|
|
|
ABSTRACT |
Repeated-sprint training in hypoxia (RSH) has been shown to boost team-sport players’ repeated-sprint ability (RSA). Whether players’ global inspiratory muscle (IM) and core muscle (CM) functions would be altered concomitantly with RSH was not reported. This study was designed to compare the concomitant alternations in players’ RSA and their IM and CM functions during a team-sport-specific intermittent exercise protocol (IEP) before and after the intervention. Twenty players were assigned into either RSH or control (CON) groups (n = 10 for each). RSH players participated in 5-wk RSH (15 sessions, 3 sets 5x5-s all-out treadmill sprints interspersed with 25-s passive recovery under the hypoxia of 13.5%) while CON players had no corresponding training. The changes in RSA between pre- and post-intervention, and the alterations in IM and CM functions that were revealed by maximum inspiratory mouth pressure (PImax) and sport-specific endurance plank test (SEPT) performance, respectively, between pre- and post-IEP and across pre- and post-intervention in the RSH group were compared with that of CON. Following the 5-wk RSH, players’ RSA improved significantly (>6%, p < 0.05) while PImax and SEPT performance did not alter (P > 0.05). Nevertheless, PImax which declined markedly in pre-intervention IEP (pre-IEP 155.4 ± 22.7 vs post-IEP 140.6 ± 22.8 cmH2O, p < 0.05) was alleviated significantly in post-intervention IEP (152.2 ± 27.4 vs 152.6 ± 31.8, p > 0.05), while the concomitant declined SEPT performance in the pre-intervention IEP (155 ± 24.6 vs 98.1 ± 21.7 s, p < 0.05) was retained post intervention (170.7 ± 38.1 vs 100.5 ± 33.4, p < 0.05). For the CON, all variables were unchanged (p > 0.05). Such findings suggest that 5-wk RSH could enhance players’ RSA but not global IM and CM functions. Nonetheless, the decline in PImax in pre-intervention IEP alleviated significantly post intervention led to a postulation that players’ IM endurance, rather than strength, might improve with the 5-wk RSH regimen, while the possible improved IM endurance did not advance the fatigue resistance of CM. |
Key words:
Intermittent hypoxic training, inspiratory muscle strength, respiratory muscle training, maximum inspiratory mouth pressure, sport-specific endurance plank test
|
Key
Points
- 5-wk repeated-sprint training in hypoxia (RSH) enhanced team-sport players’ repeated sprint ability in a team-sport-specific intermittent exercise protocol (IEP), while their global inspiratory muscle strength did not alter.
- Nevertheless, IEP-induced inspiratory muscle fatigue in players alleviated significantly following RSH.
- However, the alleviation of global inspiratory muscle fatigue following RSH did not advance the fatigue resistance of core muscles during the IEP.
|
It is known that the repeated-sprint ability (RSA) of players in intermittent-type sports such as football and handball is of the essence in determining their competitive edge in matches (Mohr et al., 2005). In order to maximize players’ performance, various repeated-sprint training (RST) regimens are inevitably adopted by sports teams in different training periods (Ferrari Bravo et al., 2008; Turner and Stewart, 2013). In the last decade, numerous investigations supported the notion that RST carried out in a hypoxic condition could boost the enhancement effects of RST on players’ sea-level RSA (Brocherie et al., 2017). The boosting effects of repeated-sprint training in hypoxia (RSH) on players’ RSA are mainly attributed to the cellular and molecular adaptive mechanisms emerged in exercising muscles under severe hypoxia that eventually enhance the O2 availability and utilization of the fast-twitch fibres and promote their resynthesis of phosphocreatine following every all-out sprint (Faiss et al., 2013; Millet et al., 2019). However, the extra stress induced by repeated sprints in hypoxia was not solely imposed on working muscles, the reduced oxygen availability might also influence other physiological responses such as oxidative stress and ventilation (Gatterer et al., 2019). In hypoxia, the diminished arterial O2 pressure triggers the compensatory response of increased pulmonary ventilation to maintain O2 delivery to the tissues (Constantini et al. 2021). As a result, work of breathing increases. The increase in work of breathing coupling with the reduced O2 transport to the respiratory muscles as during heavy endurance exercise in hypoxia have been shown to exaggerate inspiratory muscle (IM) fatigue, mainly the diaphragmatic fatigue (Vogiatzis et al., 2007). Nonetheless, the long-term strenuous ventilatory work elicited during exercise in other normoxia scenarios has been shown effective in enhancing one’s respiratory muscle function. It was found that the aggregate ventilatory demand during 4-wk high-intensity interval training could improve untrained healthy individuals’ respiratory muscle strength and endurance (Dunham and Harms, 2012; Yalman et al., 2021). However, the ventilatory demand during short-term repeated-sprint cycling in normoxia was found not to induce respiratory muscle fatigue (Minahan et al., 2015), implying less effect on enhancement of the muscle function with repeated-sprint training. Notwithstanding, in an animal model of sprint interval training in hypoxia, additional ventilatory load resulted in greater increase in diaphragm oxidative capacity compared with normoxia (Ogura et al., 2005). It has been reported that the ventilatory work of athletes’ global IM and associated sensation of breathing effort are the factors limiting their exercise tolerance in high-intensity intermittent exercise (Tong et al., 2003; 2004). Enhanced IM function through 4-6 weeks pressure-threshold IM training could improve their tolerance of high- intensity intermittent bouts of running (Tong et al., 2008; 2010). Whether the added heavy ventilatory work during short-term RSH would enhance the global IM function and hence contribute to the boosting effects of RSH on sea-level RSA have not been investigated. In humans, both inspiratory and expiratory muscles, apart from the performance of breathing work, are also recruited for maintaining core stability in many non-respiratory activities (Al-bilbeisi and McCool, 2000). Specifically, the respiratory muscles, in sports and exercise settings, usually jointly work with other core muscles (CM) to optimize the transfer of energy to the extremities by controlling the position and motion of the torso over the pelvis (Kibler et al., 2006). During RSH, ventilatory demand increases. It was not known if, under such severe ventilatory stress, the dual role of the respiratory muscles would lead to bilateral enhancement in global IM and CM functions following the short-term RSH. The purposes of this study were to examine the effects of 5-wk RSH on team-sport players’ RSA and their global IM and CM functions. The alterations in the RSA as well as the functions of the two muscle groups during a team-sport-specific intermittent exercise protocol (IEP) were compared before and after the intervention. It was hypothesised that 5-wk RSH would concomitantly enhance players’ RSA and their IM and CM functions. The improved muscle functions would promote their fatigue resistance during the IEP.
