|
|
|
ABSTRACT |
This study aimed to narrow down the possible mechanisms of Post-Activation Performance Enhancement (PAPE), especially if they are exclusively found in the muscle. It was therefore investigated whether (1) the PAPE effect is influenced by neural factors and (2) if Post-Activation-Potentiation (PAP) influences PAPE. Thirteen strength-trained participants (26.5 ± 3.2 years) took part in at least one of three interventions (PAP, PAPE-Electrical (PAPEE), and PAPE-Voluntary (PAPEV)). Conditioning contractions (CC) and testing involved isometric knee extensions performed on an isokinetic device at an 80° knee flexion angle. The CC was either performed voluntarily (PAP, PAPEV) or was evoked through electrical stimulation (PAPEE). Testing was performed at baseline and after two seconds, four minutes, eight minutes, and twelve minutes of the CC. Maximum voluntary isometric contractions (MVIC) for the PAPE trials and supramaximal twitches for the PAP trial were used for testing. Parameters of interest were peak torque and rate of torque development (RTD), and electromyography (EMG) amplitude of the quadriceps (only PAPE). Repeated measures ANOVA and simple contrast comparisons were used for statistical analysis. Peak torque (p < 0.001, η2p = 0.715) and RTD (p = 0. 005, η2p = 0.570) increased significantly during the PAP protocol immediately two seconds after the CC and decreased to near baseline values for the following time points (p > 0.05). Peak torque, RTD, and peak EMG showed no significant differences during PAPEE and PAPEV trials (p > 0.05). Due to the lack of a visible PAPE effect, the question of whether neural mechanisms influence PAPE cannot be answered. Due to the time course of the PAP analysis, it is questionable if these mechanisms play a role in PAPE. The assumption that the PAP mechanism influences PAPE cannot be confirmed for the same reason. |
Key words:
electric stimulation, torque, isometric contraction, quadriceps muscle, phosphorylation, human
|
Key
Points
- A post-activation-potentiation-effect was clearly visible in this study immediately after the conditioning contraction.
- Post-activation-performance-enhancement could not be seen with the employed protocol.
- The post-activation-potentiation time course seems to be outside of the proposed post-activation-performance-enhancement time window.
|
In sports, athletes look for ways to improve their performance, even if it is only for a short time window. One of the techniques said to increase sporting performance is Post-Activation-Potentiation (PAP). In recent years, PAP has been used increasingly in the context of enhancing voluntary force or the rate of force development (RFD) (Krzysztofik et al., 2020; Lorenz, 2011; Seitz and Haff, 2016; Tillin and Bishop, 2009). In the studies researching the voluntary performance increase, the protocol consists of a voluntary pre- and post-test (different forms of jumping, sprints, or throws) with a voluntary conditioning contraction (CC) usually ranging from 60% to 100% of the maximum voluntary force (Dobbs et al., 2019; Gouvêa et al., 2013; Seitz and Haff, 2016; Wilson et al., 2013). An increase in performance is predominantly noted five to ten minutes after the CC (Seitz and Haff, 2016; Wilson et al., 2013). Conversely, a rest interval of less than three minutes appears to be counterproductive to this effect (Dobbs et al., 2019). Originally, PAP was used to describe an increase in a muscle’s peak twitch torque and its RFD conditioned by a voluntary activation of the same muscle (Blazevich and Babault, 2019; Vandervoort et al., 1983). The mechanism proposed for this increase is the phosphorylation of the regulatory myosin light chain, which allows the myosin head to move closer to the actin-binding sites and, by doing so, increases the possibility of a cross-bridge formation (Blazevich and Babault, 2019; Lorenz, 2011). It was also proposed that this effect is most effective at submaximal contractions (Blazevich and Babault, 2019; Brown and Euler, 1938) and that it is significant for only a few minutes (<3min) with a half-life of about 28s (Blazevich and Babault, 2019; Vandervoort et al., 1983). These discrepancies between the originally described PAP effect and the attempt to demonstrate PAP through voluntary muscle activation led to the idea of introducing the term Post-Activation-Performance-Enhancement (PAPE) to describe the latter phenomenon (Blazevich and Babault, 2019; Cuenca-Fernández et al., 2017; Prieske et al., 2020). The fact that PAPE is showing a voluntary performance increase and is mostly seen in a different time window raises the question of whether the mechanism underpinning PAP is also responsible for PAPE, namely the phosphorylation of the regulatory myosin light chain. Because of the previously mentioned short half-life of the phosphorylation, this seems rather implausible, but the PAP mechanism still could influence PAPE in its early stages (<6 minutes). Several theories have been proposed about possible PAPE mechanisms, such as increased muscle temperature, muscle and muscle fiber water content, and motivational aspects (Elmubarak and Ranatunga, 1984, Blazevich and Babault, 2019). For instance, increased muscle temperature could lead to an increased RFD. This is because the myosin ATPase reaction appears to be temperature sensitive and, therefore, can influence the cross-bridge cycling rates (Stein et al., 1982). According to Blazevich and Babault (2019), an increase in voluntary performance by 1-5% can be fully explained, and an increase of 5-10% can be at least partly explained by an increase in muscle temperature. Also, the time course where PAPE should show its’ peak effect (around 5-10 minutes after the CC) seems to correspond to the time course of the peak increase in temperature after an intense exercise bout (González-Alonso et al., 2000). An increase in muscle and muscle fiber water content could not only increase RFD but also the muscle fiber force (Edman and Hwang, 1977; Sugi et al., 2015). This is achieved by a decreased ionic strength within the muscle fibers and the subsequent increase in force generated per cross-bridge (Sugi et al., 2015; Wang et al., 2015). Missing information in the current literature is if the voluntary CC that is usually done in PAPE studies also influences neural factors(e.g., increased central neural drive, enhanced excitation of the alpha motor neuron) of the participants and, therefore, if these changes might also explain parts of the effects seen in PAPE studies. Previous studies investigating the PAPE effect often report no or inconclusive changes in the electromyographic (EMG) activity, although an increase in performance was visible (Seitz et al., 2015; Mina et al., 2016). At the same time, a study from Nuzzo et al. (2016) showed that an acute bout of strength training can enhance the effectiveness of corticospinal-motoneuronal synapses or increase motoneuron excitability. Therefore, whether this also happens during the CC of PAPE has to be clarified. To test if neural, besides peripheral factors, play a role in the PAPE effect, a voluntary CC (PAPEV) and an electrical stimulated CC (PAPEE) were performed in this study, matched for intensity and duration. Hence, if neuronal factors play a role in the PAPE effect, the effect after the voluntary CC should be more considerable than after the stimulated CC, relative to the baseline testing. To summarize, this study aimed to answer two questions. First, if the PAPE effect depends on neural factors, and second, if the PAP mechanism contributes to possible PAPE effects during a maximal isometric knee extension. Based on the previously mentioned information, we hypothesize that neural factors play a role in the PAPE effect. Furthermore, we hypothesize that the PAP mechanism does not play a significant role for the PAPE effect during maximal isometric knee extensions.
