|
|
|
ABSTRACT |
Recently, a novel type of high-intensity interval training known as sprint interval training has demonstrated increases in aerobic and anaerobic performance with very low time commitment. However, this type of training program is unpractical for general populations. The present study compared the impact of a low-volume high-intensity interval training to a "all-out" sprint interval training. Twenty-four active young males were recruited and randomized into three groups: (G1: 3-5 cycling bouts ËŸ 30-s all-out with 4 min recovery; G2: 6- 10 cycling bouts ËŸ 125% Pmax with 2 min recovery) and a non-trained control group. They all performed a VO2max test, a time to exhaustion at Pmax (Tmax) and a Wingate test before and after the intervention. Capillary blood lactate was taken at rest, 3, and 20 min after the Wingate trial. Training was performed 3 sessions per week for 4 weeks. In G1, significant improvements (p < 0.05) following training were found in VO2max (9.6%), power at VO2max (12.8%), Tmax (48.4%), peak power output (10.3%) and mean power output (17.1%). In G2, significant improvements following training were found in VO2max (9.7%), power at VO2max (16.1%), Tmax (54.2%), peak power output (7.4%; p < 0.05), but mean power output did not change significantly. Blood lactate recovery (20th min) significantly decreased in G1 and G2 when compared with pre-testing and the CON group (p < 0.05). In conclusion, the results of the current study agree with earlier work demonstrating the effectiveness of 30-s all-out training program to aerobic and anaerobic adaptations. Of substantial interest is that the low volume high intensity training provides similar results but involves only half the intensity with double the repetitions. |
Key words:
Wingate test, repeated sprints, blood lactate, training adaptations.
|
Key
Points
- Given the markedly lower training volume in the training groups, our results suggest that intense interval training is indeed a time-efficient strategy to induce rapid metabolic and performance adaptations.
- The results demonstrate that a practical low-volume HIT program is effective for improving metabolic and performance adaptations that resemble many of the same performance gains occurred in all-out SIT protocol.
|
It is well established that factors such as poor cardiorespiratory fitness, adiposity, impaired glucose tolerance, hypertension, and arteriosclerosis are independent threats to health and that physical inactivity increases the risk for premature death and elevates the incidence of the abovementioned unhealthy conditions. Epidemiologic cross-sectional investigations and longitudinal intervention studies have provided experimental evidence for the effectiveness of prolonged aerobic exercise training such as continuous running, brisk walking, or bicycling as interventions that may lower the relative risk for developing several metabolic diseases (Nybo et al., 2010). However, lack of time is a common reason why many people fail to accomplish the training programs (Godin et al., 1994). Recently, a novel type of high-intensity interval training (HIT) known as sprint interval training (SIT; repeated 30-s “all-out” efforts) has demonstrated increases in aerobic performance in a short time frame (Burgomaster et al., 2005; Gibala et al., 2006). It is interesting that SIT may induce similar improvements in cardiorespiratory fitness and skeletal muscle oxidative capacity as prolonged training, (Burgomaster et al., 2008; Nybo et al., 2010) and a recent study reported that SIT substantially improves insulin action (Babraj et al., 2009). Apparently, the repeated SIT bouts stress many of the physiological/biochemical systems used in aerobic efforts (Hazell et al., 2010) and poses a considerable metabolic challenge to skeletal muscle as large reductions in muscle glycogen and pH and increases in blood lactate as well as whole body carbohydrate and fat oxidation (Stepto et al., 2001). A wide range of adaptations have been shown after this type of training, including increased resting glycogen content (Barnett et al., 2004; Rodas et al., 2000), increased activity of various glycolytic and oxidative enzymes (Burgomaster et al., 2005; Jacobs et al., 1987), H+ buffering capacity (Gibala et al., 2006), and time to exhaustion (Burgomaster et al., 2005; Gibala et al., 2006). Increased (Rodas et al., 2000; Tabata et al., 1996) or unchanged (Burgomaster et al., 2005) values of VO2max after SIT have also been reported. As a result, it has been speculated that SIT could be a time-efficient strategy for health promotion. Nonetheless, use of 30-s “all- out” efforts as a training program is often dismissed outright as unsafe, unpractical, or intolerable for general populations. Therefore, an evaluation of the physiological adaptations induced by different interval-training strategies in a wide range of populations will permit evidence-based recommendations that may provide an alternative to current exercise prescriptions for time-pressed individuals. Accordingly, the purpose of this study was to compare the established SIT protocol (30-s “all-out” efforts with 4 min recovery) versus a modified type of HIT (30-s efforts with 125% Pmax with 2 min recovery) on both aerobic and anaerobic performance. The intensity of the modified training is the half of the intensity of SIT but with doubled repetitions. We hypothesized that the training induced changes are the same.
