|
|
|
ABSTRACT |
Thermoregulation is critical for athletes, particularly those for those who must perform in the heat. Most strategies aimed at reducing heat stress have cooled participants before or during activity. The objective of this study is to investigate whether seven minutes of head cooling applied between bouts of aerobic exercise in hot (35 ± 1.0 °C) and dry (14.68 ±4.29% rh) environmental conditions could positively effect participants peak power output (PP) on a maximal effort graded exercise test (GXT). Twenty-two recreational active men ages 18 to 23 (19.8 ± 1.6 yrs.) completed three performance trials over a 21 day period. During the first trial, participants were familiarized with procedures and completed a maximal effort GXT on a cycle ergometer to establish maximal baseline performances. The second and third trials, which were counterbalanced, consisted of a cooling and placebo condition. During both of these trials, participants cycled 40 minutes at 65% of their maximum VO2, in hot (35 ± 1.0 °C) and dry (17-20% rh) environmental conditions. Immediately after this initial bout of activity, participants were given seven minutes of recovery in which head cooling was applied during the cooling condition and withheld during the placebo condition. Participants then completed a maximal effort GXT. Significant differences (p < 0.001) in participants peak power output (W) were measured when cooling was applied compared to the placebo condition (304.23(W) ± 26.19(W) cooling, 291.68(W) ± 26.04(W) placebo). These results suggest that a relatively brief period of intermittent cooling may enhance subsequent aerobic performance. |
Key words:
Thermoregulation, thermal-loading, heat-stress, hot conditions, endurance
|
Key
Points
- Thermoregulation is a critical performance variable
- Pre-cooling and Mid-cooling methods have been shown to benefit aerobic and anaerobic performance
- To date, intermittent head mid-cooling has not been investigated
- This study demonstrated that seven minutes of intermittent head cooling was sufficient to positively effect aerobic performance
|
The physiological demands of thermoregulation during intense physical activity are substantial, particularly for athletes performing in hyperthermic conditions. Several studies have reported diminished physical performance in environmental conditions greater than 32°C (Howley et al., 1995; Midgley et al., 2007; Tyler et al., 2015). Humans seem to be especially susceptible to heat in that they can generally tolerate twice the decline in core temperature (10°C) as they can in its increase (5°C) (McArdle et al., 2010). In an effort to ameliorate the effects of thermal stress, various cooling methods have been employed. To date, approximately 100 original investigations and 13 reviews have examined the effectiveness of cooling on motor performance (Stevens et al., 2016). The results of these studies indicate that external cooling methods, in general, have a positive effect on performance (Ruddock et al., 2016; Stevens et al., 2016; Tyler et al., 2015). As cooling research emerged a more nuanced understanding of when cooling can be applied has developed. Most early investigations administered cooling prior to physical activity, commonly referred to as pre-cooling. A strong majority of these studies demonstrated that pre-cooling had a positive effect on subsequent motor performance (Stevens et al. 2016; Tyler et al. 2015). More recently, investigators have examined the effect of cooling during performance, referred to as mid-cooling. As with pre-cooling, positive results were largely reported with some investigators suggesting that the benefits gained from mid-cooling outweigh that of pre-cooling (Tyler et al. 2015; Stevens et al. 2016). Mid-cooling can further be subdivided into continuous and intermittent cooling. Continuous mid-cooling is when cooling is applied throughout activity. This is often accomplished by having participants don cooling equipment such as a vest, collar, hand pads or the administration of spray/fanning (Tyler and Sunderland 2011; Minniti et al. 2011; Amorim et al. 2010; Kenny et al. 2011). Intermittent mid-cooling is when cooling is applied during a motor task periodically rather than continuously. Such as ingesting a cold drink or ice slurry every 15 minutes while cycling (Mundel et al. 2006; Siegel et al. 2010). Both continuous and intermittent mid-cooling have resulted in positive effects to heat stressed participants ((González-Alonso et al. 1999; Ruddock et al. 2016; Stevens et al. 2016). The purpose of this study is to investigate whether seven minutes of head cooling applied in between a bout of aerobic exercise in hot (35 ±1.0 degree °C) and dry (15 ±3% rh) conditions could benefit participant’s aerobic performance. The scope of this study was selected for four reasons. First, intermittent cooling is the least examined form of cooling research. In a recent review, approximately 80% of cooling studies examined pre-cooling will 20% investigated mid-cooling (Stevens et al. 2016). In addition, the majority of mid-cooling studies have utilized continuous cooling strategies as compared to intermittent (Ruddock et al. 2016; Stevens et al. 2016). Second, the application of intermittent cooling to many popular sports such as cricket, field hockey, tennis, volleyball, baseball, golf, basketball, and football is compelling. Each of these events have naturally occurring phases of inactivity in which cooling could be applied. Third, intermittent cooling allows for the application of cooling at strategic times when the outcome of competitive events is decided. Finally, investigators in the most review of mid-cooling strategies recommended that head cooling be examined (Stevens et al., 2016). Some of the rational for head-cooling includes: ease of access, high degree of blood flow to this region, and the ability to cool without reducing working muscle temperature (Palmer et al., 2001). Currently, no studies exist examining this method of head cooling on performance.
