeated-measures ANOVA. Statistical significance was defined as P < 0.05. The effect size was calculated using Cohen’s d index and classified as small (d = 0.2), medium (d = 0.5), or large (d = 0.8) (Jacob, 1992).
The participants completed all study visits. There were no significant differences in subjective fatigue levels (RPE and VAS-F) and HRV parameters (SDNN, RMSSD, nLF/nHF, nLF, and nHF) between the three protocols at baseline. PF- and PMF-inducing protocols significantly increased RPE scores (F = 169.463, P < 0.001, η2 = 0.939; F = 222.803, P < 0.001, η2 = 0.953) and led to extreme physical exertion (Figure 3A). MF- and PMF-inducing protocols significantly increased VAS-F scores (F = 56.171, P < 0.001, η2 = 0.836; F = 140.819, P < 0.001, η2 = 0.928) and caused severe mental fatigue (Figure 3B). These results suggested that the PF, MF, and PMF models were effective. The peak power and experimental load (60% of the peak power) were 216.32 ± 37.15 W and 129.74 ± 22.30 W for males and 154.71 ± 30.44 W and 92.82 ± 18.26 W for females (Table 1).
Effects of PMF on ANS activityThe paired-samples t-test showed that PMF significantly decreased the RMSSD, SDNN, and nHF, while increasing nLF and the nLF/nHF ratio compared to baseline (Table 2). However, there were no significant differences observed in the nLF, nHF, and the nLF/nHF ratio for male participants, nor in the SDNN for female subjects.
Effects of PF and PMF on ANS activity and kinematic measuresAn investigation into the influence of fatigue type (PMF vs. PF) and gender (male vs. female) on HRV parameters was performed using a two-way ANOVA. M and SD for ∆RMSSD, ∆SDNN, ∆nLF, ∆nHF, and ∆nLF/nHF, are presented in supplementary Table 3. A significant main effect of gender was found on ∆nHF (F = 4.288, P < 0.05, η2 = 0.079), but not others (see supplementary Table 4). For kinematic parameters, two-way repeated-measures ANOVA indicated no significant interactions between fatigue type and exercise block for average HR (F = 1.744, P = 0.060; see Figure 4A) and TRIMP (F = 0.929, P = 0.511; Figure 4B). Heart rate (F = 43.245, P < 0.001, η2 = 0.888) and TRIMP (F = 12.048, P < 0.001, η2 = 0.692) increased gradually during PF- and PMF-inducing tasks, without significant difference between these tasks (F = 1.600, P = 0.210; F = 1.814, P = 0.182).
Effects of MF and PMF on ANS activity and cognitive task performanceIn examining the effects of fatigue type (PMF and MF) and gender (male and female) on HRV paraments, a two-way ANOVA was conducted. There was a significant main effect for fatigue type on ∆RMSSD (F = 20.809, P < 0.001, η2 = 0.431), ∆SDNN (F = 13.361, P < 0.001, η2 = 0.327), but not ∆nLF (F = 3.107, P = 0.083), ∆nHF (F = 1.840, P = 0.180) and ∆nLF/nHF (F = 2.493, P = 0.119). The main effect for gender as well as the interaction effect between fatigue type and gender were not significant. Post-hoc analyses indicated that ∆RMSSD and ∆SDNN were significantly lower in the PMF model than in the MF model (Figure 5). Two-way repeated-measures ANOVA indicated no significant interactions between fatigue types and exercise block for cognitive task performance (F = 1.064, P = 0.388) (Figure 4C). Cognitive performance (i.e., accuracy in the AX-CPT) decreased gradually during MF- and PMF-inducing protocols (F = 5.971, P < 0.001, η2 = 0.531) and was lower in the latter (F = 7.643, P < 0.01, η2 = 0.101).
This study evaluated the effects of PF, MF, and PMF on HRV, marking the first to assess these specific fatigue types on autonomic nervous system (ANS) activity. Our tasks induced distinct fatigue states, confirmed by subjective, physiological, and cognitive measures. Regarding ANS activity, PMF closely resembles PF by enhancing cardiac sympathetic activity while diminishing parasympathetic activity. PMF decreased RMSSSD, SDNN more strongly than MF, indicating that different fatigue types influenced cardiac ANS activity differently.