ParticipantsTwenty-four healthy young male graduate students volunteered to participate in the investigation (age = 25 ± 0.8 years; height = 1.72 ± 0.08 m; mass = 70 ± 11 kg; Percent body fat = 18 ± 6%). All were habitually active but not engaged in any sort of structured training program nor had they been for at least 3 months prior to the study. Prior to any participation, the experimental procedures and potential risks were explained fully to the participants and all provided written informed consent. Dietary and physical activity patterns were maintained throughout the study. Subjects were matched into three groups based on the time to exhaustion test and VO2max. The study was approved by the Ethical Committee of the Faculty of Medical Sciences of Tarbiat Modares University and was in accordance with the Declaration of Helsinki.
Baseline testingPrior to any baseline testing, all participants attended a laboratory familiarization visit to introduce the testing/training procedures and to ensure that any learning effect was minimal for the baseline measures. Each participant first completed tests for body composition and graded exercise test (GXT) to determine their VO2max and power at VO2max (Pmax). The second test was a Tmax test used to determine the time to exhaustion at Pmax. On the third day, the participants performed 30-second Wingate test to determine peak power output (PPO), mean power output (MPO) and total work (Wtot). All tests were separated by at least 24 h, post-testing occurred within 48 h of the final training session in the same order (Hazell et al., 2010).
Graded exercise testVO2max was determined using a progressive incremental test on a cycle ergometer until volitional fatigue. After 5 min warm-up and performing stretching exercises, the test commenced at an initial power output of 50 W and was increased by 30 W every minute until the participant was fatigued (Laursen et al., 2002a). Expired gas analysis was acquired with an automated metabolic system (ZAN600 CPET: ZAN Messgerate GmbH, Oberthulba, Germany), and VO2max was calculated as the highest oxygen consumed over a 1 min period. VO2max was confirmed when three or more of the following criteria were met: (1) a plateau in VO2 despite an increase in work load; (2) a respiratory exchange ratio (RER) higher than 1.2 (Esfarjani and Laursen, 2007); (3) peak heart rate at least equal to 90% of the age-predicted maximum (Tanaka et al., 2001); and/or (4) visible exhaustion. Pmax was defined as the final completed work rate (e.g., maintained for 10 s) (Laursen et al., 2002a).
Time to exhaustion at PThe second test performed was a Tmax test used to determine the time to exhaustion at Pmax that was defined as the minimal power output that elicited a VO2 reading that was within 2 ml·kg-1·min-1 of the previous reading, despite an increase in workload (Laursen et al., 2005). After a 10- minute warm-up at 50 W, participants cycled to fatigue at Pmax at a self-selected cadence; the test was stopped when cadence fell below 60 rev·min-1. The total amount of work completed during the Tmax test (WTmax) was calculated as a product of Pmax and Tmax (WTmax = (Pmax ËŸ Tmax)/1000) and expressed in kJ.
Wingate testPeak power output (PPO), mean power output (MPO) and total work (Wtot) were assessed over Wingate test on a mechanically braked cycle ergometer (model 894E, Monark, Sweden). Participants performed a Wingate test against a resistance equivalent to 0.075 kg/kg body mass. Participants were instructed to begin pedaling as fast as possible against the ergometer’s inertial resistance, and then the appropriate load was manually applied. Participants were verbally encouraged to continue pedaling as fast as possible throughout the 30-s test. PPO was defined as the highest work output in a 5-second period, and MPO as the average work output for the 30-second test period (Nottle and Nosaka, 2007). Wtot was used to describe muscle endurance and was calculated by multiplying MP by 30 seconds and expressed in kJ. Previously determined intraclass correlation coefficient (ICC) for Wingate variables was 0.94 (Bar-Or, 1987).