ParticipantsDue to the possible gender difference in the sensitivity to the arterial O2 desaturation (Billaut and Smith, 2009), only male participants were included in the present study. 20 university team-sport players (football: 12, handball: 8) who volunteered to participate in this study were assigned into either experimental or control groups (n = 10 for each). The sample size was estimated by the priori, two-tailed power calculation at an alpha of 0.05 and a power of 80%, and based on our previous study findings of alterations in maximal static inspiratory pressure (ES: 2.21) following IM training in team-sport players (Tong et al., 2008). After being fully informed of the experimental procedures and possible exercise- and blood test-induced discomfort, players gave their written informed consent. Ethical approval for this study was obtained from the Committee on the Use of Human and Animal Subjects in Teaching and Research at Macao Polytechnic University. The study was conducted in accordance with the Declaration of Helsinki.
Experimental designFigure 1 is the overview of the study design. Within a week prior to the intervention, players’ RSA during the IEP, and their IM and CM functions before and after the IEP were assessed. Afterwards, each two players with matched pre-IEP IM function were randomly assigned into RSH or CON group. For examining the effects of RSH on players’ RSA and their IM and CM functions during the IEP, 5-wk RSH was implemented in RSH group. The RSH was carried out on a non-motorized treadmill (Force 3, Woodway, USA) which was placed inside a normobaric hypoxic chamber (HS500, Welltech, China). Players were blinded to the hypoxic level throughout the study. CON players did not participate in the repeated-sprint training while the sport-team training of both groups during the intervention period were identical. Following two days after the last training session, the RSA and muscle function assessments were repeated in both groups. The changes in the RSA between pre- and post-intervention, and the alterations in IM and CM functions between pre- and post-IEP and across pre- and post-intervention in the RSH group were compared with that of CON. For controlling the diurnal variation influences, all exercise tests were scheduled at the same time of day. In avoidance of residual fatigue induced by preceded exercise test, all the tests were separated by a minimum of two days. During the study period, all players were instructed to maintain their dietary habits. Prior to each test, players refrained from eating for at least two hours, and were prohibited to take any additional energy supplements.
ProceduresPreliminary tests and familiarization trials
Prior to the experimental trials, players’ physical characteristics including lung function, aerobic capacity and percentage of body fat were assessed. Afterwards, the maximum treadmill braking resistance (TRmax) and the maximal sprint velocity of the players on the Woodway non-motorized treadmill were determined individually for subsequent treadmill ergometry use (Shi et al., 2023). The TRmax (kp) was defined as the maximum resistance of the treadmill belt that the players can move consecutively for three steps. The maximal sprint velocity was measured by performing three maximal 4-s sprints on the treadmill at 5% TRmax, each separated by 14 s of standing recovery. The highest velocity obtained in a single second from the three sprints was the result (Zois et al., 2013). Following preliminary testing, players were familiarized with the assessments of the IM and CM functions, as well as the IEP. The familiarization trial introduced the testing equipment and protocols of the specific muscle function tests to the players, and provided them with experience of exercising at assigned velocities of walking and running on the treadmill in the IEP.
Experimental trialsInspiratory muscle and core muscle function tests
The maximum inspiratory mouth pressure at quasi-zero flow (PImax in cmH2O) provided a surrogate measure of global IM strength (Laveneziana et al., 2019). It was measured by performing maximal inspiratory efforts at residual volume against a semi-occluded rubber-scuba-type mouthpiece with a 1 mm orifice. The change in the mouth pressure during the maximal inspiratory maneuver was detected by a differential pressure transducer coupled with a signal conditioner (Collins, Braintree, MA, USA). The maximal inspiratory efforts were repeated at least 5 times until the results were stable (vary by <10% in consecutive three maneuvers), and the highest value was recorded for analysis. Global CM function was assessed by complying with the protocol of the sport-specific endurance plank test (SEPT) reported previously (Tong et al., 2014a). This test requires players to maintain the prone bridge in good form throughout the following stages with no rest in between: (a) hold the basic plank position for 60 s; (b) lift the right arm off the ground and hold for 15 s; (c) return the right arm to the ground and lift the left arm for 15 s; (d) return the left arm to the ground and lift the right leg for 15 s; (e) return the right leg to the ground and lift the left leg for 15 s; (f) lift both the left leg and right arm from the ground and hold for 15 s; (g) return the left leg and right arm to the ground, and lift both the right leg and left arm off the ground for 15 s; (h) return to the basic plank position for 30 s; (i) repeat the steps from (a) to (i) until the maintenance of the prone bridge failed. The formation of the prone bridge during the SEPT in all trials were standardized by using identical body posture. It referred to the distances between the left and right elbows (medial epicondyle), the left and right feet (1st metatarsal), the elbow and feet on the left and right sides of the body, and the hip position. The distances among elbows and feet were measured during the familiarization trial while players were comfortably performing the prone bridge on a bench. Further, two elastic strings of ~80 cm length which were attached horizontally to a pair of vertical scales were placed beside the bench during the test. The two strings maintained at a distance of 10 cm were adjusted up and down until a height was reached that was at the same level as the players’ hip (the iliac crest was evenly in between the two strings). This setting acted as a reference for the objective monitoring of hip displacement during the test. The measured distances among the elbows and feet, as well as the hip height, were consistent in all experimental trials. During the assessment, the test administrator sat one meter away from the bench with the seat height adjusted to a level so that the hip displacement of the players could be monitored horizontally. The players were then asked to maintain the prone bridge throughout the test with maximum effort. For each time that the hip was beyond either of the reference lines, a verbal warning was given. The test was terminated when the hip failed to be maintained at the required level after receiving two consecutive warnings. The measured time to the limit of tolerance was used as the index of global CM function.