Experimental approach to the problemA randomized cross-over study design was selected to determine whether seven minutes of head cooling between bouts of activity could attenuate the decline in performance that is often associated with heat stressed performances. This design was selected in order to minimize the effects of between participants physiological variability. Each subject was required to complete three sessions. Session one consisted of acclimatizing participants to testing procedures and obtaining baseline measures. The second and third sessions were counterbalanced and consisted of treatment and placebo (See Table 1). The independent variable in this investigation was head cooling via the Welkins Sideline Cooling System (Downers Grove, IL). This device consists of three major components: a cooling cartridge, heat exchange, and tethered neoprene cap (see Figure 1). The heat exchange circulates 9oz of cooling fluid through the tubing and neoprene cap which snuggly fits on the subject’s head. During maximal cooling, the circulating fluid maintains a temperature between 5-10°C. During treatment conditions, maximal cooling from this device was applied. Power to this device is supplied via internal batteries. In order to create a placebo condition, participants were informed that the focus of this investigation was to compare the effects of two modes of cooling on aerobic performance: traditional and innovative. The traditional method of cooling was explained as cooling the head via circulating fluid, in which participants would recognize the sensation of cooling via the head gear. The innovative method of cooling (placebo) was explained as using MRI technology to target thermoreceptors within the brain for deep brain cooling. Participants were told that although inner cooling of the brain was occurring, no noticeable cooling via sensation could be recognized. These explanations were given to minimize the effect of the sensory reinforcement. The dependent variable was peak power output. A graded exercise test (GXT) was used to measure performance indicators.
ParticipantsTwenty-two recreationally active men between the ages of 18 and 23 years participated in the study (19.8 ± 1.6 yrs.). The average mass and height of the participants were 1.82 ± 0.08 m. and 78.4 ± 15.6 kg. All participants reported participating in at least 90 minutes of moderate to vigorous physical activity per week within the previous six months. Each participant agreed not to alter their typical activity and dietary habits throughout the duration of this study. Participants reported no cardiovascular, neural, or musculoskeletal disease or injuries that would prevent them from completing the exercise bouts. After reviewing research procedures, protocol, and potential risks, participants were given an opportunity to ask questions before reading and signing an informed consent form, which received approval from the Institutional Review Board (IRB). Each participant that complied with al established procedures and guidelines was financially compensated.