ParticipantsA priori power analysis using G*Power determined that a total sample size of 6 was needed for our study. For the analysis an effect size f of 0.74 (cohen’s d: 1.48) (Lowery et al., 2012), an alpha level of 0.05 and a power of 0.8 was chosen. Fourteen male sport students were recruited (26.9 ± 3.26 years, 182.6cm ± 4,94cm height, 85.3kg ± 9.6kg weight). Of those 14 participants, one declined to participate after the familiarization session. Ten participants completed all three interventions. Two participants completed one intervention, and one subject completed two interventions. It ended up with 11 participants for each condition (Figure 1). Requirements for participation were regular strength training (at least 2x per week), experience in strength training for at least three years, a minimum age of 25 years, and a maximum age of 35 years. In addition, no orthopedic or neuromuscular injuries of the lower extremities and no cardiovascular diseases were allowed to be present. Before study participation, all participants were extensively informed about the possible risks and consequences of the study. Furthermore, before familiarization, written informed consent and privacy statements were obtained from the participants. The study was approved by the local Ethics Committee (number) of the Technical University of Munich and conducted according to the declaration of Helsinki.
Experimental approach to the problemA within-subject repeated measures design was chosen to determine whether neural factors can be attributed to the PAPE effect, whether there are changes in the muscle activity due to the PAPE effect, and to which extent the PAP mechanism influences PAPE. The quadriceps muscle of the dominant leg of the subject was selected as this muscle group showed PAP (Johnson et al., 2019; Mitchell and Sale, 2011; Requena et al., 2011) and PAPE (Beato et al., 2021; Chaouachi et al., 2011) effects in previous studies. Another reason the quadriceps was chosen for the present study was that the study population consisted of resistance-trained participants who already used knee extensions as part of their regular training program. Isometric knee extensions were used as testing and conditioning interventions (Arabatzi et al., 2014; Esformes et al., 2011; Rixon et al., 2007). Participants were required to be present in the lab a total of four times. The first time served as a familiarization session to ensure that the participants could perform constant maximal voluntary contraction (MVIC) trials and to familiarize them with electrostimulation (EMS) of the tested muscle. For all MVICs performed by the participants, the instruction was to contract as hard and fast as possible. In addition, the supervisor gave maximal verbal encouragement. The different interventions (PAP, PAPEE, PAPEV) were randomized on the remaining test days. Between the test days, there had to be a break of at least one week to ensure that the interventions did not influence each other. Before each test, participants had to fill out a short questionnaire about their state of mind, means of transportation, and hydration status to check for irregularities.
Experimental ProtocolWarm-up
The standardized warm-up consisted of 60 active dynamic quadriceps stretches (3 sets of 20 repetitions) with a cadence of 1s in each direction. This warm-up was chosen as it was found to be the best option for injury prevention (Behm et al., 2016; Iwata et al., 2019; Zakaria et al., 2015) without raising the muscle and core temperature by the likes of cycling, treadmill, or similar warm-up (Bishop, 2003; Faulkner et al., 2013; Levels et al., 2012). Previous studies showed that a high increase in muscle temperature could affect the PAPE trials (Blazevich and Babault, 2019; Cuenca-Fernández et al., 2017). This warm-up was done during all sessions.
Post-Activation PotentiationAt first, a baseline stimulation was done with the subjects’ specific supramaximal stimulus. This was done twice with a 10s gap between the two twitches (Pääsuke et al., 2007). Peak torque of both twitches was compared to determine if both elicited a similar response (± 5%). Out of those two, the second one was chosen for future analysis. Then, the subject had to rest for five minutes to ensure that these two twitches did not affect the subsequent testing (Requena et al., 2008). An MVIC for 10s was performed afterward (Vandervoort et al., 1983), followed by a supramaximal twitch after two seconds, four minutes, eight minutes, and twelve minutes to see the potential time course of the PAP effect (Figure 2A).
