|
|
|
ABSTRACT |
In swimming competition, optimal swimming performance is characterized by a variety of interchangeable components, such as aerobic exercise capacity, anaerobic power and muscular function. Various hypoxic training methods would potentiate greater performance improvements compared to similar training at sea-level. Therefore, this study aimed to evaluate the effects of six-weeks of hypoxic training on exercise performance in moderately trained competitive swimmers. Twenty swimmers were equally divided into a normoxic training group (n = 10) for residing and training at sea-level (PIO2 = 149.7 mmHg), and a hypoxic training group (n = 10) for residing at sea-level but training at 526 mmHg hypobaric hypoxic condition (PIO2 = 100.6 mmHg). Aerobic exercise capacity, anaerobic power, muscular function, hormonal response and 50 and 400 m swimming performance were measured before and after training, which was composed of warm-up, continuous training, interval training, elastic resistance training, and cool-down. The training frequency was 120 min, 3 days per week for 6 weeks. Muscular function and hormonal response parameters showed significant interaction effects (all p < 0.032, η2 > 0.288) in muscular strength and endurance, growth hormone; GH, insulin like growth factor-1; IGF-1, and vascular endothelial growth factor; VEGF. The other variables demonstrated no significant interaction effects. However, a hypoxic training group also showed significantly increased maximal oxygen consumption; VO2max (p = 0.001), peak anaerobic power (p = 0.001), and swimming performances for 50 m (p = 0.000) and 400 m (p = 0.000). These results indicated that the hypoxic training method proposed in our study is effective for improvement of muscular strength and endurance in moderately trained competitive swimmers compared to control group. However, our hypoxic training method resulted in unclear changes in aerobic exercise capacity (VO2max), anaerobic power, and swimming performance of 50 m and 400 m compared to normoxic training. |
Key words:
Hypoxic training, muscular strength and endurance, normoxic exercise performance, swimmers
|
Key
Points
- Hypoxic training composed or warm-up, continuous training, interval training, resistance training, elastic resistance training, and cool-down with proper methodology had a positive effect on the muscular strength, muscular endurance and hormonal response (GH, IGF-1, and VEGF) of moderately trained competitive swimmers.
- Our hypoxic training method resulted in unclear changes in aerobic exercise capacity (VO2max), anaerobic power, and swimming performance of 50 m and 400 m compared to normoxic training.
|
Altitude/hypoxic training is a common and popular practice among various athletes, coaches, and sports scientists for improving exercise performance at the sea-level. The most typical altitude/hypoxic training methods proposed include living high-training high (LHTH) or living high-training low (LHTL). Recently, various living low training high (LLTH) methods such as intermittent hypoxic training (IHT), repeated sprint training in hypoxia (RSH), and resistance training in hypoxia (RTH) has become an increasingly popular altitude/hypoxic practice, where athletes live at or near sea-level but train at 2,000 to 4,500 m simulated hypobaric or normobaric hypoxic conditions (Faiss et al., 2013a; Girard et al., 2017; Hamlin et al., 2010). Among the various LLTH altitude/hypoxic training methods, IHT may be of interest to athletes and coaches because this training method commonly involves shorter hypoxic exposure time and duration. Such hypoxic exposure typically last < 3 hours, two to five times per week, for 2 to 6 weeks (McLean et al., 2014). Theoretically, the stress from hypobaric or normobaric hypoxic exposure, in addition to the training stress, will compound the training adaptations experienced with normal aerobic training and will lead to greater improvements in aerobic exercise capacity (Czuba et al., 2011). IHT may trigger various biochemical and structural changes in skeletal and cardiac muscles that favor oxidative process; that is, IHT may enhance erythropoietic, metabolic, and hemodynamic functions, which results in the stimulation of serum erythropoietin synthesis, elevated red blood cell volume, improved exercise economy, increased blood flow, and thus in enhanced oxygen transporting and utilizing capacity of the blood (Czuba et al., 2011; Geiser et al., 2001; Hamlin et al., 2010). However, the efficiency of IHT for the enhancement of aerobic exercise capacity in various athletes at sea-level is controversial. Several