Training interventionParticipants were assigned to one of the three groups: (G1) 30-s all-out efforts: 4 min recovery; (G2) 30-s with 125% Pmax: 2 min recovery and control group (no training) (Table 1). Participants trained three times per week on alternate days for a total of 4 wk. The G1 cycling program began with 3 intervals in week 1, progressing to 5 in week 3 followed by a reduction to 4 intervals in week four. The G2 cycling program began with 6 intervals in week 1, progressing to 10 in week 3 followed by a reduction to 8 intervals in week four.
Blood lactateCapillary blood samples were taken (by finger prick) from the forefinger at rest, 3, and 20 min after the Wingate trial. Blood lactate was analyzed on site using a Lactate Analyzer (Lactate Scout, Senslab GmbH Leipzig, Germany).
Data analysisAll results are reported as a mean ± SD. The Kolmogorov-Smirnov test was used to test the normality of the distribution. A 3 ËŸ 2 (Group ËŸ time) repeated measures analysis of variance (ANOVA) was performed. When a significant difference was revealed, Tukey’s post hoc test was used to specify where the difference occurred. The alpha level for statistical significance was set at p ≤ 0.05. Effect size was calculated using Cohen’s d (d).
Changes in physiological variables associated with the GXT are presented in Table 2. Following the 4-week training program, VO2max (G1: d = 1.10; G2: d = 1.19), Pmax (G1: d = 1.27; G2: d = 1.77), Tmax (G1: d = 3.13; G2: d= 3.53) and WTmax (G1: d = 2.49; G2: d = 3.02) were significantly increased in the G1 and G2 groups compared with pre-testing. As well, the increases in Pmax (G1: p = 0.011, d = 1.31; G2: p = 0.006, d = 1.58), Tmax (G1: p = 0.00, d = 3.01; G2: p = 0.00, d = 3.34) and WTmax (G1: p = 0.00, d = 3.38; G2: p = 0.00, d = 4.09) were significantly greater in G1 and G2 compared with CON group. No variables were significantly changed in the CON group. PPO increased significantly in G1 (p = 0.006, d = 1.48; Table 3) and G2 (p = 0.04, d = 1.86) after the training. As well, the increase in PPO was significantly greater in G1 compared with CON group (p = 0.009, d = 1.66). In addition, MPO increased significantly in G1 compared with pre-testing (p = 0.025, d = 1.32; Table 3) and the CON group (p = 0.017, d = 1.49). MPO in G2 did not change significantly with the training (p = 0.064). There were significant increases in Wtot in G1 (p = 0.025, d = 1.38; Table 3), which were statistically significant compared with CON group (p = 0.017, d = 1.52). After the 4-week training period, maximal BLa_ (3th min) was significantly higher in G1 compared with pre-testing (Post: 15.6 ± 1.6 vs. Pre: 13.5 ± 1.3 mmol·l-1; p = 0.01, d = 1.45; Figure 1) and CON group (G1: 15.6 ± 1.6 vs. CON: 13.1 ± 1.2 mmol·l-1; p = 0.003, d = 1.76). Maximal BLa_ in G2 was only significantly different compared with CON group (G2: 14.8 ± 1.6 vs. CON: 13.1 ± 1.2 mmol·l-1; p = 0.032, d = 1.2). Blood lactate recovery (20th min) significantly decreased in G1 (Post: 12.9 ± 1.8 vs. Pre: 15.8 ± 3.0 mmol·l-1; p = 0.007, d = 1.21) and G2 (Post: 13.0 ± 1.0 vs. Pre: 15.1 ± 1.4 mmol·l-1; p = 0.04, d = 1.75) when compared with pre-testing and the CON group (p < 0.05).