Effectiveness of protocolsPerforming a cycling exercise at 60% of the peak power output for 60 min strongly increased RPE and marginally increased VAS-F, suggesting the successful induction of PF without MF (VAS-F < 50), in line with a previous study (Davidow et al., 2020), wherein prolonged high-intensity intermittent running induced PF without MF in rugby union players. In addition, we observed a linear increase in HR and TRIMP, suggesting that an increase in physical workload was consistent with the time-on-protocol. Performing 60-min AX-CPT induced MF without PF (RPE < 5), evidenced by a strong increase in subjective scores (VAS-F) and a significant decrease in cognitive task performance (accuracy). Previous research has shown that 60-min AX-CPT can effectively induce mental fatigue in healthy college students (VAS > 60) without physical fatigue (VAS < 40) (Loch et al., 2020). Moreover, participants’ responses became gradually faster as accuracy decreased; this effect was also observed during the execution of the PMF-inducing protocol, consistent with previous studies (Delliaux et al., 2019; Thomson et al., 2009). Decreased accuracy may be due to a loss of motivation and low levels of self-efficacy due to MF (Loch et al., 2020) and an increase in wrong responses due to short reaction times (Thomson et al., 2009). AX-CPT combined with cycling exercise strongly induced PMF, demonstrated by increased subjective PF and MF, increased workload consistent with the time-on-task, and decreased cognitive task performance. The effectiveness of this model in inducing PM and MF has been validated previously (Barzegarpoor et al., 2020; Haji Agha Bozorgi et al., 2021). For instance, Barzegarpoor et al. (Barzegarpoor et al., 2020) found that PF and MF levels increased significantly (RPE > 16, NASA-TLX > 60) in male recreational cyclists after 45-min AX-CPT combined with cycling exercises.
Effects of fatigue on cardiac autonomic functionThe effect of different types of fatigue on cardiac autonomic activity was assessed by measuring HRV. The results showed that PF remarkably decreased RMSSD, SDNN, nHF, and nLF, suggesting decreased parasympathetic activity. Meanwhile, the increase in the nLF/nHF ratio, an indicator of sympathovagal balance, suggests higher sympathetic activity and lower parasympathetic activity (Michael et al., 2017), in line with a previous study (Dobbs et al., 2020) showing a significant decrease in the time and frequency domains at 30 min after high-volume back squats. In addition, cycling exercise increases HR linearly because of higher sympathetic activity to maintain physical performance (Borresen and Lambert, 2008). Long-term physical exercise-induced PF increases cardiac sympathetic activity and parasympathetic withdrawal. Regarding MF, the results showed that SDNN, nLF, and nLF/nHF were increased 5 min after 60-min AX-CPT, suggesting that increased cardiac parasympathetic activity is a feature of MF. Moreover, the slight decrease in HR in each exercise block during the MF-inducing task indicates an increase in cardiac parasympathetic modulation (Hallman et al., 2019), in line with previous studies (Matuz et al., 2021; Qin et al., 2021). The previous study reported that healthy college students had a significant increase in parasympathetic activity (lnRMSSD, pNN50, lnHF, lnLF, and SD2) after completing a 1.5-h dual 2-back task (Matuz et al., 2021). However, Delliaux et al. (Delliaux et al., 2019) found that most HRV paraments were remarkedly decreased (a significant decrease in time domain measures and a slight increase in the LF/HF ratio) during a 1-h task-switching task, indicating either decreased parasympathetic activity or increased sympathetic activity. This inconsistency might be because HRV was measured under fatigue conditions at different time points (i.e., during or after cognitive task completion). Consistent with our hypothesis, our results showed that fatigue induced by physical and cognitive load had a markedly different effect on cardiac autonomic function. More specifically, PF increased sympathetic activity and decreased parasympathetic activity, while MF exhibited the opposite effect. These observations were corroborated by variations in HR following and after different types of stimulation (i.e., physical and mental workloads). In comparison to the baseline, HR exhibited a significant increase during physical load and PF scenarios, but a minor decrease during mental load and MF scenarios, suggesting that different types of load-induced fatigue elicit unique patterns of ANS activation. There changes in HR and ANS might be related to the activation of the central autonomic network, which controls sympathetic-parasympathetic balance (Tanaka et al., 2015), such as the dorsal anterior cingulate