studies reported improved aerobic exercise capacity by enhanced oxygen transporting or utilizing capacity of the blood after IHT (Czuba et al., 2011; Ponsot et al., 2006), although numerous studies did not support the improvement effect on aerobic exercise performance after IHT (Beidleman et al., 2009; Katayama et al., 2004; Rodriguez et al., 2007; Roels et al., 2007). Moreover, some researchers reported that there are no additional benefits of IHT on anaerobic performance when compared to similar normoxic training (Faiss et al., 2013a; Millet et al., 2014). To overcome some of inherent limitations of IHT (lower training stimulus due to hypoxia), RSH is being utilized, and this method improved the anaerobic power of athletes with significant molecular adaptations and larger blood perfusion variations in active muscles, increased lactate tolerance and muscle buffering capacity, increased glycolytic enzyme activity (Faiss et al., 2013b; Galvin et al., 2013; Hamlin et al., 2017). Recently, some researchers have attempted to identify the effect of RTH on muscular function (strength and endurance) via greater accumulation of metabolites (e.g., blood lactate level, inorganic phosphate, and hydrogen ion), greater hormonal response (e.g., growth hormone; GH, insulin-like growth factor 1; IGF-1, vascular endothelial growth factor; VEGF, epinephrine, norepinephrine, cortisol, and testosterone), neuromuscular adaptation and muscle hypertrophy (Kon et al., 2010; 2012; Manimmanakorn et al., 2013; Nishimura et al., 2010; Scott et al., 2014). Based on previous studies, the effect of RTH on the improvement of muscular strength and endurance has been reported to be caused by the accumulation of metabolites (blood lactate level), augmented hormone response (GH, IGF-1, VEGF, epinephrine, norepinephrine, and testosterone), and muscle hypertrophy (Kon et al., 2010; 2012; Nishimura et al., 2010; Scott et al., 2014). However, the mechanism for the improvement of muscular function by RTH currently is unclear, and there is a point of debate among scientists. Unlike the positive results of IHT, RSH, and RTH described above, the current several studies reported that no additional benefits on exercise performance (aerobic exercise capacity, anaerobic power, and muscular function) compared to the same training performed in normoxia (Friedmann et al., 2003; Kime et al., 2003; Puype et al., 2013). These conflicting reports on the efficacy of hypoxic training for improving aerobic exercise capacity, anaerobic power, and muscular function at sea-level may be due to methodological differences, including training type, volume, and intensity in the hypoxic conditions and the intensity and time of hypoxic stimulus (Czuba et al., 2011; Faiss et al., 2013a; McLean et al., 2014; Park et al., 2016). In other words, hypoxic training should be composed of appropriate training modality to maximize its effectiveness. In addition, investigating the effectiveness of IHT, RSH, and RTH for normoxic exercise performance in competitive swimmers who participate in almost all events (e.g., sprint, middle distance, and long distance) that require aerobic exercise capacity, anaerobic power, and pull and push muscular strength and endurance of the upper limbs would be valuable. Competitive swimmers perform a large volume with high-intensity practices to gain endurance, strength, and power (Hibberd et al. 2016). Therefore, this study aimed to investigate the effects of hypoxic training composed of warm-up, continuous training, interval training, elastic resistance training, and cool-down on the aerobic exercise capacity, anaerobic power, muscular function, and swimming performance of 50 and 400 m in moderately trained competitive swimmers. Based on this study purpose, we hypothesis that 6 weeks of hypoxic training is sufficient to elicit an increase in 50 and 400m swimming performance with improvement of aerobic exercise capacity, anaerobic power, and muscular function in moderately trained competitive swimmers.
ParticipantsThe participants were South Korean moderately trained competitive swimmer (n = 20) who were registered in the Korea Swimming Federation (Table 1). The participants received information about the study purpose and methods. They consented by signature after receiving sufficient explanation of the experiment and understanding the possible adverse effects prior to the start of the study. All the procedures followed were in accordance with the ethical standards of the responsible committee on human experimentation and the Declaration of Helsinki. This study was approved by the Institutional Review Board.