Recent evidence suggests that sprint interval training is a time-efficient strategy to stimulate a number of skeletal muscle adaptations that are comparable to traditional endurance training (Gibala and McGee, 2008). It has been demonstrated that SIT up-regulates peroxisome proliferator-activated receptor-co-activator-1α (PGC-1α), a potent regulator of mitochondrial biogenesis (Gibala et al., 2009), which could be the underlying mechanism responsible for the observed aerobic adaptation. This information is exciting because it means that such adaptations can be obtained with a substantial reduction in exercise training time. However, the feasibility of this type of training is questionable for general populations. Therefore, the purpose of the current study was to compare the established SIT protocol versus a modified type of HIT on both aerobic and anaerobic performance. The first finding of the present study was that both training programs significantly and similarly improved VO2max in untrained subjects (G1: 9.6% vs. G2: 9.7%; Table 2). Several studies have shown an increase in VO2max following established SIT program (Creer et al., 2004; Barnett et al., 2004). In contrast to these findings, Burgomaster et al., 2005 recently showed in untrained subjects that six sessions of sprint interval training (30-s all-out Wingate sprints) increased citrate synthase activity by 38% and doubled cycle time to exhaustion. Interestingly, no change occurred in VO2max. Improvements in VO2max may occur through increases in both oxygen delivery (i.e., increases in stroke volume) and/or oxygen utilization by active muscles (i.e., increases in capillarization/mitochondrial density). Given that maximal heart rate remains unchanged in response to training, improvements in oxygen delivery to exercising muscles during high-intensity exercise can be attributed to an increase in stroke volume. Stroke volume can increase through a higher left-ventricular contractile force and/or through an increase in cardiac filling pressure, which raises end-diastolic volume and resultant stroke volume (Laursen and Jenkins, 2002b). In addition, the increases in VO2max and oxidative enzymes that has been reported by several authors (Barnett et al., 2004; Gibala et al., 2006) indicate the present sprint-training protocol evoked changes in the capacity to produce energy via oxidative metabolism. It is also known that the aerobic contribution to sprint exercise increases, depending on the recovery between bouts, as a function of successive sprints (Bogdanis et al., 1996). The relatively short recovery periods used in the present study would have imposed a considerable demand on aerobic metabolism in meeting ATP resynthesis in the latter sprint bouts. All of these factors may explain the observed increase in VO2max. Tmax significantly increased in both groups following training (G1: 48% vs. G2: 54%; Table 2). Tmax improvements are greater than those reported by Esfarjani and Laursen, 2007 who reported a 32% improvement in moderately trained runners using HIT prescribed at 130% vVO2max with interval durations of 30-s. In addition, 30-s all-out SIT program (over 2 wk) has been shown to improve (100%) cycle time to exhaustion at 80% VO2max in untrained subjects (Burgomaster et al., 2005). Franch et al., 1998 also reported a significant increase (65%) in time to exhaustion after 6 weeks of short-interval training. From a metabolic point view, the increased mitochondrial enzyme content, which is associated with an increase in the rate of lipid utilization and consequently a decrease in rate of glycogen depletion contributes to an improved exercise tolerance, the glycogen depletion being strongly linked with fatigue during prolonged exercise (Demarle et al., 2003). Interestingly, a comprehensive work by Harmer et al., 2000 indicated a reduced anaerobic ATP synthesis during intense exercise following seven weeks of sprint training, and also suggested an enhanced aerobic metabolism. Another possible reason for the improved Tmax found in the training groups may be a decreased lactate accumulation throughout the test and/or a greater muscle buffering capacity, which has been demonstrated in repeated 30-s sprint training (Gibala et al., 2006). It is possible that the greater improvement in Tmax in G2 versus G1 was aided through the higher training volume in G2 (6-10 30-s bouts) compared with G1 (3-5 30-s bouts). PPO elicited during the Wingate test increased by 10.3% and 7.3% in G1 and G2, respectively, with no difference between groups (Table 3); whereas, MPO was increased only in G1 by 17.1% (p < 0.05). This result is