cortex (ACC) controlling sympathetic output (Critchley et al., 2003). Previous studies have shown that higher parasympathetic activity under MF is closely associated with decreased activity in the medial prefrontal cortex and ACC (Caseras et al., 2008; Lorist et al., 2005). In contrast, Liu et al. (Liu et al., 2007) found that PF increased activity in ipsilateral sensorimotor, prefrontal, orbitofrontal, and anterior cingulate regions. Another explanation for this finding is that moderate-high-intensity physical exercise increases sympathetic activity as a result of arterial baroreflex control and the secretion of epinephrine and norepinephrine, increasing HR to meet the demands of working muscles (maintain physical performance), increase peripheral blood flow (for heat dissipation), and maintain the function of other organs (Hebisz RG et al., 2020). However, acetylcholine release is increased during cognitive task performance (Passetti et al., 2000); this neurotransmitter binds to muscarinic receptors and augments parasympathetic activity, leading to a slight decrease in HR (Shaffer et al., 2014). Regarding PMF, the 60-min AX-CPT combined with cycling exercises significantly decreased RMSSD, nLF, and nHF and significantly increased the nLF/nHF ratio, suggesting that PMF increased cardiac sympathetic activity and decreased parasympathetic activity. Nonetheless, changes in HRV paraments were similar between PF and PMF, which was unexpected but in line with a previous study (Garde et al., 2002). This result may be related to the regulation of sympathetic and parasympathetic nerves. Parasympathetic nerves on the sinoatrial node, atrial myocardium, and atrioventricular node are activated at rest, while sympathetic nerves are activated during physical activity (Borresen and Lambert, 2008). Sympathetic activation triggers the release of norepinephrine and epinephrine (Shaffer et al., 2014), increasing HR and cardiac contractility to maintain exercise performance. Therefore, sympathetic nerves may play a dominant role during moderate-high intensity physical activity, regardless of whether cognitive tasks are performed. Sympathetic drive persists for some time despite parasympathetic activation to facilitate recovery from medium-high intensity exercises in which sympathetic activity predominates (Borresen and Lambert, 2008). The effects of MF and PMF on ∆RMSSD, ∆SDNN, ∆nLF, ∆nHF, and ∆nLF/nHF were distinct, suggesting that these protocols influenced cardiac ANS activity differently. PMF increased sympathetic activity and decreased parasympathetic activity, while MF had the opposite effect. One possible explanation for this effect is that sympathetic activity predominates during the dual task to maintain physical performance. In addition, participants tend to maintain exercise performance at the expense of cognitive performance (accuracy), and the latter was significantly lower during the dual task than during AX-CPT.
Effects of gender on cardiac autonomic functionThe study yielded a notable observation that autonomic nervous activity differed among participants based on gender, which was in line with the previous studies (Voss et al., 2015; Woo and Kim, 2015). It was observed that males exhibited a less significant reduction in nHF power in response to fatigue when compared to females. While the precise reasons for this discrepancy are challenging to ascertain, it may be attributed to inherent physiological differences between genders, notably in hormonal responses (Voss et al., 2015). Such differences could potentially influence cardiac autonomic regulation and stress adaptation.
Strengths and limitationsThis study analyzed the effects of PF and MF on cardiac autonomic activity under rigorously controlled conditions, including protocol duration and respiratory control during HRV measurements. These findings elucidated how different types of fatigue affected cardiac autonomic activity and showed that HRV was useful for assessing fatigue. For workers with physically and mentally demanding jobs, investigating the association between ANS and PMF is critical to maintaining job performance and reducing fatigue-related morbidity from work-related musculoskeletal disorders (Mehta and Agnew, 2011). This study has some limitations. First, since the study implemented three distinct fatigue-inducing protocols, measuring HRV during task execution under identical control conditions (i.e., sitting still for at least 5 min) was unfeasible. Second, the effects of long-term fatigue on HRV were not assessed. Third, the effects of fatigue on ANS function may be affected by fatigue-inducing tasks not encountered in real-life situations. Therefore, the association of HRV with ANS function and real-life long-term fatigue needs to be further researched.