Study designThe study design is shown in Figure 1 and twenty of participants were equally divided for number (n = 10) and sex (5 men and 5 women) and assigned to one of the two method groups by VO2max, 50 m and 400 m swimming performance, a normoxic training group for sea-level training (760 mmHg, PIO2 = 149.7 mmHg) and a hypoxic training group for training in a simulated 3,000 m (526 mmHg, PIO2 = 100.6 mmHg) hypobaric hypoxic condition. There was no significant difference in body composition, VO2max, 50 m and 400 m swimming performance between groups (Table 1). All testing were performed in a 6.5 m (width) × 7.5 m (length) × 3 m (high) chamber with temperature (20 ± 2 C) and humidity (60 ± 2%) regulated by a thermo-hygrostat (Submersible Systems, Huntington Beach, CA). The research was conducted as follows: 8 days of pre-test sessions (i.e., 4 testing days and 1 day of rest between testing days), 6 weeks of training sessions under each environmental conditions, and finally, 8 days of post-test sessions. The post-test began three days after the last training session. On the first test day, blood samples were collected between 8:00 and 10:00 am after a day of fasting for analysis of blood variables related to muscular function at sea-level. Then, after the athletes were allowed to eat and relax during 4 hours, anaerobic power and muscular function were measured at normoxic condition and had an interval of two hours between measurements. On the second test day, maximal oxygen consumption (VO2max) and maximal heart rate (HRmax) were measured using a graded exercise testing protocol on a treadmill for evaluation of aerobic exercise capacity at normoxic condition. On the third test day, all participants measured the exercise load (watt) corresponding to 90% HRmax at each condition (control group: normoxic condition, IHT group: 526 mmHg hypoxic condition) using a graded exercise testing by the McArdle protocol on a bicycle. On the fourth test day, 50 m and 400 m swimming performance in a free-style was evaluated on authorized indoor swimming pool (50 m track) at sea-level in Seoul. All the participants performed the following five kinds of training in 120 min sessions: warm-up, continuous training, interval training, elastic resistance training, and cool-down. The training frequency was 120 min, 3 days per week for 6 weeks. Warm-up and cool-down were set at 50% HRmax for each participant for 5 min and then increased by 10% HRmax every 5 min and performed for 15 min. The continuous training sessions consisted of 30 min of continuous treadmill exercise that corresponded to 80% of the HRmax. The running velocity on a treadmill was changed using a heart rate monitor (Polar S610i, Finland) to match 80% HRmax. The interval training sessions consisted of 10 repetitions of interval cycling exercise (2 min of exercise corresponding to 90% of the HRmax and 1 min rest). The measured bicycle load with 90% HRmax during maximal bicycle exercise at each environmental condition on the third pre-test day was set as the exercise intensity during interval training session. All participants performed elastic resistance training sessions consisted of push up, bent over row, shoulder stretch, front raise, and bent over triceps kickback. The participants performed 3 sets of 6-8 repetitions at an exercise intensity ranging from 8 to 9 on the OMNI-Resistance Exercise Scale of Perceived Exertion (OMNI-RES AM; from 0, extremely easy to 10, extremely hard), which has been reported to correspond to exercise intensity levels ranging from 80% to 90% of 1 RM, with rest for 60 s per set (Colado et al., 2012). Elastic resistance training sessions were conducted for approximately 30 min. Also, all swimmers performed equally additional normoxic training session composed of swimming training session; warm-up, drills, main set, and swim down and resistance training session; bench press, shoulder press, dumbbell curl, lat pull down, bent over rowing, bent over back, push up, front push, front raise, and bent over triceps kickback at sea-level. The swimming training session is shown in Table 2 and participants performed resistance training; 3 sets of 8-10 repetitions at exercise intensity range from 70% to 80% of 1RM and were rested for 60 second per set. Additional normoxic training sessions were conducted on days when no training was given in each environmental condition. The swimming training session was performed for 2 to 3 hours and the resistance training was performed for 1 hour. The frequency of additional level training was 2 days per week for 6 weeks. All participants were rested on the weekend without any exercise.