Team-sport-specific intermittent exercise protocolIEP was performed on the Woodway non-motorized treadmill with braking resistance set at 5% TRmax for ~30 min (Shi et al., 2023). It was interspersed with 42 8-s standing still (19.2% overall duration), 75 8-s walking (34.2%), 56 8-s jogging (25.6%), 36 6-s running (12.3%), 20 4-s dashing (4.6%), and 24 3-s sprinting (4.1%) activities. The speed for each foregoing activity was defined at 0%, 20%, 35%, 50%, 70% and 100% of the maximal sprint velocity, respectively. During the IEP, all players were required to perform the six activities by complying with the velocity assigned. Figure 2 is the order of the activities in the IEP and the assigned velocity of each activity of a sample player whose maximal sprint velocity was 5.4 m·.s-1. In addition, 4 sets of RSA test each composed of 3 x 4-s all-out sprints at 20% TRmax, with 14-s passive recovery in between, were incorporated into the IEP at the beginning (1 set), middle (2 sets), and end (1 set) of the protocol. During each sprint, the alterations in the velocity and power output were recorded at 25 Hz using the Woodway Force 3.0 software. The average of the mean velocity (M-Vel) and mean power output (M-Po) of the sprints were the players’ performance. Besides, the total work done (WTot) of the IEP (excluded the 4 sets of RSA test) was also recorded using the same software. Before the IEP, pre-IEP PImax and SEPT performance were assessed. After that, a standardized warm-up exercises including a 5-min motorized treadmill run at moderate intensity and a 5-min dynamic stretching exercise mainly using the legs were performed. Following capillary sampling from fingertip for blood lactate measurement, and performance of one bout of each activity and three bouts of 5-s submaximal sprints on the non-motorized treadmill for warm-up purposes, the IEP commenced. Exercise heart rate (HR) was monitored using a Polar HR monitor (H6, Finland). Five minutes after the IEP, identical blood sampling was repeated for examining the change in blood lactate (ΔLa) using the Lactate Scout+ analyzer (EKF Diagnostics, UK). Following the blood sampling, players were required to score from 0 to 10 based on the Borg CR-10 scale (Borg et al., 1985) on how hard they perceived the IEP - ratings of perceived exertion (RPE), and how heavy they sensed their breathing effort during the exercise - ratings of perceived breathlessness (RPB). The session RPE (s-RPE) and RPB (s-RPB) for quantifying the associated internal exercise stress were then calculated as:
s-RPE/RPB (AU) = RPE/RPB score * duration of the IEP (minute) (McLaren et al., 2017)
Immediately following the perceptual ratings, post-IEP PImax measurement and SEPT were carried out successively.
Repeated-sprint training in hypoxiaThe RSH protocol has been reported in our recent study (Shi et al., 2023). Briefly, each RSH session consisted of three sets of 5 x 5-s sprints interspersed with 25-s passive recovery at the ratio of exercise to recovery of 1:5, and with 5-min passive recovery in between sets; one session per day, three days per week for five weeks (Figure 1). The specific RSH protocol has been shown effective to induce maximum stress of hypoxia in players during the training, and to enhance their RSA (Tong et al. 2021; Shi et al., 2023). The repeated sprints were performed on the same Woodway non-motorized treadmill with the braking resistance set at 20% of TRmax. All the trainings were taken place in the Welltech normobaric, hypoxic chamber (35 m2, 105 m3) with the O2 concentration inside the chamber set at 13.5%. During the intervention, all players were blinded to the hypoxic level. Prior to the repeated-sprint exercise in each session, players’ peripheral capillary O2 saturation (SpO2) was monitored using a pulse oximeter (Masimo, USA) for two minutes while they were resting on a chair in the hypoxic chamber. The SpO2 was recorded at the end of the monitoring period. Thereafter, following three bouts of 5-s submaximal sprints (70-90% all-out effort) on the non-motorized treadmill for warm-up purposes, the RSH protocol commenced. The mean power output of each set of the repeated sprints was recorded. At the time when the average of the mean power output of the three sets increased significantly from original level in consecutive two sessions, the treadmill resistance would be increased with each increment of 1 kp.
Statistical analysesThe Shapiro-Wilk normality test revealed that data for all variables were normally distributed. The differences in physical characteristics between the RSH and CON groups (Table 1) were assessed using paired sample t test. To examine the differences in the exercise stress variables and the perceptual variables, as well as the exercise performance variables of the IEP between the pre- and post-intervention trials and across the RSH and CON groups (Table 2 and Table 3), two-way ANOVA with repeated measures were computed. For the PImax and SEPT (Figure 3 and Figure 4), the differences between Pre- and Post-IEP, across trials, and among groups were assessed by applying three-way ANOVA with repeated measures in two factors (IEP and trials). Post hoc analyses for ANOVA, using the Bonferroni test for identifying simple main effects, were performed when a significant interaction was detected. Partial eta squared (ηρ²) was used to indicate the effect size and to measure the main and interaction effects, where values of 0.04 = small, 0.25 = medium, and 0.64 = large effect size (Ferguson, 2009). The effect size of pairwise comparison was revealed by calculating Cohen's d, where d = 0.2, 0.5, and 0.8 indicate small, medium, and large effect sizes, respectively (Cohen, 1988). Statistical significance was set at p < 0.05, and values are reported as means ± SD.