consistent with a greater reliance on glycolysis during training in G1 and could explain at least partially why the less intense 30-s repeats seemed less difficult. Such results are in accordance with previous studies reporting an improvement in PPO with SIT. Burgomaster et al., 2005 reported increase in PPO after 2 weeks of SIT but MPO did not change in their investigation. Increased muscle phosphocreatine concentration (Rodas et al., 2000), anaerobic enzyme activities (MacDougall et al., 1998; Parra et al., 2000), and a significant increase in FTa fibers, along with a decrease in ST fibers (Jansson et al., 1990; Dawson et al., 1998), are possible explanations of our findings. As expected with SIT, there was an increase in maximal blood lactate from pre to post training in G1 (15.5%; Figure 1). An increase in maximal BLa? in post-testing coinciding with increased peak power, mean power, and total work in G1. Sharp et al., 1986 reported an increase in blood lactate concentrations and total work performed during a 45-s maximal cycle sprint after eight weeks of intense sprint training in untrained subjects. These data were reported in conjunction with an increase in the glycolytic enzyme phosphofructokinase (PFK), suggesting that increased lactate and total work values were due to improved glycolytic output. Increases in glycolytic enzymes such as hexokinase and PFK due to SIT have been shown as well (MacDougall et al., 1998; Rodas et al., 2000), indicating that increases in blood lactate and total work values in G1 may be due to improved glycolytic function. Interestingly, Sharp and colleague (1986) saw no change in muscle pH in spite of increased lactate concentrations suggesting an increase in buffering capacity, which may have also contributed to increases in total work in the current study in spite of increased blood lactate concentrations. However, the changes in G2 did not reach to significant level (p = 0.05). In addition, blood lactate recovery changed significantly and similarly with either training regimen (Figure 1). As a result, 30-s all-out SIT should be easily incorporated into the training program of any athlete desiring to increase both aerobic and anaerobic power quickly but not into the training program of general population. The Wingate-based training model requires a specialized ergometer and an extremely high level of subject motivation. Given the extreme nature of the exercise, it is doubtful that the general population could safely or practically adopt the model. Like the our study, future studies should examine modified interval-based approaches to identify the optimal combination of training intensity and volume necessary to induce adaptations in a practical time-efficient manner.
The results of the current study agree with earlier work demonstrating the effectiveness of 30-s all-out SIT to aerobic and anaerobic adaptations. However, of substantial interest is our observation that many of the same performance gains occurred in modified HIT protocol (30-s with 125% Pmax).
ACKNOWLEDGEMENTS |
This work was supported by the Research Center of Tarbiat Modares University (TMU), Tehran, Iran. The authors would like to acknowledge the participants of this study for their time and efforts. |
|
AUTHOR BIOGRAPHY |
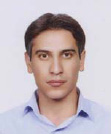 |
Mahdi Bayati |
Employment: PhD student in exercise physiology at Tarbiat Modares University, Tehran, Iran |
Degree: MSc |
Research interests: High-intensity interval training and testing. |
E-mail: m.bayati@modares.ac.ir |
|
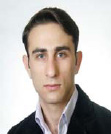 |
Babak Farzad |
Employment: Department of Physical Education and Sports Sciences, Faculty of Humanities, Tarbiat Modares University, Tehran, Iran. |
Degree: MSc |
Research interests: Exercise training induced metabolic and hormonal changes and exercise training and public health. |
E-mail: farzad.babak@gmail.com |
|
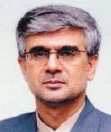 |
Reza Gharakhanlou |
Employment: Associate professor at the Tarbiat Modares University, Tehran, Iran |
Degree: PhD |
Research interests: Neuromuscular adaptation to exercise. |
E-mail: ghara_re@modares.ac.ir |
|
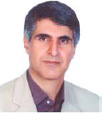 |
Hamid Agha-Alinejad |
Employment: Assistant professor at the Tarbiat Modares University, Tehran, Iran |
Degree: PhD |
Research interests: Exercise immunology. |
E-mail: halinejad@modares.ac.ir |
|
|
|
REFERENCES |
 Babraj J.A., Vollaard N.B., Keast C., Guppy F.M., Cottrell G., Timmons J.A. (2009) Extremely short duration high intensity interval training substantially improves insulin action in young healthy males. BMC Endocrine Disorders 28, 3-.