Test proceduresThe first session was conducted under hyperthermic, low humidity environmental conditions (35 ±1.0 °C, 15% ±3% rh). Participants’ peak oxygen consumption and peak aerobic power output were determined using a ramp protocol on a Velotron electronically-braked cycle ergometer (Racermate, Seattle, WA. Participants biked for three minutes at a constant power output of 100 watts. After this warm-up period, power increased at a rate of one watt per 2.5 seconds. Participants were asked to continue cycling until volitional exhaustion. Oxygen Consumption (VO2) and respiratory exchange ratio (RER) were measured continuously using open-circuit spirometry (TrueOne 2400 metabolic cart, Parvo Medics, Sandy, UT). Heart Rate (HR) (FT1-Polar, Kempele, Finland) and rectal temperature (RT) (RT-YSI 400 thermistor and 43TA tele-thermometer, YSI, Yellow Springs, OH) were also measured continuously. Values were averaged over 5-second epochs. At the point of volitional exhaustion, VO2 peak, peak power output, HR, RPE (Borg, 1998), rectal temperature, blood lactate (Lactate Plus, Nova Biomedical, Waltham, MA), and peak watts were recorded. A GXT was deemed successful if participants met a minimum of two of four requirements: RPE above 18, HR within 10% of age-predicted maximum (Karvonen Formula), RER > 1.10, and a blood lactate concentration > 7 mm (Howley et al., 1995; Midgley et al., 2007). Participants were encouraged to come to the lab well hydrated, as no fluids were given until after the session was complete. The second and third trials were conducted in a hyperthermic, low humidity environment (35 ±1.0 °C, 15% ±3% rh) and counterbalanced so that half of the participants performed the cooling condition first while the the other half performed the placebo condition first. Procedures for these trials were identical with one exception: the cooling cap was turned on at full power during the cooling trial and turned off during the placebo trial. Both sessions began with participants biking for 40 minutes at 65% of maximal oxygen consumption. During this time period, HR, RER, RPE, core temperature, and watts were recorded every five minutes. As these measures were evaluated, power output was adjusted to maintain a workload of 65% of maximal oxygen consumption. After 40 minutes, the participants stopped pedaling and were given a seven-minute recovery period. During this rest interval, the participants’ heads were sprayed with water per manufacturer’s instructions to allow for enhanced thermal transference and the cooling cap was firmly fitted to their heads. All participants remained on the bike for the rest interval. At the cessation of rest interval, the cooling cap was removed and the graded exercise test began immediately. The GXT was identical to each subject’s first trail, except for the omission of a three-minute warm up. After participants reached a point of exhaustion, VO2 peak, peak power output, HR, RPE, rectal temperature and blood lactate were recorded. Participants were required to complete three sessions with a week between each session. Each subject’s session was performed on the same weekday and at the same time of day so that diurnal fluctuations in core temperature could be minimized. In addition, each subject was required to maintain activity and dietary pattern for the duration of data collection. After completing all test trials, participants were given full disclosure as to the treatment and placebo conditions. Gross efficiency was calculated as the ratio of peak work rate to energy expended. Watts were converted to kcal/min (0.004184 kcal/kJ) and VO2 was converted to kcal/min (5.047kcal/LO2) (Coyle et al. 1992).
Statistical analysesTo determine if seven minutes of intermittent head cooling could blunt the decline in peak aerobic power output, change scores were calculated for all performance metrics (cooling/placebo session – baseline) and compared using t-tests for paired data (SPSS v.21, IBM). The decline in VO2 peak and peak power output relative to the baseline GXT were compared between the head cooling and placebo treatment. Statistical differences between means were established at a minimum level of p ≤ 0.05.
Chamber temperatures were not different between any of the testing sessions (Baseline: 35.0 ± 0.1°C; Placebo: 35.0 ± 0.3°C; 35.0 ± 0.1°C). Relative Humidity was maintained at a low level for all trials (Baseline: 12.82 ± 4.79% rh; Placebo: 17.45 ± 3.88% rh; Intermittent Cool: 18.91 ± 2.72% rh). The thermal load induced by exercising in the heat was not different between conditions as shown in Figure 2. Subject’s effort on both placebo and cooling trials appeared to be maximal since there where no differences in participants’ peak core temperature, peak HR, blood lactate, and RPE. (see Table 2). As depicted in Figure 3, the subjects’ peak power output was lower during both placebo (291 ± 26.04 Watts) and cooling (304 ± 26.19) compared to their baseline GXT (316.72 ± 33.65 Watts). Though head cooling significantly blunted the decline in power output (p = 0.002). Based on the rate at which power increased during the GXT (1 watt every 2.5 seconds), this difference in peak aerobic power output equated to a difference of 31.4 ± 9.1 seconds. Though power output declined, the decline in VO2peak was not different between placebo and cooling (Figure 3). When gross efficiency was calculated from Watts and VO2, there was no difference between placebo (21.16,0.45%) and cooling (21.78 ± 0.58%) conditions (p = 0.18).