Post-Activation Performance Enhancement–VoluntaryAt first, three maximal voluntary contractions (each 3s) with a rest period of four minutes in between (Salles et al., 2009) were performed as a baseline test. Out of those three, the one with the highest peak torque was chosen for future analysis. A break of 15 minutes was administered after these MVIC tests to ensure they would not influence the following steps (Lowery et al., 2012; Wilson et al., 2013). For the CC, an intensity of 80% of the MVIC was chosen (Garbisu-Hualde and Santos-Concejero, 2021; Wilson et al., 2013). A biofeedback application (ProActive, Prophysics AG, CH) ensured the subject held the CC constant at the desired torque level. The CC had to be repeated three times (Arabatzi et al., 2014; Lim and Kong, 2013; Seitz and Haff, 2016; Wilson et al., 2013) for a total of six seconds (Dobbs et al., 2019; Fukutani et al., 2013; Seitz et al., 2015) with a two-minute break in-between (Arabatzi et al., 2014; Lim and Kong, 2013; Rixon et al., 2007). Immediately two seconds after the last CC, the subject had to perform another MVIC, followed by another one at four, eight, and twelve minutes (each lasting 3s) to see the potential time course of PAPE (Figure 2B).
Post-Activation Performance Enhancement–ElectricalThe PAPEE trials consisted of the same steps as the PAPEV trial. Also, the same intensity, breaks, and the same volume was chosen for the CC. The only difference was that the CC was performed via electrical stimulation. After the baseline MVICs, the stimulation intensity was tested and set to match 80% of the baseline MVICs. A break of 15 minutes was administered after the stimulation testing to ensure it would not influence the following steps (Lowery et al., 2012; Wilson et al., 2013) (Figure 2C).
Electrical stimulationTwo self-adhesive stimulating electrodes were used to stimulate the quadriceps. One was placed proximal to the patella and the other distal to the hip joint on the front of the thigh (Figure 3) (Bentley et al., 2000; Sacilotto et al., 2017). Before electrostimulation, the respective leg was shaved, disinfected with alcohol, and smeared with a skin preparation gel for electrodes. The stimulation was performed with a constant current stimulator (DS7A - Constant Current Stimulator, Digitimer Ltd). For all tests, the voltage was set to 400V.
Electrical stimulation–PAPFor the PAP intervention, rectangular voltage pulses of two milliseconds were chosen. The intensity of the supramaximal stimulus was determined by gradually increasing the electrical current until the produced twitch torque reached a plateau (Miyamoto et al., 2010; Requena et al., 2008). To ensure that a supramaximal stimulus was used during the PAP trial, the final current was multiplied by a factor of 1.5. (MacIntosh et al., 2012).
Electrical stimulation–PAPEEFor the PAPEE intervention, an additional train generator (Model DG2A – Digitimer Ltd.) was used to generate a tetanic contraction. Therefore, a pulse duration of 200 microseconds at a frequency of 60 Hz was used. The duration of the stimulation was set to 6s. The stimulation intensity was set to match a contraction intensity of 80% MVIC.
ElectromyographyThe EMG signal of the rectus femoris (RF), vastus medialis (VM), and vastus lateralis (VL) was recorded for both PAPE conditions using a wired EMG system (AnEMG12, OT Bioelecttronica, IT). For the electrode placement, the SENIAM (Surface Electromyography for the Non-Invasive Assessment of Muscles) (Hermens et al., 2000) protocol was followed (Figure 2). The reference electrode was placed on the patella (Johnson et al., 2019) (Figure 2). Before testing, the respective leg was shaved, disinfected with alcohol, and smeared with a skin preparation gel for electrodes.
DynamometerFor the torque measurement, an isokinetic device (Isomed 2000, D&R Ferstl GmbH, Germany) was used (Figure 2). The participants were fixed on the device (shoulder pads, hip/ shoulder belts) so that unwanted movement was minimal, and only the necessary isometric knee extension could be performed without any limitation. The hip and knee joints were set at an angle of 80° of flexion (Hahn et al., 2007). The rotational axis of the dynamometer was carefully aligned with the knee joint axis of rotation.
Data collection and analysisData recording took place with the ProEMG software (ProEMG, Prophysics AG, CH) using a sample rate of 2000 Hz. Data filtering and analysis were conducted with a custom-written Python script. The EMG signal was recorded only during PAPEE and PAPEV trials. The signals were filtered using a second-order zero-lag Butterworth low- and high-pass filter with cut-off frequencies of 500Hz and 10Hz. Further, a root mean square smoothing was used with a window length of 250ms for calculating amplitude parameters (Konrad, 2006; Rose, 2019; Seiberl et al., 2016). The torque signal was smoothed using a root mean square window length of 20ms (Seiberl et al., 2016). The outcome variables that were calculated from this data were (a) peak torque (measured from baseline to the point of maximal torque (Johnson et al., 2019)), (b) rate of torque development (slope between 20-70% of maximal torque) (RTD) of each analyzed MVIC and supramaximal stimulus trial (PAP) and the (c) EMG peak values from the RF, VM, and VL of the quadriceps muscle for the MVIC trials. Furthermore, an overall activation of the quadriceps muscle (net-EMG) was calculated based on the sum of the weighted EMG signals of the RF, the VM, and VL (Seiberl et al., 2012; Seiberl et al., 2016). For RTD a possible confounder of analysis might be detecting the muscle on event during the contraction. Therefore, to minimize the impact of this confounder and increase the reliability of the analysis, RTD in the time window between 20-70% of peak torque during the rising part of the torque signal was calculated (Maffiuletti et al., 2016)
weighted EMG = (RFmeasured × 0.17 + (VLmeasured × 0.35) + (VMmeasured × 0.23)
Statistical analysisStatistics were done using R Studio (Rstudio, PBC, USA) and JASP (JASP, University of Amsterdam). The alpha level was set to p ≤ 0.05. The data was checked for extreme outliers, normality (Shapiro-Wilk test), and sphericity (if appropriate) (Mauchly’s test). To identify outliers in the dataset, box plots were utilized. Extreme outliers were excluded from the specific analysis. After exclusion of outliers, we had a total of 9 participants for analysis for the PAP trial. For PAPEV, we analyzed a total of 7 participants. For the peak torque and RTD parameters of PAPEE we analyzed 8 participants and for the net-EMG of the PAPEE trial a total of 7 participants. A repeated-measures ANOVA was used with subsequent simple contrast comparisons (if needed) to see if the values after two seconds (t2), four minutes (t3), eight minutes (t4), or twelve minutes (t5) were significantly different from baseline values (t1), for each condition separately. As effect size for the ANOVA, partial eta squared (η2p) was used. A η2p value of <0.06 was interpreted as a small effect, a value of 0.06-0.14 as a medium effect, and a value of >0.14 as a large effect.