|
 Bar-Or O. (1987) The Wingate anaerobic test. An update on methodology, reliability and validity. Sports Medicine 4, 381-394.
|
 Barnett C., Carey M., Proietto J., Cerin E., Febbraio M.A., Jenkins D. (2004) Muscle metabolism during sprint exercise in man: influence of sprint training. Journal of Science and Medicine in Sport 7, 314-322.
|
 Blair S.N., LaMonte M.J., Nichaman M.Z. (2004) The evolution of physical activity recommendations: how much is enough?. American Journal of Clinical Nutrition 79, 913-.
|
 Bogdanis G.C., Nevill M.E., Boobis L.H., Lakomy H.K. (1996) Contribution of phosphocreatine and aerobic metabolism to energy supply during repeated sprint exercise. Journal of Applied Physiology 80, 876-884.
|
 Burgomaster K.A., Howarth K.R., Phillips S.M., Rakobowchuk M., Macdonald M.J., McGee S.L., Gibala M.J. (2008) Similar metabolic adaptations during exercise after low volume sprint interval and traditional endurance training in humans. The Journal of physiology 586, 151-160.
|
 Burgomaster K.A., Hughes S.C., Heigenhauser G.J.F., Bradwell S.N., Gibala M.J. (2005) Six sessions of sprint interval training increases muscle oxidative potential and cycle endurance capacity in humans. Journal of Applied Physiology 98, 1985-1990.
|
 Creer A.R., Ricard M.D., Conlee R.K., Hoyt G.L., Parcell A.C. (2004) Neural, metabolic, and performance adaptations to four weeks of high intensity sprint-interval training in trained cyclists. International Journal of Sports Medicine 25, 92-98.
|
 Dawson B., Fitzsimons M., Green S., Goodman C., Carey M., Cole K. (1998) Changes in performance, muscle metabolites, enzymes and fibre types after short sprint training. European Journal of Applied Physiology 78, 163-169.
|
 Demarle A.P., Heugas A.M., Slawinski J.J., Tricot V.M., Koralsztein J.P., Billat V.L. (2003) Whichever the initial training status, any increase in velocity at lactate threshold appears as a major factor in improved time to exhaustion at the same severe velocity after training. Archives of Physiology & Biochemistry 111, 167-176.
|
 Esfarjani F., Laursen P.B. (2007) Manipulating high intensity interval training: Effects on VO2max, the lactate threshold and 3000m running performance in moderately trained males. Journal of Science and Medicine in Sport 10, 27-35.
|
 Franch J., Madsen K., Djurhuus M.S., Pedersen P.K. (1998) Improved running economy following intensified training correlates with reduced ventilatory demands. Medicine and Science in Sports and Exercise 30, 1250-1256.
|
 Gibala M.J., Little J.P., Essen M.V., Wilkin G.P., Burgomaster K.A., Safdar A., Raha S., Tarnopolsky M.A. (2006) Short-term sprint interval versus traditional endurance training: similar initial adaptations in human skeletal muscle and exercise performance. Journal of Physiology 575, 901-911.
|
 Gibala M.J., McGee S.L. (2008) Metabolic adaptations to short-term high-intensity interval training: a little pain for a lot of gain?. Exercise and Sport Sciences Reviews 36, 58-63.
|
 Gibala M.J., McGee S.L., Garnham A.P., Howlett K.F., Snow R.J., Hargreaves M. (2009) Brief intense interval exercise activates AMPK and p38 MAPK signaling and increases the expression of PGC-1alpha in human skeletal muscle. Journal of Applied Physiology 106, 929-934.
|
 Godin G., Desharnais R., Valois P., Lepage L., Jobin J., Bradet R. (1994) Differences in perceived barriers to exercise between high and low intenders: observations among different populations. American Journal of Health Promotion 8, 279-285.