These findings suggest that intermittent cooling is an effective means of increasing aerobic performance but not capacity. These results are consistent with the previous research when pre-cooling or continuous cooling has been applied (Ruddock et al., 2016; Stevens et al., 2016; Tyler et al., 2015). While further evidence concerning the usefulness of cooling is affirming, there are noteworthy aspects of this investigation that can be useful especially in an applied setting. First, intermittent head cooling ameliorates some of the criticisms directed toward pre-cooling or continuous cooling. One criticism specific to pre-cooling is that this method works in opposition to warm-up procedures. The studies that have been conducted on the effect of heat on subsequent performance validate the assumption that warm-up procedures tend to increase the rate and force of muscular contractions, lower the viscous resistance in muscles and joints, and increase blood flow to active muscles (Bergh and Ekblom, 1979; Fradkin et al., 2010). Given the fact that warm-up behaviors are almost universally practiced, a concern exists that pre-cooling may work in opposition to that practice. Another criticism is that cooling to specific regions of the body can result in the diminished rate and force of muscular contractions in the regions cooled (Bergh and Ekblom, 1979). Finally, during continuous cooling, individuals must often support cooling devices throughout their motor performance. While advancements have been made regarding methods for supporting cooling devices, the concern remains that the additional resistance, restricted movement and additional drag from donning cooling equipment may offset any gain from the cooling (Ansley et al., 2008; Stevens et al., 2016; Tyler et al.,2015). These criticisms are largely alleviated through the use of intermittent cooling-specifically of the head. If cooling is applied after an individual’s initial phase of activity, then confounding effects from pre-cooling in close proximity with warming up activities can be avoided. In addition, cooling the head as opposed to anatomical sites located on or near large groups of muscular density can potentially reduce central factors of fatigue, while not adversely affecting localized muscular temperature (Fradkin et al., 2010). Furthermore, intermittent cooling can be applied during the cessation of activity, inherent in many sporting activities, thus eliminating the need to dawn cooling equipment throughout activity as in the case of continuous cooling. While intermittent cooling reduces some of the criticisms associated with pre-cooling or continuous cooling, this particular investigation has limitations that need to be addressed in subsequent research. First, the participants in this study were exclusively male. Females were excluded from in this initial investigation because of the potential confounding effects of menstrual fluctuations in temperature and the thermal barrier associated with additional cranial hair (Marsh and Jenkins, 2012). In addition, subjects were recreationally active as opposed to elite athletes. It is likely that performances differences would likely be reduced as participant’s ability increases. Furthermore, while no differences in core temperature were observed it would have been useful to observe if subject’s brain temperature varied between the treatment and placebo condition. During preliminary trials tympanic temperature was gathered, however due to this devices marginal ability to reliable measure brain temperature it was not used in this study. This finding is supported in the literature (Ganio et al., 2009). Other forms of obtaining brain temperature has much higher degrees of validity and reliability such as microwave radiometry, magnetic resonance thermometry, and ultrasound thermometry, however such instruments were unavailable in the existing laboratory (Corbett et al., 1997; Maruyma et al., 2000). Finally, while a placebo was employed in this study, the strength of the placebo did not have all of the accompanying sensory feedback as did the experimental trial. Although the results of this study support the hypothesis that cooling can positively effect motor performance, the physiological mechanisms that produce this effect are not clearly understood. The greater aerobic power output, coupled with similar VO2peak in our participants suggest that exercise efficiency was improved after intermittent cooling. Mechanical efficiency was not measured, though it is unlikely that heating or cooling would significantly effect cycling biomechanics. Thus, an improvement in metabolic efficiency would have to be hypothesized. While studies have shown that increased muscle temperature is associated with increased metabolic efficiency (Olschewski and Bruck, 1988; Marino et al., 2004), our data indicate that rectal temperature was not altered by intermittent cooling of the head. Therefore, it is unlikely that short-term, intermittent cooling of the head impacted metabolic efficiency. Another possibility is that our bout of active heating (40 minutes of cycling at 65% of VO2peak) produced some degree of central fatigue (Hettinga et al., 2007), and that even brief intermittent cooling of the head reduce central fatigue, allowing for greater power output. Future studies will be needed to clarify the peripheral and central mechanisms that intermittent cooling directly or indirectly effects.