Mean and standard deviation of the results can be found in Table 1 (Torque, RTD) and Table 2 (EMG).
Peak torqueDuring the PAP protocol, there was a significant change in the peak torque between the different time points, as revealed by the ANOVA (F(4,32) = 20.029, p < 0.001, η2p = 0.715). Simple contrast analysis showed a significant difference between the two-second timepoint and t1 (p < 0.001) but not for the other time points (p > 0.05). The peak torque value of t2 was 55.6% (SD 37.2%) higher than t1 (Figure 4A). For the PAPEE trial, there was no significant change in the peak torque values between the different time points (F(4,28) = 0.062, p = 0.992, η2p = 0.009) (Figure 3B). Same is true for the PAPEV condition (F(4,24) = 2.442, p = 0.074, η2p = 0.289) (Figure 4C).
Rate of torque developmentRTD showed a significant change between time points for the PAP trial (F(4,32) = 10.616, p = 0.005, η2p = 0.570). Simple contrast analysis showed a significant difference between t2 and t1 (p = 0.009) but not for the other time points. The RTD value of t2 was 63.7% (SD 37.5%) higher than t1 (Figure 5A). For the PAPEE and PAPEV conditions, no significant differences between the time points were found (F(4,28) = 0.456, p = 0.768, η2p = 0.061, F(4,24) = 1.173, p = 0.328, η2p = 0.164) (Figure 5B/C).
Peak EMGFor the PAPEV trial, the repeated measures ANOVA did not show any significant differences for the RF (F(4,24) = 2.130, p < 0.108, η2p = 0.262), the VM (F(4,24) = 0.641, p = 0.519, η2p = 0.096), the VL (F(4,24) = 2.083 p = 0.115, η2p = 0.258) or the net-EMG (F(4,24) = 1.616, p < 0.203, η2p = 0.212) between the measured time points (Figure 6B). The peak EMG of the RF (F(4,24) = 21.770, p = 0.001, η2p = 0.784), VM (F(4,28) = 12.096, p = 0.006, η2p = 0.633), VL (F(4,24) = 22.523, p = 0.002, η2p = 0.790) and the net-EMG (F(4,24) = 21.999, p = 0.002, η2p = 0.786) showed a significant difference between the time points of the PAPEE trial (Figure 6A). Simple contrast analysis showed a significant difference between t2 and t1 in the RF (p < 0.001), in the VM (p < 0.001), the VL (p = 0.002), and the net-EMG (p = 0.002). Important note for the results after the electrical CC: From Figure 6A, it seems that there is a considerable impact of the electrical CC on the EMG activity at t2 (increase up to ~ 695%). This effect is similar across all investigated muscles. Therefore, the authors think an interference between the electrical stimulation and the wired EMG system arbitrarily caused this increase. For clarity, we presented the results, including the activity at t2. However, for the rest of the manuscript, we exclude these results. Consequently, the ANOVA of the peak EMG analysis of the PAPEE condition, excluding results at t2, showed no differences for any muscle. By excluding t2 from the PAPEE trial, the peak EMG of the RF (F(3,18) = 1.330, p = 0.296, η2p = 0.181), VM (F(3,21) = 0.759, p = 0.530, η2p = 0.098), VL (F(3,18) = 1.468, p = 0.257, η2p = 0.197), and net-EMG (F(3,18) = 1.376, p = 0.282, η2p = 0.187) did not exhibit significant differences between the time points (Figure 7).
This study aimed to see if post-activation performance enhancement (PAPE) mechanisms are related to central processes and if mechanisms involved in the post-activation potentiation (PAP) also play a role. Despite having consistent results regarding PAP, this study did not reveal any effect for PAPE, irrespective of the type (voluntary vs. electrical stimulated) of the conditioning contraction (CC).
Post-Activation PotentiationLike in previous studies (Hamada et al., 2000; Requena et al., 2008; Vandervoort et al., 1983), the PAP effect was shown consistently in this study immediately following a voluntary CC by an increase in twitch torque of ~ 56% compared to baseline. Along with the increased twitch torque, the RTD of the twitch-induced contraction also increased significantly by ~ 64% compared to the baseline. This increase, both in peak torque and in RTD, is in line with the commonly proposed mechanism of PAP, namely the phosphorylation of the regulatory myosin light chain, which allows the myosin head to move closer to the actin-binding sites and increases the possibility of cross-bridge formation (Blazevich and Babault, 2019). Concerning the time course of the PAP effect, this study showed a significant increase in twitch torque and the measured RTD two seconds after the CC. However, the PAP effect was no longer present four to twelve minutes after the CC (Figure 4A and 5A). These findings are consistent with previous studies and reviews (Blazevich and Babault, 2019; Vandervoort et al., 1983). Blazevich and Babault (2019) summarized that the PAP-induced increase in twitch torque is short-lived. It is highest immediately after the CC and decreases dramatically over the next 28 seconds (Blazevich and Babault, 2019; Vandervoort et al., 1983). Due to the consistency of the immediate PAP effect among our participants, the effect can be classified as a general muscle property, at least for the quadriceps muscle group. However, the short lifetime makes a possible contribution to the PAPE effect that is usually detected four to twelve minutes after the CC implausible.