Our findings suggest that PF and PMF increased sympathetic activity and decreased parasympathetic function. Further, MF increased parasympathetic activity more strongly than PF and PMF.
ACKNOWLEDGEMENTS |
The authors thank all the study participants. This research was supported by the National Key R&D Project of the “Science and Technology Winter Olympics” Key Special Project (Grant No. 2019YFF0301602-3) and the National Team Technology Assisted Youth Program (Grant No. 22QN007). The authors have no conflicts of interest. The present study complies with the current laws of the country in which it was performed. The datasets generated and analyzed during the current study are not publicly available but are available from the corresponding author, who was an organizer of the study. |
|
AUTHOR BIOGRAPHY |
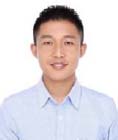 |
Yan Chen |
Employment: Sports Department, Beihang University, Beijing, China |
Degree: PhD |
Research interests: Theory and practice of sports training, strength and conditioning training, and physical health interventions |
E-mail: 11339@buaa.edu.cn |
|
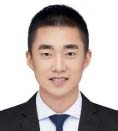 |
Meng Liu |
Employment: China Athletics College, Beijing Sport University, Beijing, China |
Degree: PhD candidate |
Research interests: Theory and practice of sports training |
E-mail: 15600693512@163.com |
|
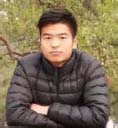 |
Jun Zhou |
Employment: China Athletics College, Beijing Sport University, Beijing, China |
Degree: MA |
Research interests: Strength and conditioning training |
E-mail: Zhouj_bsu@bsu.edu.cn |
|
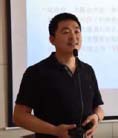 |
Dapeng Bao |
Employment: China Institute of Sport and Health Science, Beijing Sport University, Beijing, China |
Degree: PhD |
Research interests: Physical fitness test and assessment |
E-mail: baodp@bsu.edu.cn |
|
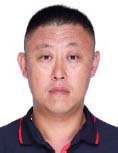 |
Bin Li |
Employment: Cycling and Fencing Administrative Center, General Administration of Sport of China, Beijing, China |
Degree: BS |
Research interests: Sports injury rehabilitation, sports medicine |
E-mail: lbjn081012@163.com |
|
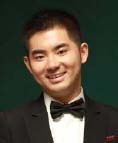 |
Junhong Zhou |
Employment: Hebrew SeniorLife Hinda and Arthur Marcus Institute for Aging Research, Harvard Medical School, Boston, MA, United States |
Degree: PhD |
Research interests: Physiotherapy, Rehabilitation, and Medicine Geriatrics |
E-mail: zhoujunhong54@gmail.com |
|
|
|
REFERENCES |
 András M., Linden D., Kisander Z., István H., Karádi K., Rpád C. (2021) Enhanced cardiac vagal tone in mental fatigue: Analysis of heart rate variability in Time-on-Task, recovery, and reactivity. Plos One 16, e0238670. Crossref
|
 Barzegarpoor H., Amoozi H., Rajabi H., Button D., Fayazmilani R. (2020) The Effects of Performing Mental Exertion during Cycling Exercise on Fatigue Indices. International Joural of Sports Medicine 41, 846-857. Crossref
|
 Borg G. (2010) The Borg CR Scales® Folder. Methods for measuring intensity of experience. J Borg Perception, Hasselby, Sweden.