In this study, players’ physical characteristics (Table 1) and their TRmax (30.0 ± 3.1 vs 31.2 ± 1.6 kp) were not varied between RSH and CON. Both groups completed all the experimental trials and intervention. No adverse events were reported in either group. During the 5-wk intervention, RSH players participated in the specific training with attendance of 100%. Two participants had increased their treadmill resistance training load by 1 kp, others were maintained at original level throughout the intervention. For the hypoxic condition during the 5-wk RSH, the averaged O2 partial pressure in the hypoxic chamber was 13.8 ± 1.0%, and the SpO2 of the players was 90.1 ± 1.9%. Table 2 shows the post-exercise HR, s-RPB, s-RPE and ΔLa during the IEP in the pre- and post-intervention trials in RSH and CON. All the variable prior to the intervention were not varied between RSH and CON. In comparison to pre-intervention level, post-exercise HR and ΔLa increased significantly (Cohen’s d = 0.62, 1.06) in post-intervention trial in RSH, but not CON. For the perceptual variables of s-RPB and s-RPE, no alteration was found post intervention in both groups. The WTot, M-Vel and M-Po of RSH and CON during the IEP in the pre- and post-intervention trials are shown in Table 3. In the pre-intervention trial, the three variables were not different between the RSH and CON. Following the intervention, significant increase in WTot, M-Vel and M-Po (Cohen’s d = 0.88, 1.33, 1.49) were observed in RSH, while it did not alter in CON. The alterations in the players’ PImax and in their performance of SEPT following IEP in the pre- and post-intervention trials are shown, respectively, in Figure 3 and Figure 4. In the pre-intervention trial, the PImax of RSH and CON, which were not varied before the IEP, declined after the exercise in similar magnitude (RSH: pre-IEP 155.4 ± 22.8, post-IEP 140.6 ± 22.8 cmH2O, p < 0.05, Cohen’s d = 0.65; CON: 152.8 ± 17.5, 142.7 ± 20.1 cmH2O, p < 0.05, Cohen’s d = 0.51). Following the intervention, the pre-IEP PImax did not change significantly from the pre-intervention level in both groups. However, the decline in the PImax after completing the IEP was attenuated in RSH (152.2 ± 27.4, 152.6 ± 31.8 cmH2O, p > 0.05). The attenuation was not observed in CON (156.5 ± 17.8, 147.6 ± 22.7 cmH2O, p < 0.05, Cohen’s d = 0.44). For the global CM function, players’ performance in the SEPT prior to the pre-intervention IEP was not different between RSH and CON. The SEPT performance declined significantly in the players after the IEP (RSH: pre-IEP 155 ± 24.6, post-IEP 98.1 ± 21.7 s, p < 0.05, Cohen’s d = 2.45; CON: 171.3 ± 43, 117.7 ± 28.4 s Cohen’s d = 1.4), with which the magnitude of the decline was not different between the two groups. Following the intervention, the pre-IEP SEPT performance did not change significantly from the pre-intervention level in both groups. Moreover, the decline in the post-IEP SEPT performance was not attenuated in either group (RSH: 170.7 ± 38.1, 100.5 ± 33.4, p < 0.05, Cohen’s d = 1.96; CON: 168.1 ± 40.3, 106.1 ± 34.9, Cohen’s d = 1.65).
This study was designed to investigate whether 5-wk RSH in team-sport players would result in enhancements of their global IM and CM functions concomitant with the increase in RSA demonstrated in an endurance-type team-sport specific IEP. The present findings show that despite of marked improvements in the RSA variables in IEP (M-Vel: 6.2 ± 4.4%, M-Po: 15.3 ± 8.4%) were observed immediately following the 5-wk RSH, RSH players’ global IM and CM functions revealed by the pre-IEP PImax and SEPT performance, respectively, did not alter significantly. Nevertheless, PImax which declined markedly in pre-intervention IEP was alleviated significantly in post-intervention IEP, while the concomitant declined SEPT performance in the pre-intervention IEP was retained post intervention. For the CON, all the responses in the variables of RSA (M-Vel: -0.04 ± 1.1%, M-Po: -0.1 ± 2.5%) as well as IM and CM functions were unchanged following the intervention period. Accordingly, the study hypothesis that 5-wk RSH would enhance players’ RSA was accepted, while the part of the enhancement of IM and CM functions was challenging. Nonetheless, the hypothesis associated to the promotion of IM fatigue resistance during the IEP is discussable. In this study, although the net influence of hypoxia on the results of RSH was not examined with the corresponding training in normoxia, the augmentation of players’ RSA in post-intervention IEP are in line with our recent findings of the boost in RSA following identical 5-wk RSH regimen in team-sport athletes (Shi et al., 2023). Such boosting effects on RSA have been found to maintain in players for at least 4 weeks after the intervention (Shi et al., 2023). Apart from those hypoxia-related adaptations and associated augmented perfusion and O2 extraction in leg’s fast-twitch fibres, the favourable residual boosting effects of RSH on RSA was partly ascribed to the possible morphological and neurological adaptations in players’ leg muscles resulting from the treadmill training that increase greater leg strength and fatigue resistance in repeating the sprints (Folland and Williams, 2007; Franks et al., 2012). However, the present study did not find concomitant enhancement in players’ global IM strength after going through the 5-wk RSH. Previous studies have demonstrated that increase in IM strength with specific IM training could improve players’ abilities in performing repeated sprint and high-intensity intermittent bouts of running, with attenuations of the breathing effort sensation and IM metaboreflex during the exercise were the potential underlying mechanisms (Tong et al., 2010; Archiza et al., 2018; Cavalcante et al., 2019). The lack of improvement in global IM strength following the RSH suggests that players’ enhanced RSA could not be attributed to any perceptual or physiological adaptations in relation to the increase in IM strength resulting from the heavy ventilatory demand during the RSH. The contradiction of the present findings to the significant increases in respiratory muscle strength reported previously in untrained individuals after 4-wk high-intensity interval training (5 x 1-min cycling at 90% of peak work rate interspersed with 3-min recovery) could not be explained clearly (Dunham and Harms, 2012; Yalman et al., 2021). It was noted that respiratory muscle adapta-tions to exercise training appear to be dose dependent, i.e. exercise training-induced increases in respiratory muscle function require a significant training volume (Shei, 2018). The hypoxia-induced hyperventilation elicited during the low-volume RSH regimen might have not effectively overloaded players’ IM in the same way as that achieved previously in relative high-volume exercise trainings (Dunham and Harms, 2012; Yalman et al., 2021) and in low-volume specific IM training with pressure-threshold resistance imposed (Tong et al., 2008; Shei, 2018). In post-intervention IEP, despite the RSH players’ M-Po and WTot increased, the resultant impairment