Post-Activation Performance EnhancementAt first, this study just used a minimalistic warm-up. There is an ongoing discussion in the literature if the warm-up itself and not the conditioning exercise is responsible for the PAPE effect. Usually, a comprehensive warm-up is often chosen as part of the protocol (Arabatzi et al., 2014; Esformes et al., 2011; Rixon et al., 2007; Seitz et al., 2014). However, Cuenca-Fernández et al. (2017) showed that a PAPE effect could be seen with a sufficient warm-up, even if the CC consists of quiet standing. Therefore, in this study, the warm-up was kept as short as possible to avoid an excessive increase in muscle temperature but long enough to prevent potential injuries. Hence, possible effects triggered by the CC could be classified more directly as the PAPE effect. As the proposed mechanism of PAP (Blazevich and Babault, 2019; Lorenz, 2011) seems to reside primarily inside the muscle and does not seem to involve neural mechanisms, the question arose if the same is true for PAPE. Therefore, this study compared an electrical CC with a voluntary CC matched for intensity and duration. With the study design, we assumed that effects on the peripheral side that increase muscle function (e.g., muscle temperature, calcium sensitivity) are similar between conditions. Hence, an increased effect after the voluntary CC compared to the stimulated CC would provide evidence for central processes involved in the PAPE effect. However, this study neither found increased values for peak torque nor RTD comparing different time points after the respective CC with the baseline values before the CC. Therefore, the conclusion concerning central processes remains to be seen. Peak torque is often used as a parameter reflecting the maximum strength capabilities of a participant of the involved muscles. Our participants showed similar values across all time points on the individual PAPE conditions. This shows a high familiarity and reliability in terms of MVC contractions. Skof and Strojnik (2007) showed that an intensive warm-up in middle-distance runners can increase the voluntary activation level by enhancing central nervous efficiency. By employing a minimalistic warm-up in this study, the observed elevation in peak torque could potentially be attributed to the PAPE effect induced by the voluntary CC. However, peak torque values did not change after the voluntary CC, and hence, the central nervous efficiency might not be altered by the CC. The EMG analysis further supports this, as peak EMG showed no changes across analyzed time points. The reader should note that our subjects were experienced in strength training and, therefore, might already have a high voluntary activation level, which might diminish potential effects concerning central nervous efficiency. However, this was not tested directly in this study. Although PAPE is often used as a term for a voluntary force or power enhancement (Blazevich and Babault, 2019), in most studies, the effect of PAPE has been researched in explosive movements like jumping or sprinting (Arabatzi et al., 2014; McCann and Flanagan, 2010; Till and Cooke, 2009). For that reason, it could be that there was no visible change in the peak torque during the tested MVIC trials, as PAPE might be most effective in the initial phase of a voluntary contraction. For explosive contractions, the rate of force development plays an important role. RFD reflects the increase in force/ torque directly after the onset of the contraction. Hence, RFD is a crucial parameter for many sports due to the time pressure to execute a specific task (e.g., sprint running, boxing), which is often below the time a muscle needs to develop maximal strength (> 300 ms). As discussed in the literature (Maffiuletti et al., 2016) and as mentioned in the methodology, the authors decided to analyze RTD in the time window between 20-70% of peak torque during the rising part of the torque signal to increase the reliability of the analysis. As can be seen in the Table 3, the endpoint of the analysis window (70% of peak torque) was below or slightly above 300ms (Table 3), representing activation dynamics quite well. In general, RFD is influenced by neural as well as contractile factors. As contractile factors like fiber type are unlikely to change within this study design, possible changes of RFD might be related to changes on the neuronal side and/or mechanisms associated with PAP immediately after the CC. However, RTD in both PAPE conditions did not show any significant results supporting the idea of increased neuronal efficiency or PAP mechanisms being involved. From a descriptive point of view, RTD values 2s after the end of the CC for the PAPEV condition show a steeper slope (~50%) compared to the baseline. However, as mentioned, ANOVA did not show a main effect concerning different time points; therefore, this trend needs further investigation. A reason why, for both PAPE trials, no significant differences were found could be that the PAPE effect, which does not occur immediately but after a specific break, is influenced by all the MVIC testing conducted before that time point. This could lead to an accumulation of fatigue that overshadows the actual PAPE effect in this study. However, this is speculative, as an increase in fatigue would likely be visible in peak torque values, but this is not the case in our data. Also, peak EMG values, especially at t3, t4, and t5, seem relatively stable, which further speaks against this point. A limitation of this study is the lack of temperature control of the thigh region. Since a precondition of this study was to exclude an extensive warm-up due to its possible effects on the PAPE trials, the temperature of the thigh region plays an important role. Minimalist warm-up and extended rest periods between contractions were used to not excessively increase muscle temperature, but this could not be adequately controlled. eBecause this study could not find an apparent effect regarding peak torque and rate of torque development, this may further point towards a temperature-dependent effect of PAPE. However, this remains speculative and is beyond the explanatory power of this study. Another limitation could be that the performed MVICs (pre- and post-testing) during the two PAPE trials could have had an enhancing effect themselves and therefore affect all the subsequent MVICs. We tried to limit this however by ensuring that subjects would have a sufficient break in between the trials, especially the 15min break between the pre-MVICs and the CCs. However, we cannot completely exclude this possibility of an enhancement. Lastly, we want to mention that with our methodology (PAPEE and PAPEV) it is not possible to strictly differentiate between central and peripheral factors. We think however, that with our setup, general comments about the predominant character of the contraction (peripheral or central) can be made.
A significant PAP effect was visible in this study regarding the peak torque and the RTD. However, a PAPE effect (voluntarily or electrically evoked) could not be identified for the chosen time points. Therefore, whether PAPE depends on neural mechanisms could not be answered, as the time points of PAPEE and PAPEV showed no significant peak torque or RTD changes. The assumption that the PAP mechanism influences PAPE can also not be confirmed, as no similarities between the time points for PAP and PAPE could be shown. Based on this finding, future research should distinguish between the terms post-activation potentiation and post-activation performance enhancement while considering the original research.