|
 Borresen J., Lambert M. I. (2008) Autonomic control of heart rate during and after exercise. Sports Medicine 38, 633-646. Crossref
|
 Caseras X., Mataix-Cols D., Rimes K. A., Giampietro V., Brammer M., Zelaya F., Chalder T., Godfrey E. (2008) The neural correlates of fatigue: an exploratory imaginal fatigue provocation study in chronic fatigue syndrome. Psychological Medicine 38, 941-951. Crossref
|
 Castillo-Aguilar M., Valdés-Badilla P., Herrera-Valenzuela T., Guzmán-Muñoz E., Delgado-Floody P., Andrade D. C., Moraes M.M., Arantes R.M., Núñez-Espinosa C. (2021) Cardiac Autonomic Modulation in Response to Muscle Fatigue and Sex Differences During Consecutive Competition Periods in Young Swimmers: A Longitudinal Study. Frontiers in Physiology 12, 769085. Crossref
|
 Chen Y.-S., Lu W.-A., Pagaduan J. C., Kuo C.-D. (2020) A novel smartphone app for the measurement of ultra–short-term and short-term heart rate variability: validity and reliability study. JMIR mHealth and uHealth 8, e18761. Crossref
|
 Craig C.L., Marshall A.L., Sjöström M., Bauman A.E., Booth M.L., Ainsworth B.E., Pratt M., Ekelund U.L.F., Yngve A., Sallis J.F., Oja P. (2003) International physical activity questionnaire: 12-country reliability and validity. Medicine Science in Sports Exercise 35, 1381-1395. Crossref
|
 Critchley H.D., Mathias C.J., Josephs O., O’Doherty J., Zanini S., Dewar B.K., Cipolotti L., Shallice T., Dolan R.J. (2003) Human cingulate cortex and autonomic control: converging neuroimaging and clinical evidence. Brain 126, 2139-2152. Crossref
|
 Davidow D., Redman M., Lambert M., Burger N., Smith M., Jones B., Hendricks S. (2020) The effect of physical fatigue on tackling technique in Rugby Union. Journal of science and medicine in sport 23, 1105-1110. Crossref
|
 Delliaux S., Delaforge A., Deharo J. C., Chaumet G. (2019) Mental Workload Alters Heart Rate Variability, Lowering Non-linear Dynamics. Frontiers in Physiology 10, 565-578. Crossref
|
 Dernis-Labous E., Messow M., Dougados M. (2003) Assessment of fatigue in the management of patients with ankylosing spondylitis. Rheumatology 42, 1523-1528. Crossref
|
 Dobbs W. C., Fedewa M. V., MacDonald H. V., Tolusso D. V., Esco M. R. (2020) Profiles of Heart Rate Variability and Bar Velocity after Resistance Exercise. Medicine and Science in Sports and Exercise 52, 1825-1833. Crossref
|
 Dutheil F., Boudet G., Perrier C., Lac G., Ouchchane L., Chamoux A., Duclos M., Schmidt J. (2012) JOBSTRESS study: comparison of heart rate variability in emergency physicians working a 24-hour shift or a 14-hour night shift - a randomized trial. International journal of cardiology 158, 322-325. Crossref
|
 Escorihuela R. M., Capdevila L., Castro J. R., Zaragozà M. C., Maurel S., Alegre J., Castro-Marrero J. (2020) Reduced heart rate variability predicts fatigue severity in individuals with chronic fatigue syndrome/myalgic encephalomyelitis. Journal of Translational Medicine 18, 1-12. Crossref
|
 Garde A. H., Laursen B., Jorgensen A. H., Jensen B. R. (2002) Effects of mental and physical demands on heart rate variability during computer work. European journal of applied physiology 87, 456-461. Crossref
|
 Gianola S., Castellini G., Stucovitz E., Nardo A., Banfi G. (2017) Single leg squat performance in physically and non-physically active individuals: a cross-sectional study. BMC Musculoskeletal Disorders 18, 1-10. Crossref
|
 Haji Agha Bozorgi H., Rajabi H., Barzegarpoor H., Fayazmilani R. (2021) The effect of simultaneous submaximal physical exercise and mental exertion on fatigue indices in active men. Journal of Sport Exercise Physiology 14, 1-10. Crossref
|