in PImax and increases in s-RPB and s-RPE during the exercise did not exacerbate further. In opposite, the decline in the PImax compared to that occurred in pre-intervention trial was markedly attenuated, and there was no further increase in s-RPB and s-RPE. Although players’ ventilatory responses during the IEP have not been measured on site in avoidance of potential disturbances in perceived breathing effort sensation and repeating maximal sprints, large decreases in pulmonary ventilation and associated ventilatory work following RSH were not likely according to the findings in previous RSH studies (Galvin et al., 2013; Giovanna et al., 2022). It is therefore reasonable to postulate that players’ IM endurance might have been improved in the same way as that resulting from respiratory muscle endurance training to counteract exercise-induced diaphragmatic fatigue (Verges et al., 2007). In fact, respiratory muscle endurance appears to vary as a dependent function of exercise training (Powers et al., 1997). It has been reported that a 16% increase in respiratory muscle endurance was detected following long-term running training in untrained individuals (Robinson and Kjeldgaard, 1982). Likewise, significant increase in swimmers’ IM endurance was found after a 12-wk training program (Clanton et al., 1987). In line with these findings, small but significant increases in oxidative and antioxidant enzyme activities in both inspiratory and expiratory muscles, as well as fast-to-slow shift myosin heavy chain isoforms within the costal diaphragm have been found in animals after various intensities of chronic training (Sugiura et al., 1990; Powers et al., 1992). In addition, the increase in citrate synthase activity and alterations in myosin heavy chain isoforms within Type II isoforms in animals’ diaphragm resulting from 9-wk sprint interval training in hypoxia were greater in comparison to that in normoxia (Ogura et al., 2005). Interestingly, the increase in respiratory muscle endurance in runners following 6-wk isocapnic hyperpnoea training under hypoxic conditions (SpO2 set at 90-80%) was found not different from that of their normoxic counterparts when similar ventilatory volume (50-60% 12-s maximal voluntary ventilation for 30 mins) and pattern (tidal volume fixed at 60% vital capacity) were performed by both groups throughout the training period (Katayama et al., 2019). Accordingly, the possible improved IM endurance in players following the 5-wk RSH is likely to be the integrated results of the adaptations in IM mediated by the two different stimulus sources of RST and hypoxia. However, the current data could not identify the net effects of the hypoxia on boosting up the potential RST-induced changes in IM endurance. It has been reported that the increases in maximal glycolytic enzyme activity and Na+-K+-pump capacity in locomotor muscles following 7-wk sprint interval training underlay the augmented maximum power output over four repeated 30-s efforts on a cycle ergometer (MacDougall et al., 1998). Further, the increases in carbonic anhydrase mRNA concentration and lactate dehydrogenase activity in cyclists’ leg muscles after 8 sessions of RSH were greater in comparison with their normoxic counterparts while the change in citrate synthase activity post intervention was not significant in both groups (Faiss et al., 2013). The enhanced buffer capacity together with the unchanged mitochondrial enzyme activity indicates that there would be a shift from aerobic to anaerobic glycolytic activity in locomotor muscles during post-RSH repeated-sprint exercise (Faiss et al., 2013). On the other hand, the additional ventilatory load in animals during sprint interval training in hypoxia resulted in greater reduction in diaphragmatic lactate dehydrogenase activity and increase in citrate synthase activity in comparison to normoxia (Ogura et al., 2005). The increased oxidative capacity of the respiratory muscles is likely to increase the lactate uptake and improve the lactate removal from circulating blood (Bigard et al., 1992). Indeed, increased respiratory endurance in athletes following 4-wk isocapnic hyperpnoea training have been found to reduce whole-body blood lactate during intense endurance exercise (Spengler et al., 1999). Accordingly, the increased ΔLa in consequence of the augmented M-Po and WTot in post-intervention IEP appears to be the net results of the possible RSH-induced physiological adaptations in locomotor and respiratory muscles, respectively, in producing and consuming lactate. It has been shown that the restoration of power output during repeated sprints is more associated with the quantity of muscle phosphocreatine resynthesis than the recovery of muscle pH (Mendez-Villanueva et al., 2012). Hence, the attenuation of IM metaboreflex secondary to the enhancement of IM strength and the resultant reductions in whole-body metabolite accumulations was considered as an important underlying mechanism for the enhancements of team-sport players’ RSA, as well as their tolerance of maximum intense intermittent run, following a specific IM training (Tong et al., 2008, Archiza et al., 2018; Cavalcante et al., 2019). Indeed, the augmented fatigue resistance in respiratory muscles following either specific strength or endurance training has been shown to lead to reduction or delay of the metaboreflex arising from the muscles and, consequently, enhancement of exercise performance (Illi et al., 2012). Whether the attenuation of PImax decline in post-intervention IEP following the 5-wk RSH had contributed to the augmentation of players’ RSA in IEP awaits further investigation. In pre-intervention IEP, the decline in PImax was concomitant with the impairment of SEPT performance in players. Following the 5-wk RSH, both the pre-IEP PImax and SEPT performance did not alter. Although the current findings could not clearly demonstrate the dual role of the IM in breathing and core stability during exercise, related evidence has been shown in following two studies: During intense running to exhaustion, runners’ heavy ventilatory work that induced IM fatigue could independently lead to global CM fatigue (Tong et al., 2014b). In contrast, 4-wk pressure-threshold IM training which markedly improved runners’ PImax could concomitantly result in tiny but significant enhancement in their SEPT performance (Tong et al., 2016). In the present study, the impaired SEPT performance of players during IEP did not gain any benefit from the attenuation of the IEP-induced decline in PImax in post-intervention trial. The revived PImax concomitant with no corresponding improvement in post-IEP SEPT performance could not be clearly explained, and yet these findings suggest that the underlying mechanisms for the revival of the post-IEP PImax in post-intervention trial might not be identical to those for the improved PImax in response to pressure-specific IM training (Tong et al., 2016). It was noted that IM training using voluntary isocapnic hyperpnoea requires individual to perform the targeted ventilation at higher flow rates with