ApplicationIn addition to removing many of the limitations associated with administering pre-cooling and continuous cooling, intermittent cooling can be administered to a wide range of athletes who participate in sports with frequent periods of activity cessation. During a typical National Football League game, the ball is in play for only 11 minutes out of approximately 2.5 hours of game time (Biderman 2010). The average ice time for a top ranked player in the National Hockey League is approximately 19 minutes during a 60 minute interval (NHL Website, 2014). The average amount of time the ball is in play during a major league baseball game that typically lasts three hours is 18 minutes (Moyer, 2013). Thus, in many sports, there is ample opportunity for athletes to apply intermittent cooling in order to optimize performance. Due to the ease of application to the head region and the relatively short period of time needed for cooling to be applied, intermittent cooling is well suited for dynamic environmental conditions, as when pronounced temperature fluctuations occur within a sporting event or due to travel. In 2007, the Chicago Marathon began in the low 70’s, some 3.5 hours later the event was canceled due to temperatures reaching into the 90’s (Snyder, 2007). In one study, Soccer players’ accustomed to performing in relatively cool temperatures (12°C) experienced a 6% decline in anaerobic power output while traveling to a match where moderate thermal stress (30°C) was encountered (Mohr and Krustrup, 2013). Finally, the degree to which participants performances were enhanced as a result of intermittent cooling was striking. Placebo and trial conditions lasted between 7-9 minutes. Power increased during the GXT at a rate of 1 watt every 2.5 seconds. On average, there was a 31 second improvement in time to exhaustion when participants received intermittent cooling as compared to when they did not (cooling 510 sec., placebo 479 sec.). While a direct comparison between aerobic performances and a cycling GXT can be made, athletic events such as the Tour de France prologue, 2 mile run, and the 800 meter swim are aerobic activities have approximately the same duration (7-9 minutes). In the 2012 Tour de France prologue, 31 seconds was the difference between 1st Place and 164th place (VeloNews, 2014). According to the Men’s All-time Best 2 mile run times, there are over 300 individuals between the first Place time and 31 seconds later (Larsson, 2016). In the 800m men’s swim at the 2015 World Aquatics Championship, 31 seconds was the difference between first and 37th place (FINA, 2015). While time to exhaustion and performance times are not parallel and recreational participants should not be confused with elite athletes, results from this study does indicate that intermittent head cooling is associated with improved aerobic performance. These findings, which are consistent with prior research, provide trainers, coaches, and practioners with another tool for blunting the negative effects of thermal stress.
These results suggest that a relatively brief period of intermittent head cooling may enhance subsequent aerobic performance for individuals performing in hot (35 ± 1.0 °C) environmental conditions. The application of these findings holds promise to a wide range of athletes who participate in sports with frequent periods of activity cessation.