ACKNOWLEDGEMENTS |
Thanks to Josefina Manieu Seguel for her organizational help and great contribution during experimental testing. The authors declare no conflicts of interest. The present study complies with the current laws of the country in which it was performed. The datasets generated and analyzed during the current study are not publicly available but are available from the corresponding author, who was an organizer of the study. |
|
AUTHOR BIOGRAPHY |
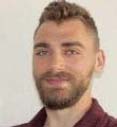 |
Josef Fischer |
Employment: Ph.D. student at the Institute of Human Movement Science, University of Graz. |
Degree: M.Sc. |
Research interests: Sports Biomechanics, fundamental muscle mechanics, muscle activation and hypertrophy during various exercise interventions. |
E-mail: josef.fischer@uni-graz.at |
|
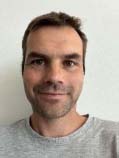 |
Florian K. Paternoster |
Employment: Associate Professorship of Biomechanics in Sports. Technical University of Munich |
Degree: PhD |
Research interests: Sports Biomechanics, fundamental muscle mechanics, stretch-shortening cycle, muscle-tendon unit. |
E-mail: florian.paternoster@tum.de |
|
|
|
REFERENCES |
 Aagaard P. (2003) Training-Induced Changes in Neural Function. Exercise and Sport Sciences Reviews 31, 61-67. Crossref
|
 Arabatzi F., Patikas D., Zafeiridis A., Giavroudis K., Kannas T., Gourgoulis V., Kotzamanidis C. (2014) The post-activation potentiation effect on squat jump performance: age and sex effect. Pediatric Exercise Science 26, 187-194. Crossref
|
 Beato M., Stiff A., Coratella G. (2021) Effects of Postactivation Potentiation After an Eccentric Overload Bout on Countermovement Jump and Lower-Limb Muscle Strength. Journal of Strength and Conditioning Research 35, 1825-1832. Crossref
|
 Behm D. G., Blazevich A. J., Kay A. D., McHugh M. (2016) Acute effects of muscle stretching on physical performance, range of motion and injury incidence in healthy active individuals: a systematic review. Applied Physiology, Nutrition and Metabolism 41, 1-11. Crossref
|
 Bentley D. J., Smith P. A., Davie A. J., Zhou S. (2000) Muscle activation of the knee extensors following high intensity endurance exercise in cyclists. European Journal of Applied Physiology 81, 297-302. Crossref
|
 Bishop D. (2003) Warm up II: performance changes following active warm up and how to structure the warm up. Sports Medicine 33, 483-498. Crossref
|
 Blazevich A. J., Babault N. (2019) Post-activation Potentiation Versus Post-activation Performance Enhancement in Humans: Historical Perspective, Underlying Mechanisms and Current Issues. Frontiers in Physiology 10, 1359. Crossref
|
 Brown G. L., Euler U. S. (1938) The after effects of a tetanus on mammalian muscle. The Journal of Physiology 93, 39-60. Crossref
|
 Chaouachi A., Haddad M., Castagna C., Del Wong P., Kaouech F., Chamari K., Behm D. (2011) Potentiation and recovery following low- and high-speed isokinetic contractions in boys. Pediatric Exercise Science 23, 136-150. Crossref
|
 Contreras B., Vigotsky A. D., Schoenfeld B. J., Beardsley C., Cronin J. (2015) A comparison of two gluteus maximus EMG maximum voluntary isometric contraction positions. PeerJ 3, e1261. Crossref
|
 Cuenca-Fernández F., Smith I. C., Jordan M. J., MacIntosh B. R., López-Contreras G., Arellano R., Herzog W. (2017) Nonlocalized postactivation performance enhancement (PAPE) effects in trained athletes: a pilot study. Applied Physiology, Nutrition and Metabolism 42, 1122-1125. Crossref
|
 Dobbs W. C., Tolusso D. V., Fedewa M. V., Esco M. R. (2019) Effect of Postactivation Potentiation on Explosive Vertical Jump: A Systematic Review and Meta-Analysis. Journal of Strength and Conditioning Research 33, 2009-2018. Crossref
|
 Edman K. A. P., Hwang J. C. (1977) The force-velocity relationship in vertebrate muscle fibres at varied tonicity of the extracellular medium. The Journal of Physiology 269, 255-272. Crossref
|
 Elmubarak M. H., Ranatunga K. W. (1984) Temperature sensitivity of tension development in a fast-twitch muscle of the rat. Muscle Nerve 7, 298-303. Crossref
|
 Esformes J. I., Keenan M., Moody J., Bampouras T. M. (2011) Effect of different types of conditioning contraction on upper body postactivation potentiation. Journal of Strength and Conditioning Research 25, 143-148. Crossref
|
 Faulkner S. H., Ferguson R. A., Gerrett N., Hupperets M., Hodder S. G., Havenith G. (2013) Reducing muscle temperature drop after warm-up improves sprint cycling performance. Medicine and Science in Sports and Exercise 45, 359-365. Crossref
|
 Fukutani A., Miyamoto N., Kanehisa H., Yanai T., Kawakami Y. (2013) Potentiation of isokinetic torque is velocity-dependent following an isometric conditioning contraction. SpringerPlus 2. Crossref
|
 Garbisu-Hualde A., Santos-Concejero J. (2021) Post-Activation Potentiation in Strength Training: A Systematic Review of the Scientific Literature. Journal of Human Kinetics 78, 141-150. Crossref
|
 González-Alonso J., Quistorff B., Krustrup P., Bangsbo J., Saltin B. (2000) Heat production in human skeletal muscle at the onset of intense dynamic exercise. The Journal of Physiology 524, 603-615. Crossref