which the increase in airways resistance at these higher flows would be minimal (HajGhanbari et al., 2013). Such flow-specific IM training has been shown favourable in improving related muscle endurance capacity, while the increase in IM strength or PImax was trivial (Romer and McConnell, 2003; HajGhanbari et al., 2013). The present data could not reveal whether this scenario also existed during the 5-wk RSH and led to solely the enhancement of players’ IM endurance. Further evidence on related muscle endurance resulting from RSH is required. Regardless, the lack of improvement in pre- and post-IEP SEPT performance in post-RSH trial suggests that the effect of 5-wk RSH on players’ global CM function is negligible. In the present study, here are some limitations that deserve discussion. The lack of corresponding RST in CON might limit the interpretation of the net effect of hypoxia on RSH-resulted counteraction of IEP-induced PImax decline. Nonetheless, the present findings that players’ IM fatigue induced by team-sport specific IEP alleviated following 5-wk RSH, to our best knowledge, are novel and have not been reported. Besides, the present study did not measure players’ ventilatory responses during the 5-wk RSH and IEPs in avoidance of the interference created by ventilatory measurement device in perceptual and sprint performances, related evidence referred for elucidating our findings are postulated. Ventilatory measurement taken place at selected time points rather than throughout the RSH session or IEP in future studies may be helpful to extricate from the dilemma. In this study, the alteration in the IM function following RSH was only revealed by measuring players’ PImax that was relevant to IM strength. The inclusion of measurement of ventilatory endurance may provide further evidence about the potential enhancement in respiratory muscle endurance after the intervention. Lastly, it has been suggested that only a significant volume of exercise training could induce training adaptations in the respiratory muscles as related adaptations to the exercise training are likely to be dose dependent (Shei et al., 2018). Despite long-term hypoxic training may not be practical in real team-sport training settings, to study further whether an RSH regimen longer than 5 weeks would induce marked improvements in respiratory muscle strength and endurance in players may bring further insight into this specific ergogenic aid.
In conclusion, 5-wk RSH markedly improved players’ RSA in IEP while their pre-IEP PImax and SEPT performance did not alter. In pre-intervention IEP, both PImax and SEPT performance declined markedly. In post-intervention IEP, despite of significant increases in M-Po and WTot, corresponding PImax decline attenuated and the s-RPB was unchanged. In contrast, the decline in SEPT performance in pre-intervention IEP was retained in post-intervention trial. Such findings lead to a postulation that players’ IM endurance, rather than strength, was improved with the 5-wk RSH regimen, while the possible improved IM endurance did not advance the fatigue resistance of CM in SEPT. In this study, although the enhancement of players’ RSA in post-intervention trial was found in accompanying with alleviation of IM fatigue, the current findings are not sufficient to assess if the alleviated IM fatigue had played a role in boosting the RSA. To examine the corresponding alterations in the RSH-resulted enhancements in players’ RSA with manipulations of their exercising ventilatory work and associated alleviation of PImax decline may inspire further.
ACKNOWLEDGEMENTS |
This research was funded by Macao Polytechnic University (RP/ESCSD-04/2020). The experiments comply with the current laws of the country in which they were performed. The authors have no conflict of interest to declare. The datasets generated during and/or analyzed during the current study are not publicly available but are available from the corresponding author who was an organizer of the study. |
|
AUTHOR BIOGRAPHY |
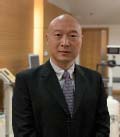 |
Qingde Shi |
Employment: Faculty of Health Sciences and Sports, Macao Polytechnic University |
Degree: PhD |
Research interests: Exercise physiology |
E-mail: qdshi@mpu.edu.mo |
|
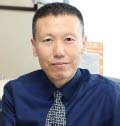 |
Jinlei Nie |
Employment: Faculty of Health Sciences and Sports, Macao Polytechnic University |
Degree: PhD |
Research interests: Exercise physiology, Sports Cardiology |
E-mail: jnie@mpu.edu.mo |
|
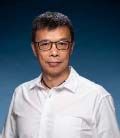 |
Tomas K. Tong |
Employment: Dr. Stephen Hui Research Centre for Physical Recreation and Wellness, Hong Kong Baptist University |
Degree: PhD |
Research interests: Exercise physiology, Athletic training |
E-mail: tongkk@hkbu.edu.hk |
|
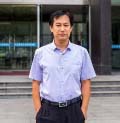 |
Haifeng Zhang |
Employment: Physical Education College, Hebei Normal University |
Degree: PhD |
Research interests: Exercise physiology |
E-mail: zhanghaifeng@hebtu.edu.cn |
|
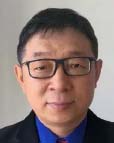 |
Zhaowei Kong |
Employment: Faculty of Education, University of Macau |
Degree: PhD |
Research interests: Exercise physiology |
E-mail: zwkong@um.edu.mo |
|
|
|
REFERENCES |
 Al-bilbeisi F., McCool F.D. (2000) Diaphragm recruitment during nonrespiratory activities. American Journal of Respiratory and Critical Care Medicine 162, 456-459. Crossref
|
 Archiza B., Andaku D.K., Caruso F.C.R., Bonjorno J.C., Oliveira C.R., Ricci P.A., Amaral A.C.D., Mattiello S.M., Libardi C.A., Phillips S.A., Arena R., Borghi-Silva A. (2018) Effects of inspiratory muscle training in professional women football players: a randomized sham-controlled trial. Journal of Sports Sciences 36, 771-780. Crossref
|
 Bigard A. X., Brunet A., Serrurier B., Guezennec C.Y., Monod H. (1992) Effects of endurance training at high altitude on diaphragm muscle properties. Pflugers Archiv : European Journal of Physiology 422, 239-244. Crossref
|
 Billaut F., Smith K. (2009) Sex alters impact of repeated bouts of sprint exercise on neuromuscular activity in trained athletes. Applied Physiology, Nutrition, and Metabolism 34, 689-699. Crossref
|
 Borg G., Ljunggren G., Ceci R. (1985) The increase of perceived exertion, aches and pain in the legs, heart rate and blood lactate during exercise on a bicycle ergometer. European Journal of Applied Physiology and Occupational Physiology 54, 343-349. Crossref
|
 Brocherie F., Girard O., Faiss R., Millet G.P. (2017) Effects of Repeated-Sprint Training in Hypoxia on Sea-Level Performance: A Meta-Analysis. Sports Medicine (Auckland, N.Z.) 47, 1651-1660. Crossref
|
 Cavalcante Silva R.L., Hall E., Maior A.S. (2019) Inspiratory muscle training improves performance of a repeated sprints ability test in professional soccer players. Journal of Bodywork and Movement Therapies 23, 452-455. Crossref
|
 Clanton T.L., Dixon G.F., Drake J., Gadek J.E. (1987) Effects of swim training on lung volumes and inspiratory muscle conditioning. Journal of Applied Physiology 62, 39-46. Crossref
|
 Cohen, J. (1988) Statistical power analysis for the behavioral sciences.