MeasurementsIn our study, the aerobic exercise capacity, anaerobic power, muscular function, hormonal response, and swimming performance of 50 m and 400m were measured to examine changes in the exercise performance of moderately trained competitive swimmers by 6 weeks of training in each environmental condition. For measurement of aerobic exercise capacity, VO2max was measured before and after training using the Bruce protocol for graded exercise testing on a treadmill (Precor 932i, USA), with a Vmax-229 breath-by-breath auto metabolism analyzer (SensorMedics, Yorba Linda, CA), a breathing valve in the facemask form, and a treadmill (Precor 932i, USA). To verify anaerobic power, after a 10-min self-selected warm-up, interspersed with three maximal sprints for approximately 5 s, the participants performed a 30 s Wingate test (Velotron Wingate version 1.0 software, Racermate Inc., Seattle, WA) on a bicycle (Monark Exercise AB, Vansbro, Sweden), in which they pedaled maximally against a constant load (male: 9.8% body weight, female: 9.5% body weight) while seated, and the load was applied after an initial acceleration phase of 3 s (Hamlin et al., 2010). Muscular function was evaluated in terms of muscular strength and endurance. Muscular strength was measured as 1 RM for the bench-press and latissimus dorsi (lat) pull-down. Before measuring 1 RM, the participants performed 10 repetitions of warm-up exercises at 50% of the perceived 1 RM and stretching of the major muscle group. Three maximal trial separated by 3 min of rest were used to determine individual 1 RM for each resistance exercise (Kon et al., 2012). Muscular endurances in the bench-press and lat-pull-down was measured as the maximal number of repetitions at 70% of 1 RM before and after training. Muscular exhaustion was defined as the moment when the weight ceased to move or the participants failed to maintain the prescribed pace. Following the test, the performed exercise volume was calculated as load i repetition and used as the measurement value of muscular endurance (Kon et al., 2014). Hormonal response related to muscular function was analyzed by the Green Cross Medical Foundation (Certified organization in The Korea Society for Laboratory Medicine). The concentrations of the following blood variables were quantified: GH, IGF-1, and VEFG. A 3-mL sample of venous blood was collected into SST (serum separating tube) for serum. Clot formation was ensured in the SST by centrifuging the sample at 3,500 rpm for 10 min. GH, IGF-1, and VEGF levels were measured by using the enzyme-linked immunosorbent assay (ELISA) method with GH, IGF-I, and VEGF Quantikine ELISA kits (R&D Systems, Minneapolis, MN). Swimming performance was measured in the freestyle 50 and 400 m time trials; it was measured twice by an automatic system installed on authorized indoor swimming pool (50 m) at sea-level in Seoul and the median time was used.
Statistical analysesMeans and standard deviations (SD) were calculated for each primary dependent variable. Normality of distribution of all outcome variables was verified using a Kolmogorov-Smirnov test. A two-way analysis (“group” × “time”) of variance with repeated measures on “time” factor was used to analyzing the effects of training programs on each dependent variable. Partial eta-squared (η2) was calculated as measures of effect size. If a significant effect was found, a Bonferroni method post-hoc test was used to identify within-group change over time. Additionally, paired t-test was used to compare post-training vs pre-training values of dependent variables in each group separately. Finally, Pearson’s correlation coefficient was used to analyze the correlation between the muscular function and the hormonal response. All analyses were performed using Statistical Package for Social Science (SPSS) version 23.0 (IBM Corp., Armonk, NY, USA). A priori, the level of significance was set at 0.05.
Aerobic exercise capacityBefore and after training data for aerobic exercise capacity in both groups are shown in Table 3. No significant interaction effect was observed in VO2max, but, significant main effects within time were observed (p < 0.001, η2 = 0.598). Post-hoc analyses found that swimmers in the IHT group significantly increased VO2max in both testing condition (p = 0.001).
Anaerobic powerAs shown in Table 3, there was no significant interaction effect in peak and mean anaerobic power, but significant main effects within time were observed in peak anaerobic power (p = 0.008, η2 = 0.402). Post-hoc analyses found that swimmers in the IHT group significantly increased peak anaerobic power via 6 weeks of training (p = 0.001).
Muscular function and hormonal responseTable 3 depicts pre- and post-training data for muscular strength, muscular endurance, and hormonal response related to muscular function in both training groups. The repeated two-way ANOVA analyses revealed significant interaction effect (all p < 0.032, η2 > 0.288) in all muscular function parameters. Post-hoc analyses found that control group revealed significant increase in bench press 1 RM (p = 0.033), bench press exercise volume (p < 0.001), lat pull down exercise volume (p < 0.001), GH (p < 0.001), and IGF-1 (p < 0.001), also, IHT group showed significant increase in bench-press 1 RM (p = 0.001), lat pull down 1 RM (p = 0.001), bench-press exercise volume (p < 0.001), lat pull down exercise volume (p < 0.001), GH (p < 0.001), IGF-1 (p < 0.001), VEGF (p = 0.023) in both testing condition. Also, there was no correlation between the muscular function and the hormonal response (data no shown).