 Hallman D. M., Krause N., Jensen M. T., Gupta N., Birk Jørgensen M., Holtermann A. (2019) Objectively measured sitting and standing in workers: cross-sectional relationship with autonomic cardiac modulation. International Journal of Environmental Research and Public Health 16, 650. Crossref
|
 Hayano J., Yuda E. (2021) Assessment of autonomic function by long-term heart rate variability: Beyond the classical framework of LF and HF measurements. Journal of Physiological Anthropology 40, 21. Crossref
|
 Hebisz R.G., Hebisz P., Zatoń M.W. (2020) Heart Rate Variability After Sprint Interval Training in Cyclists and Implications for Assessing Physical Fatigue. Journal of Strength and Conditioning Research 36, 558-564. Crossref
|
 Hegde V., Borade N., Naveen S., Devarakonda B. V. (2018) Heart rate variability in trained athletes and sedentary individuals-A Comparative study. Indian Journal of Clinical Anatomy Physiology 5, 237-240. Crossref
|
 Jacob C. (1992) A power primer. Psychological Bulletin 112, 155-159. Crossref
|
 Kume S., Nishimura Y., Mizuno K., Sakimoto N., Hori H., Tamura Y., Yamato M., Mitsuhashi R., Akiba K., Koizumi J.I., Watanabe Y. (2017) Music improves subjective feelings leading to cardiac autonomic nervous modulation: a pilot study. Frontiers in Neuroscience 11, 108. Crossref
|
 Laborde S., Mosley E., Thayer J. F. (2017) Heart Rate Variability and Cardiac Vagal Tone in Psychophysiological Research - Recommendations for Experiment Planning, Data Analysis, and Data Reporting. Frontiers in psychology 8, 213. Crossref
|
 Lian R., Yang L., Wu L. (2005) The relationship between college students’ professional commitment and learning burnout and the development of the scale. Acta Psychologica Sinica 37, 632-636.
|
 Liu J. Z., Lewandowski B., Karakasis C., Yao B., Siemionow V., Sahgal V., Yue G. H. (2007) Shifting of activation center in the brain during muscle fatigue: an explanation of minimal central fatigue?. Neuroimage 35, 299-307. Crossref
|
 Loch F., Hof Zum Berge A., Ferrauti A., Meyer T., Pfeiffer M., Kellmann M. (2020) Acute Effects of Mental Recovery Strategies After a Mentally Fatiguing Task. Frontiers in psychology 11, 558856. Crossref
|
 Lorist M. M., Boksem M. A., Ridderinkhof K. R. (2005) Impaired cognitive control and reduced cingulate activity during mental fatigue. Cognitive Brain Research 24, 199-205. Crossref
|
 Marcora S. M., Staiano W., Manning V. (2009) Mental fatigue impairs physical performance in humans. Journal of applied physiology 106, 857-864. Crossref
|
 Matuz A., van der Linden D., Darnai G., Csathó Á. (2022) Generalisable machine learning models trained on heart rate variability data to predict mental fatigue. Scientific Reports 12, 20023. Crossref
|
 Matuz A., van der Linden D., Kisander Z., Hernadi I., Kazmer K., Csatho A. (2021) Enhanced cardiac vagal tone in mental fatigue: Analysis of heart rate variability in Time-on-Task, recovery, and reactivity. Plos One 16, e0238670. Crossref
|
 Maynard S., Filtness A., Miller K., Pilkington-Cheney F. (2021) Bus driver fatigue: A qualitative study of drivers in London. Applied Ergonomics 92, 103309-. Crossref
|
 Mehta R. K., Agnew M. J. (2011) Effects of concurrent physical and mental demands for a short duration static task. International Journal of Industrial Ergonomics 41, 488-493. Crossref
|
 Michael S., Graham K. S., Davis G. M. (2017) Cardiac autonomic responses during exercise and post-exercise recovery using heart rate variability and systolic time intervals -a review. Frontiers in physiology 8, 301. Crossref
|
 Mizuno K., Tanaka M., Yamaguti K., Kajimoto O., Kuratsune H., Watanabe Y. (2011) Mental fatigue caused by prolonged cognitive load associated with sympathetic hyperactivity. Behavioral and Brain Functions 7, 1-7. Crossref
|
 Ni Z., Sun F., Li Y. (2022) Heart Rate Variability-Based Subjective Physical Fatigue Assessment. Sensors 22, 3199. Crossref
|