2nd ed. Hillsdale, MI, Lawrence Erlbaum Associates
|
 Constantini K., Bouillet A.C., Wiggins C.C., Martin B.J., Chapman R.F. (2021) Ventilatory Responsiveness during Exercise and Performance Impairment in Acute Hypoxia. Medicine and Science in Sports and Exercise 53, 295-305. Crossref
|
 Dunham C., Harms C.A. (2012) Effects of high-intensity interval training on pulmonary function. European Journal of Applied Physiology 112, 3061-3068. Crossref
|
 Faiss R., Léger B., Vesin J. M., Fournier P. E., Eggel Y., Dériaz O., Millet G.P. (2013) Significant molecular and systemic adaptations after repeated sprint training in hypoxia. PloS One 8, e56522. Crossref
|
 Ferguson C.J. (2009) An effect size primer: A guide for clinicians and researchers. Professional Psychology, Research and Practice 40, 532-538. Crossref
|
 Ferrari Bravo D., Impellizzeri F.M., Rampinini E., Castagna C., Bishop D., Wisloff U. (2008) Sprint vs. interval training in football. International Journal of Sports Medicine 29, 668-674. Crossref
|
 Folland J.P., Williams A.G. (2007) The adaptations to strength training: morphological and neurological contributions to increased strength. Sports Medicine (Auckland, N.Z.) 37, 145-168. Crossref
|
 Franks K.A., Brown L.E., Coburn J.W., Kersey R.D., Bottaro M. (2012) Effects of Motorized vs Non-Motorized Treadmill Training on Hamstring/Quadriceps Strength Ratios. Journal of Sports Science and Medicine 11, 71-76. Pubmed
|
 Galvin H.M., Cooke K., Sumners D.P., Mileva K.N., Bowtell J.L. (2013) Repeated sprint training in normobaric hypoxia. British Journal of Sports Medicine 47, 74-79. Crossref
|
 Gatterer H., Menz V., Untersteiner C., Klarod K., Burtscher M. (2019) Physiological factors associated with declining repeated sprint performance in hypoxia. Journal of Strength and Conditioning Research 33, 211-216. Crossref
|
 Giovanna M., Solsona R., Sanchez A.M.J., Borrani F. (2022) Effects of short-term repeated sprint training in hypoxia or with blood flow restriction on response to exercise. Journal of Physiological Anthropology 41, 32. Crossref
|
 HajGhanbari B., Yamabayashi C., Buna T.R., Coelho J.D., Freedman K.D., Morton T.A., Palmer S.A., Toy M.A., Walsh C., Sheel A.W., Reid W.D. (2013) Effects of respiratory muscle training on performance in athletes: a systematic review with meta-analyses. Journal of Strength and Conditioning Research 27, 1643-1663. Crossref
|
 Illi S.K., Held U., Frank I., Spengler C.M. (2012) Effect of respiratory muscle training on exercise performance in healthy individuals: a systematic review and meta-analysis. Sports Medicine (Auckland, N.Z.) 42, 707-724. Crossref
|
 Katayama K, Goto K, Ohya T, Iwamoto E, Takao K, Kasai N, Sumi D., Mori H., Ishida K., Shimizu K., Shiozawa K., Suzuki Y. (2019) Effects of Respiratory Muscle Endurance Training in Hypoxia on Running Performance. Medicine and Science in Sports and Exercise 51, 1477-1486. Crossref
|
 Kibler W.B., Press J., Sciascia A. (2006) The role of core stability in athletic function. Sports Medicine (Auckland, N.Z.) 36, 189-198. Crossref
|
 Laveneziana P., Albuquerque A., Aliverti A., Babb T., Barreiro E., Dres M., Dubé B.P., Fauroux B., Gea J., Guenette J.A., Hudson A.L., Kabitz H.J., Laghi F., Langer D., Luo Y.M., Neder J A., O'Donnell D., Polkey M.I., Rabinovich R.A., Rossi A., Series F., Similowski T., Spengler C.M., Vogiatzis I., Verges S. (2019) ERS statement on respiratory muscle testing at rest and during exercise. The European Respiratory Journal 53, 1801214. Crossref
|
 MacDougall J.D., Hicks A.L., MacDonald J.R., McKelvie R.S., Green H.J., Smith K.M. (1998) Muscle performance and enzymatic adaptations to sprint interval training. Journal of Applied Physiology 84, 2138-2142. Crossref
|
 McLaren S.J., Smith A., Spears I.R., Weston M. (2017) A detailed quantification of differential ratings of perceived exertion during team-sport training. Journal of Science and Medicine in Sport 20, 290-295. Crossref
|
 Mendez-Villanueva A., Edge J., Suriano R., Hamer P., Bishop D. (2012) The recovery of repeated-sprint exercise is associated with PCr resynthesis, while muscle pH and EMG amplitude remain depressed. Plos one 7, e51977. Crossref
|
 Millet G.P., Girard O., Beard A., Brocherie F. (2019) Repeated sprint training in hypoxia - an innovative method. Deutsche Zeitschrift fur Sportmedizin 70, 115-122. Crossref
|
 Minahan C., Sheehan B., Doutreband R., Kirkwood T., Reeves D., Cross T. (2015) Repeated-sprint cycling does not induce respiratory muscle fatigue in active adults: measurements from the powerbreathe® inspiratory muscle trainer. Journal of Sports Science and Medicine 14, 233-238. Pubmed
|
 Mohr M., Krustrup P., Bangsbo J. (2005) Fatigue in soccer: a brief review. Journal of Sports Sciences 23, 593-599. Crossref
|