|
 Harmer A.R., Mckenna M.J., Sutton J.R., Snow R.J., Ruell P.A., Booth J., Thompson M.W., Mackay N.A., Stathis C.G., Crameri R.M., Carey M.F., Eager D.M. (2000) Skeletal muscle metabolic and ionic adaptation during intense exercise following sprint training in humans. Journal of Applied Physiology 89, 1793-1803.
|
 Hazell T.J., MacPherson R.E.K., Gravelle B.M.R., Lemon P.W.R. (2010) 10 or 30-s sprint interval training bouts enhance both aerobic and anaerobic performance. European Journal of Applied Physiology 110, 153-160.
|
 Jacobs I., Esbjörnsson M., Sylvén C., Holm I., Jansson E. (1987) Sprint training effects on muscle myoglobin, enzymes, fiber types, and blood lactate. Medicine and Science in Sports and Exercise 19, 368-374.
|
 Jansson E., Esbjörnsson M., Holm I., Jacobs I. (1990) Increase in the proportion of fast-twitch muscle fibres by sprint training in males. Acta Physiologica Scandinavica 140, 359-363.
|
 Laursen P.B., Blanchard M.A., Jenkins D.G. (2002a) Acute high-intensity interval training improves Tvent and peak power output in highly trained males. Canadian Journal of Applied Physiology 27, 336-348.
|
 Laursen P.B., Jenkins D.G. (2002b) The scientific basis for high-intensity interval training: optimizing training programs and maximizing performance in highly trained endurance athletes. Sports Medicine 32, 53-73.
|
 Laursen P.B., Shing C.M., Peake J.M., Coombes J.S., Jenkins D.G. (2005) Influence of high-intensity interval training on adaptations in well-trained cyclists. Journal of Strength and Conditioning Research 19, 527-533.
|
 MacDougall J.D., Hicks A.L., MacDonald J.R., Mckelvie R.S., Green H.J., Smith K.M. (1998) Muscle performance and enzymatic adaptations to sprint interval training. Journal of Applied Physiology 84, 2138-2142.
|
 Nottle C., Nosaka K. (2007) Changes in power assessed by the Wingate Anaerobic Test following downhill running. Journal of Strength and Conditioning Research 21, 145-150.
|
 Nybo L., Sundstrup E., Jakobsen M.D., Mohr M., Hornstrup T., Simonsen L., Bülow J., Randers M.B., Nielsen J.J., Aagaard P., Krustrup P. (2010) High-intensity training versus traditional exercise interventions for promoting health. Medicine and Science in Sports and Exercise 42, 1951-1958.
|
 Parra J., Cadefau J.A., Rodas G., Amigó N., Cussó R. (2000) The distribution of rest periods affects performance and adaptations of energy metabolism induced by high-intensity training in human muscle. Acta Physiologica Scandinavica 169, 157-165.
|
 Rodas G., Ventura J.L., Cadefau J.A., Cusso R., Parra J. (2000) A short training program for the rapid improvement of both aerobic and anaerobic metabolism. European Journal of Applied Physiology 82, 480-486.
|
 Sharp R.L., Costill D.L., Fink W.J., King D.S. (1986) Effects of eight weeks of bicycle ergometer sprint training on human muscle buffer capacity. International Journal of Sports Medicine 7, 13-17.
|
 Stepto N.K., Martin D.T., Fallon K.E., Hawley J.A. (2001) Metabolic demands of intense aerobic interval training in competitive cyclists. Medicine and Science in Sports and Exercise 33, 303-310.
|
 Tabata I., Nishimura K., Kouzaki M., Hirai Y., Ogita F., Miyachi M., Yamamoto K. (1996) Effects of moderate-intensity endurance and high-intensity intermittent training on anaerobic capacity and VO2max. Medicine and Science in Sports and Exercise 28, 1327-1330.
|
 Tanaka H., Monahan K.D., Seals D.R. (2001) Age-predicted maximum heart rate revisited. Journal of the American College of Cardiology 37, 153-156.
|
|
|
|
|
|
|