 Okutucu S., Karakulak U. N., Aytemir K., Oto A. (2011) Heart rate recovery: a practical clinical indicator of abnormal cardiac autonomic function. Expert Review of Cardiovascular Therapy 9, 1417-1430. Crossref
|
 Passetti F., Dalley J., O'connell M., Everitt B., Robbins T. (2000) Increased acetylcholine release in the rat medial prefrontal cortex during performance of a visual attentional task. European Journal of Neuroscience 12, 3051-3058. Crossref
|
 Qin H., Zhou X., Ou X., Liu Y., Xue C. (2021) Detection of mental fatigue state using heart rate variability and eye metrics during simulated flight. Human Factors Ergonomics in Manufacturing 31, 637-651. Crossref
|
 Ribeiro F., Santos F., Gonçalves P., Oliveira J. (2008) Effects of volleyball match-induced fatigue on knee joint position sense. European Journal of Sport Science 8, 397-402. Crossref
|
 Russell S., Jenkins D., Halson S., Kelly V. (2020) Changes in subjective mental and physical fatigue during netball games in elite development athletes. Journal of Science Medicine in Sport 23, 615-620. Crossref
|
 Sanders D., Abt G., Hesselink M. K., Myers T., Akubat I. (2017) Methods of monitoring training load and their relationships to changes in fitness and performance in competitive road cyclists. International Journal of Sports Physiology and Performance 12, 668-675. Crossref
|
 Schmitt L., Regnard J., Desmarets M., Mauny F., Mourot L., Fouillot J.P., Coulmy N., Millet G. (2013) Fatigue shifts and scatters heart rate variability in elite endurance athletes. Plos One 8, e71588. Crossref
|
 Seiler S., Haugen O., Kuffel E. (2007) Autonomic recovery after exercise in trained athletes: intensity and duration effects. Medicine Science in Sports Exercise 39, 1366. Crossref
|
 Shaffer F., McCraty R., Zerr C. L. (2014) A healthy heart is not a metronome: an integrative review of the heart's anatomy and heart rate variability. Frontiers in psychology 5, 1040. Crossref
|
 Tanaka M., Mizuno K., Yamaguti K., Kuratsune H., Fujii A., Baba H., Matsuda K., Nishimae A., Takesaka T., Watanabe Y. (2011) Autonomic nervous alterations associated with daily level of fatigue. Behavioral and Brain Functions 7, 1-6. Crossref
|
 Tanaka Tajima S., Mizuno K., Ishii A., Konishi Y., Miike T., Watanabe Y. (2015) Frontier studies on fatigue, autonomic nerve dysfunction, and sleep-rhythm disorder. The Journal of Physiological Sciences 65, 483-498. Crossref
|
 Thomson, K., Watt, A. P. and Liukkonen, J. (2009) Differences in ball
sports athletes speed discrimination skills before and after
exercise induced fatigue. Journal of Sports Science and Medicine
8(2), 259-264. Pubmed
|
 Veness D., Patterson S. D., Jeffries O., Waldron M. (2017) The effects of mental fatigue on cricket-relevant performance among elite players. Journal of Sports Sciences 35, 2461-2467. Crossref
|
 Voss A., Schroeder R., Heitmann A., Peters A., Perz S. (2015) Short-term heart rate variability - influence of gender and age in healthy subjects. Plos One 10, e0118308. Crossref
|
 Woo J.-M., Kim T.-S. (2015) Gender plays significant role in short-term heart rate variability. Applied Psychophysiology Biofeedback 40, 297-303. Crossref
|
 Wu L., Shi P., Yu H., Liu Y. (2020) An optimization study of the ultra-short period for HRV analysis at rest and post-exercise. Journal of Electrocardiology 63, 57-63. Crossref
|
 Yoshiuchi K., Quigley K. S., Ohashi K., Yamamoto Y., Natelson B. H. (2004) Use of time-frequency analysis to investigate temporal patterns of cardiac autonomic response during head-up tilt in chronic fatigue syndrome. Autonomic Neuroscience 113, 55-62. Crossref
|
 Yılmaz M., Kayançiçek H., Çekici Y. (2018) Heart rate variability: Highlights from hidden signals. Journal Of Interventional Cardiology 4, 1-8. Crossref
|
|
|
|
|
|
|