 Ogura Y., Naito H., Aoki J., Uchimaru J., Sugiura T., Katamoto S. (2005) Sprint-interval training-induced alterations of Myosin heavy chain isoforms and enzyme activities in rat diaphragm: effect of normobaric hypoxia. The Japanese Journal of Physiology 55, 309-316. Crossref
|
 Powers S. K., Coombes J., Demirel H. (1997) Exercise training-induced changes in respiratory muscles. Sports Medicine 24, 120-131. Crossref
|
 Powers S. K., Grinton S., Lawler J., Criswell D., Dodd S. (1992) High intensity exercise training-induced metabolic alterations in respiratory muscles. Respiration physiology 89, 169-177. Crossref
|
 Robinson E.P., Kjeldgaard J.M. (1982) Improvement in ventilatory muscle function with running. Journal of Applied Physiology: Respiratory, Environmental and Exercise Physiology 52, 1400-1406. Crossref
|
 Romer L.M., McConnell A.K. (2003) Specificity and reversibility of inspiratory muscle training. Medicine and Science in Sports and Exercise 35, 237-244. Crossref
|
 Shei R.J. (2018) Recent Advancements in Our Understanding of the Ergogenic Effect of Respiratory Muscle Training in Healthy Humans: A Systematic Review. Journal of Strength and Conditioning Research 32, 2665-2676. Crossref
|
 Shi Q., Tong T.K., Nie J., Tao D., Zhang H., Tan X., Kong Z. (2023) Repeated-sprint training in hypoxia boosts up team-sport-specific repeated-sprint ability: 2-week vs 5-week training regimen. European Journal of Applied Physiology 123, 2699-2710. Crossref
|
 Spengler C.M., Roos M., Laube S.M., Boutellier U. (1999) Decreased exercise blood lactate concentrations after respiratory endurance training in humans. European Journal of Applied Physiology and Occupational Physiology 79, 299-305. Crossref
|
 Sugiura T., Morimoto A., Sakata Y., Watanabe T., Murakami N. (1990) Myosin heavy chain isoform changes in rat diaphragm are induced by endurance training. The Japanese Journal of Physiology 40, 759-763. Crossref
|
 Tong T.K., Fu F.H., Chow B.C., Quach B., Lu K. (2003) Increased sensations of intensity of breathlessness impairs maintenance of intense intermittent exercise. European Journal of Applied Physiology 88, 370-379. Crossref
|
 Tong T.K., Fu F.H., Chung P.K., Eston R., Lu K., Quach B., Nie J., So R. (2008) The effect of inspiratory muscle training on high-intensity, intermittent running performance to exhaustion. Applied Physiology, Nutrition, and Metabolism 33, 671-681. Crossref
|
 Tong T.K., Fu F.H., Eston R., Chung P.K., Quach B., Lu K. (2010) Chronic and acute inspiratory muscle loading augment the effect of a 6-week interval program on tolerance of high-intensity intermittent bouts of running. Journal of Strength and Conditioning Research 24, 3041-3048. Crossref
|
 Tong T.K., Fu F.H., Quach B., Lu K. (2004) Reduced sensations of intensity of breathlessness enhances maintenance of intense intermittent exercise. European Journal of Applied Physiology 92, 275-284. Crossref
|
 Tong T.K., McConnell A.K., Lin H., Nie J., Zhang H., Wang J. (2016) "Functional" inspiratory and core muscle training enhances running performance and economy. Journal of Strength and Conditioning Research 30, 2942-2951. Crossref
|
 Tong T.K., Tao E.D., Chow B.C., Baker J.S., Jiao J.J. (2021) Acute performance responses to repeated treadmill sprints in hypoxia with varying inspired oxygen fractions, exercise-to-recovery ratios and recovery modalities. European Journal of Applied Physiology 121, 1933-1942. Crossref
|
 Tong T.K., Wu S., Nie J. (2014a) Sport-specific endurance plank test for evaluation of global core muscle function. Physical Therapy in Sport 15, 58-63. Crossref
|
 Tong T.K., Wu S., Nie J., Baker J.S., Lin H. (2014b) The occurrence of core muscle fatigue during high-intensity running exercise and its limitation to performance: the role of respiratory work. Journal of Sports Science and Medicine 13, 244-251. Pubmed
|
 Turner A.N., Stewart P.F. (2013) Repeat sprint ability. Strength and Conditioning Journal 35, 37-41. Crossref
|
 Verges S., Lenherr O., Haner A.C., Schulz C., Spengler C.M. (2007) Increased fatigue resistance of respiratory muscles during exercise after respiratory muscle endurance training. American Journal of Physiology. Regulatory, Integrative and Comparative Physiology 292, 1246-1253. Crossref
|
 Vogiatzis I., Georgiadou O., Koskolou M., Athanasopoulos D., Kostikas K., Golemati S. (2007) Effects of hypoxia on diaphragmatic fatigue in highly trained athletes. The Journal of Physiology 581, 299-308. Crossref
|
 Yalman A., Telli Atalay O., Ünver F., Şenol H., Taşkin H. (2021) The sub-acute effects of high-intensity interval training in healthy young adults: respiratory parameters, aerobic capacity and perceived stress. The Journal of Sports Medicine and Physical Fitness 61, 617-624. Crossref
|
 Zois J., Bishop D., Fairweather I., Ball K., Aughey R.J. (2013) High-intensity re-warm-ups enhance soccer performance. International Journal of Sports Medicine 34, 800-805. Crossref
|
|
|
|
|
|
|