|
 Gouvêa A. L., Fernandes I. A., César E. P., Silva W. A. B., Gomes P. S. C. (2013) The effects of rest intervals on jumping performance: a meta-analysis on post-activation potentiation studies. Journal of Sports Sciences 31, 459-467. Crossref
|
 Hahn D., Seiberl W., Schwirtz A. (2007) Force enhancement during and following muscle stretch of maximal voluntarily activated human quadriceps femoris. European Journal of Applied Physiology 100, 701-709. Crossref
|
 Hamada T., Sale D. G., MacDougall J. D., Tarnopolsky M. A. (2000) Postactivation potentiation, fiber type and twitch contraction time in human knee extensor muscles. Journal of Applied Physiology 88, 2131-2137. Crossref
|
 Hermens H., Freriks B., Merletti R., Stegeman D., Blok J., Rau G. (2000) Development of recommendations for SEMG sensors and sensor placement procedures. Journal of Electromyography and Kinesiology 10, 361-374. Crossref
|
 Iwata M., Yamamoto A., Matsuo S., Hatano G., Miyazaki M., Fukaya T., Fujiwara M., Asai Y., Suzuki S. (2019) Journal of Sports Science & Medicine. Pubmed
|
 Johnson M., Baudin P., Ley A. L., Collins D. F. (2019) A Warm-Up Routine That Incorporates a Plyometric Protocol Potentiates the Force-Generating Capacity of the Quadriceps Muscles. Journal of Strength and Conditioning Research 33, 380-389. Crossref
|
 Konrad P. (2006) The ABC of EMG. A Practical Introduction to Kinesiological Electromyography.
|
 Krzysztofik M., Wilk M., Stastny P., Golas A. (2020) Post-activation Performance Enhancement in the Bench Press Throw: A Systematic Review and Meta-Analysis. Frontiers in Physiology 11, 598628. Crossref
|
 Levels K., Koning J. J., Foster C., Daanen H. A. M. (2012) The effect of skin temperature on performance during a 7.5-km cycling time trial. European Journal of Applied Physiology 112, 3387-3395. Crossref
|
 Lim J. J., Kong P. W. (2013) Effects of isometric and dynamic postactivation potentiation protocols on maximal sprint performance. Journal of Strength and Conditioning Research 27. Crossref
|
 Lorenz D. (2011) Postactivation Potentiation: An Introduction. International Journal of Sports Physical Therapy 6, 234-240.
|
 Lowery R. P., Duncan N. M., Loenneke J. P., Sikorski E. M., Naimo M. A., Brown L. E., Wilson F. G., Wilsk (2012) The effects of potentiating stimuli intensity under varying rest periods on vertical jump performance and power. Journal of Strength and Conditioning Research 26, 3320-3325. Crossref
|
 MacIntosh B. R., Robillard M. E., Tomaras E. K. (2012) Should postactivation potentiation be the goal of your warm-up?. Applied Physiology, Nutrition and Metabolism 37, 546-550. Crossref
|
 Maffiuletti N, A., Aagaard P., Blazevich A. j., Folland J., Tilin N., Duchateau J. (2016) Rate of force development: physiological and methodological considerations. European Journal of Applied Physiology 116, 1091-1116. Crossref
|
 McCann M. R., Flanagan S. P. (2010) The effects of exercise selection and rest interval on postactivation potentiation of vertical jump performance. Journal of Strength and Conditioning Research 24, 1285-1291. Crossref
|
 Metzger J. M., Greaser M. L., Moss R. L. (1989) Variations in cross-bridge attachment rate and tension with phosphorylation of myosin in mammalian skinned skeletal muscle fibers. Implications for twitch potentiation in intact muscle. The Journal of General Physiology 93, 855-883. Crossref
|
 Mina M. A., Blazevich A. J., Giakas G., Seitz L. B., Kay A. D. (2016) Chain-loadedvariable resistance warm-up improves free-weight maximal back squat performance. European Journal of Sport Science 16, 932-939. Crossref
|
 Mitchell C. J., Sale D. G. (2011) Enhancement of jump performance after a 5-RM squat is associated with postactivation potentiation. European Journal of Applied Physiology 111, 1957-1963. Crossref
|
 Miyamoto N., Mitsukawa N., Sugisaki N., Fukunaga T., Kawakami Y. (2010) Joint angle dependence of intermuscle difference in postactivation potentiation. Muscle & Nerve 41, 519-523. Crossref
|
 Nuzzo J. L., Barry B. K., Gandevia S. C., Taylor J. L. (2016) Acute Strength Training Increases Responses to Stimulation of Corticospinal Axons. Medicine & Science in Sports & Exercise 48, 139-150. Crossref
|
 Pasquet B., Carpentier A., Duchateau J., Hainaut K. (2000) Muscle fatigue during concentric and eccentric contractions. Muscle & nerve 23, 1727-1735. Crossref
|
 Persechini A., Stull J. T., Cooke R. (1985) The effect of myosin phosphorylation on the contractile properties of skinned rabbit skeletal muscle fibers. Journal of Biological Chemistry 260, 7951-7954. Crossref
|
 Prieske O., Behrens M., Chaabene H., Granacher U., Maffiuletti N. A. (2020) Time to Differentiate Postactivation "Potentiation" from "Performance Enhancement" in the Strength and Conditioning Community. Sports Medicine 50, 1559-1565. Crossref
|
 Pääsuke M., Saapar L., Ereline J., Gapeyeva H., Requena B., Ööpik V. (2007) Postactivation potentiation of knee extensor muscles in power- and endurance-trained and untrained women. European Journal of Applied Physiology 101, 577-585. Crossref
|