ACKNOWLEDGEMENTS |
The research team would like to thank WElkins for partially funding this project and their strong commitment in allowing scientific inquiry to determine efficacy. The authors declare no conflicts of interest existed in this study. |
|
AUTHOR BIOGRAPHY |
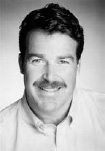 |
Peter Walters |
Employment: Professor in the Applied Health Science Department at Wheaton College. |
Degree: PhD |
Research interests: Strength training, sleep, and thermoregulation. |
E-mail: Peter.Walters@Wheaton.edu |
|
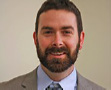 |
Nathaniel Thom |
Employment: Ass. Prof., Biology Science Center, Wheaton College. |
Degree: PhD |
Research interests: Promoting brain health via non-pharmacological interventions such as exercise, nutrition, and meditation. |
E-mail: nate.thom@wheaton.edu |
|
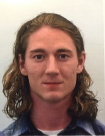 |
Kai Libby |
Employment: A graduate student at the University of Edinburgh. |
Degree: BSc |
Research interests: Management of bioeconomy, innovation and governance. |
E-mail: mylaxacademy@gmail.com |
|
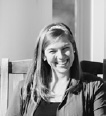 |
Shelby Edgren |
Employment: Rush University |
Degree: BSc |
Research interests: Maternal child nursing and breastfeeding education. |
E-mail: Shelbyedgren@gmail.com |
|
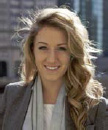 |
Amanda Azadian |
Employment: |
Degree: BSc |
Research interests: Currently runs the events at a community of healthcare entrepreneurs, researchers, and industry leaders who are seeking to build heroic businesses that change healthcare provision through technology innovation. |
E-mail: amanda.azadian@gmail.com |
|
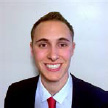 |
Daniel Tannous |
Employment: A first year student at Loma Linda University School of Medicine. |
Degree: BSc |
Research interests: Sleep, diabetes, and obesity prevention in adolescent populations. |
E-mail: danielmtannous@gmail.com |
|
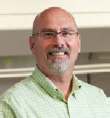 |
Brian Hunt |
Employment: Assoc. Prof. in the Department of Applied Health Science at Wheaton College. |
Degree: PhD |
Research interests: Cardiovascular physiology with specific interests in nutrition and vascular function. |
E-mail: brian.hunt@wheaton.edu |
|
|
|
REFERENCES |
 Amorim F.T., Yamada P.M., Robergs R.A., Schneider S.M. (2010) Palm cooling does not reduce heat strain during exercise in a hot, dry environment. Applied Physiology, Nutrition & Metabolism 35, 480-489.
|
 Ansley L., Marvin G., Sharma A., Kendall M.J., Jones D.A., Bridge M.W. (2008) The Effects of Head Cooling on Endurance and Neuroendocrine Responses to Exercise in Warm Conditions. Physiological Research 57, 863-872.
|
 Bergh H., Ekblom B. (1979) Influence of muscle temperature on maximal muscle strength and power output in human skeletal muscles. Acta Physiologica Scandinavica 107, 33-37.
|
 Biderman D (2010) Wall Street Journal. http://online.wsj.com/news/articles/SB10001424052748704281204575002852055561406
|
 Borg G (1998) Borg’s Perceived Exertion and Pain Scales. Champaign, IL. Human Kinetics.
|
 Corbett R., Laptook A., Weatherall P. (1997) Noninvasive measurements of human brain temperature using volume-localized proton magnetic resonance spectroscopy. Journal of Cerebral Blood Flow & Metabolism 17, 363-369.
|
 Coyle E.F., Sidossis L.S., Horowitz J.F., Beltz J.D. (1992) Cycling efficiency is related to the percentage of type I muscle fibers. Medicine and Science in Sports and Exercise 24, 782-788.
|
 FINA (2015) 16th FINA World Championship: 800 M Swim. http://omegatiming.com/File/Download?id=00010F020000000400FFFFFFFFFFFF02
|
 Fradkin A., Zazryn T., Smoliga J. (2010) Effects of warming-up on physical performance: a systematic review with meta-analysis. Journal of Strength & Conditioning Research 24, 140-148.
|
 Ganio M.S., Brown C.M., Casa D.J., Becker S.M., Yeargin S.W., McDermott B.P., Boots L.M., Boyd P.W., Armstrong L.E., Maresh C.M. (2009) Validity and Reliability of Devices That Assess Body Temperature During Indoor Exercise in the Heat. Journal of Athletic Training 44, 124-135.
|
 González-Alonso J., Teller C., Andersen S.L., Jensen F.B., Hyldig T., Nielsen B. (1999) Influence of body temperature on the development of fatigue during prolonged exercise in the heat. Journal of Applied Physiology 86, 1032-1039.
|
 Hettinga F.J., De Koning J.J., de Vrijer A., Wust R.C.I., Daanen H.A.M., Foster C. (2007) The effect of ambient temperature on gross-efficiency in cycling. European Journal of Applied Physiology 101, 465-471.
|
 Howley E.T., Bassett D.R., Welch H.G. (1995) Criteria for maximal oxygen uptake: review and commentary. Medicine & Science in Sports & Exercise 27, 1292-1301.