 Requena B., Gapeyeva H., García I., Ereline J., Pääsuke M. (2008) Twitch potentiation after voluntary versus electrically induced isometric contractions in human knee extensor muscles. European Journal of Applied Physiology 104, 463-472. Crossref
|
 Requena B., Sáez-Sáez de Villarreal E., Gapeyeva H., Ereline J., García I., Pääsuke M. (2011) Relationship between postactivation potentiation of knee extensor muscles, sprinting and vertical jumping performance in professional soccer players. Journal of Strength and Conditioning Research 25, 367-373. Crossref
|
 Rixon K. P., Lamont H. S., Bemben M. G. (2007) Influence of type of muscle contraction, gender and lifting experience on postactivation potentiation performance. Journal of Strength and Conditioning Research 21, 500-505. Crossref
|
 Rose W. (2019) Electromyogram analysis. https://www1.udel.edu/biology/rosewc/kaap686/notes/EMG%20analysis.pdf
|
 Sacilotto M. C. B., Lavagnoli C. F. R., Silveira-Filho L. M., Vilarinho K. A. d. S., Oliveira E. S. B., de Carvalho D. D., Oliveira P. P. M., Coelho-Filho O. R., Junior O. P. (2017) A Simpler and Shorter Neuromuscular Electrical Stimulation Protocol Improves Functional Status and Modulates Inflammatory Profile in Patients with End-Stage Congestive Heart Failure. International Journal of Cardiovascular Sciences. Crossref
|
 Salles B. F., de Simão R., Miranda F., Da Novaes J. S., Lemos A., Willardson J. M. (2009) Rest interval between sets in strength training. Sports Medicine 39, 765-777. Crossref
|
 Seiberl W., Hahn D., Herzog W., Schwirtz A. (2012) Feedback controlled force enhancement and activation reduction of voluntarily activated quadriceps femoris during sub-maximal muscle action. Journal of Electromyography and Kinesiology 22, 117-123. Crossref
|
 Seiberl W., Hahn D., Paternoster F. K. (2016) Reduced activation in isometric muscle action after lengthening contractions is not accompanied by reduced performance fatigability. Scientific Reports 6, 39052. Crossref
|
 Seitz L. B., Haff G. G. (2016) Factors Modulating Post-Activation Potentiation of Jump, Sprint, Throw and Upper-Body Ballistic Performances: A Systematic Review with Meta-Analysis. Sports Medicine 46, 231-240. Crossref
|
 Seitz L. B., Trajano G. S., Dal Maso F., Haff G. G., Blazevich A. J. (2015) Postactivation potentiation during voluntary contractions after continued knee extensor task-specific practice. Applied Physiology, Nutrition and Metabolism 40, 230-237. Crossref
|
 Seitz L. B., Villarreal E. S., de Haff G. G. (2014) The temporal profile of postactivation potentiation is related to strength level. Journal of Strength and Conditioning Research 28, 706-715. Crossref
|
 Siddique U., Frazer A., Avela J., Walker S., Ahtiainen J., Howatson G., Tallent J., Kidgell D. J. (2022) Determining the cortical, spinal and muscular adaptations to strength-training in older adults: A systematic review and meta-analysis. Ageing Research Reviews 82. Crossref
|
 Stein R. B., Gordon T., Shriver J. (1982) Temperature dependence of mammalian muscle contractions and ATPase activities. Biophysical Journal 40, 97-107. Crossref
|
 Sugi H., Chaen S., Akimoto T., Minoda H., Miyakawa T., Miyauchi Y., Tanokura M., Sugiura S. (2015) Electron microscopic recording of myosin head power stroke in hydrated myosin filaments. Scientific Reports 5, 15700. Crossref
|
 Till K. A., Cooke C. (2009) The effects of postactivation potentiation on sprint and jump performance of male academy soccer players. Journal of Strength and Conditioning Research 23, 1960-1967. Crossref
|
 Tillin N. A., Bishop D. (2009) Factors modulating post-activation potentiation and its effect on performance of subsequent explosive activities. Sports Medicine 39, 147-166. Crossref
|
 Timon R., Allemano S., Camacho-Cardeñosa M., Camacho-Cardeñosa A., Martinez-Guardado I., Olcina G. (2019) Post-Activation Potentiation on Squat Jump Following Two Different Protocols: Traditional Vs. Inertial Flywheel. Journal of Human Kinetics 69, 271-281. Crossref
|
 Vandervoort A. A., Quinlan J., McComas A. J. (1983) Twitch potentiation after voluntary contraction. Experimental Neurology 81, 141-152. Crossref
|
 Wang L., Bahadir A., Kawai M. (2015) High ionic strength depresses muscle contractility by decreasing both force per cross-bridge and the number of strongly attached cross-bridges. Journal of Muscle Research and Cell Motility 36, 227-241. Crossref
|
 Wilson J. M., Duncan N. M., Marin P. J., Brown L. E., Loenneke J. P., Wilson S. M. C., Jo E., Lowery P. R., Ugrinowitsch C. (2013) Meta-analysis of postactivation potentiation and power: effects of conditioning activity, volume, gender, rest periods and training status. Journal of strength and conditioning research 27, 854-859. Crossref
|
 Zakaria A. A., Kiningham R. B., Sen A. (2015) Effects of Static and Dynamic Stretching on Injury Prevention in High School Soccer Athletes: A Randomized Trial. Journal of sport rehabilitation 24, 229-235. Crossref
|
 Škof B., Strojnik V. (2007) The Effect of Two Warm-Up Protocols on Some Biomechanical Parameters of the Neuromuscular System of MiddleDistance Runners. Journal of Strength and Conditioning Research 21, 394. Crossref
|
|
|
|
|
|
|