|
 Kenny G.P., Schissler A.R., Stapleton J., Piamonte M., Binder K., Lynn A., Lan C.Q., Hardcastle S.G. (2011) Ice Cooling Vest on Tolerance for Exercise under Uncompensable Heat Stress. Journal of Occupational and Environmental Hygiene 8, 484-491.
|
 Larsson P. (2016) Men’s 2 Miles. http://www.alltime-athletics.com/m_2miok.htm
|
 Marino F.E., Lambert M.I., Noakes T.D. (2004) Superior performance of African runners in warm humid but not in cool environmental conditions. Journal of Applied Physiology 96, 124-130.
|
 Marsh S.A., Jenkins D.G. (2012) Physiological Responses to the Menstrual Cycle. Sports Medicine 32, 601-614.
|
 Maruyma K., Mizushina S., Sugiura T., Van Leeuwen G.M.J., Hand J.W., Marrocco G., Bardati F., Edwards A.D., Azzopardi D., Land D. (2000) Feasibility of noninvasive measurement of deep brain temperature in newborn infants by multifrequency microwave radiometry. IEEE Transactions on Microwave Theory and Techniques 48, 2141-2147.
|
 McArdle W.D., Katch F.I., Katch V. (2010) Exercise Physiology. Baltimore, ML. Williams & Wilkins.
|
 Midgley A.W., McNaughton L.R., Polman R., Marchant D. (2007) Criteria for Determination of Maximal Oxygen Uptake: A Brief Critique and Recommendations for Future Research. Sports Medicine 37, 1019-1028.
|
 Minniti A., Tyler C.J., Sunderland C. (2011) Effects of a cooling collar on affect, ratings of perceived exertion, and running performance in the heat. European Journal of Sport Science 11, 419-429.
|
 Mohr M., Krustrup P. (2013) Heat stress impairs repeated jump ability after competitive elite soccer games. Journal of Strength & Conditioning Research 27, 683-689.
|
 Moyer S (2013) In America’s Pastime, Baseball Players Pass A Lot of Time. http://on.wsj.com/1PM8Mbk
|
 Mundel T., King J., Collacott E., Jones D.A. (2006) Drink temperature influences fluid intake and endurance capacity in men during exercise in a hot, dry environment. Experimental Physiology 91, 925-933.
|
 NHL Website (2014) NHL Player Stats. http://www.nhl.com/ice/playerstats.htm?fetchKey=20142ALLSASAll&viewName=summary&sort=points&pg=4
|
 Olschewski H., Bruck K. (1988) Thermoregulatory, cardiovascular, and muscular factors related to exercise after precooling. Journal of Applied Physiology 64, 803-811.
|
 Palmer C.D., Sleivert G.G., Cotter J.D. (2001) Proceedings of Australian Physiological and Pharmacological Society. http://aups.org.au/Proceedings/32(2)Suppl.1/122P/122P.pdf
|
 Ruddock A., Robbins B., Tew G., Bourke L., Purvis A. (2016) Practical Cooling Strategies During Continuous Exercise in Hot Environments: A Systematic Review and Meta-Analysis. Sports Medicine , 1-16.
|
 Siegel R., Maté J., Brearley M.B., Watson G., Nosaka K., Laursen P.B. (2010) Ice slurry ingestion increases core temperature capacity and running time in the heat. Medicine and Science in Sports and Exercise 42, 717-725.
|
 Snyder J (2007) When a Marathon Goes Wrong. http://content.time.com/time/nation/article/
|
 Stevens C.J., Taylor L., Dascombe B.J. (2016) Cooling During Exercise: An Overlooked Strategy for Enhancing Endurance Performance in the Heat. Sports Medicine 1-13, -.
|
 Tyler C.J., Sunderland C. (2011) Neck Cooling and Running Performance in the Heat: Single versus Repeated Application. Medicine and Science in Sports and Exercise 43, 2388-2395.
|
 Tyler C.J., Sunderland C., Cheung S.S. (2015) The effect of cooling prior to and during exercise on exercise performance and capacity in the heat: a meta-analysis. British Journal of Sports Medicine 49, 7-13.
|
 VeloNews (2014) Tour de France 2012 prologue results. VeloNews.com
|
|
|
|
|
|
|