Time trialBefore and after training data for time trial in both groups are shown in Table 3. No significant interaction effect was observed, but, significant main effects within time were observed in 50 m (p = 0.004, η2 = 0.451) and 400 m (p = 0.004, η2 = 0.466) time trial. Post-hoc analyses found that swimmers in the IHT group significantly increased 50 m (p < 0.001) and 400 m (p < 0.001) time trial in both testing condition.
Our study investigated the effects of hypoxic training composed of warm-up, continuous training, interval training, elastic resistance training, and cool-down on the aerobic exercise capacity, anaerobic power, muscular function, hormonal response, and swimming performance of 50 and 400 m in moderately trained competitive swimmers. The main finding of our study is that hypoxic training group had greater improvement in muscular function and hormonal response; higher muscular strength and endurance, GH, IGF-1, and VEGF in moderately trained competitive swimmers compared to normoxic training group. However, although the results of the correlation analysis are not presented, there was no significant correlation between muscular strength or endurance and circulating hormones such as GH, IGF-1, and VEGF. Also, there was no significant interaction effect on VO2max, peak anaerobic power, 50 m and 400 m swimming performance between hypoxic training group and normoxic training group. Most commonly, efficiency of the oxygen transport system is most often evaluated by VO2max (Czuba et al., 2011). Theoretically, LLTH method altitude/hypoxic training (e.g. IHT and RSH) may increase aerobic exercise capacity and endurance performance at sea-level by several biochemical and structural adaptive changes (Faiss et al., 2013a; Geiser et al., 2001; Hamlin et al., 2010). However, research findings on IHT as an effective protocol for enhancing aerobic exercise capacity and sports performance at normoxic condition are inconclusive. Czuba et al. (2011) evaluated the efficacy of IHT with 95% of lactate threshold workload on aerobic capacity and endurance performance in well-trained cyclists. As results, they reported a significant increase in aerobic exercise capacity (e.g. VO2max, VO2LT, WRmax and WRLT) after three weeks of intermittent hypoxic training (IHT) with 95% of lactate threshold intensity. Dufour et al. (2006) reported that a significant improvement in VO2max by 5%, after 6 weeks of IHT, without changes in blood O2-carrying capacity and significant modifications in VO2 kinetics. Roels et al. (2005) also observed a significant improvement of VO2max after high intensive interval training sessions in hypxic condition (PIO2 of 100 mm Hg) compared to normoxic condition (PIO2 of 160 mm Hg). However, Vallier et al. (1996) reported no significant increase in VO2max in five elite triathletes by 3 weeks of IHT. During 3 weeks, the subjects modified their usual training schedule (approximately 30 h a week), replacing three sessions of bicycling exercise by three sessions on a cycle ergometer in a hypobaric chamber simulating an altitude of 4,000 m (462 mmHg). Hendriksen and Meeuwsen (2003) conducted an experiment in which they evaluated the effect of intermittent training in hypobaric hypoxia condition on physical exercise at sea-level. Over a 10 days’ period, 16 male triathletes trained for 2 h each day on a cycle ergometer placed in a hypobaric chamber. Training intensity was at 60%–70% of the heart rate reserve. There were 8 subjects who trained at a simulated altitude of 2,500 m, the other 8 trained at sea level. A year later, a cross-over study took place. As results, the VO2max did not increase significantly. Our present results do not show additional benefit on VO2max by hypoxic training. In this regard, McLean et al. (2014) suggested that greater aerobic exercise capacity (e.g. VO2max) with IHT might be more likely if the following fulfilled: (1) high-intensity intervals are performed during hypoxic training and (2) additional normoxic training with sufficient intensity and volume. In our study, sufficient intensity and volume of additional normoxing training (swimming and resistance exercise) were performed with hypoxic training, but no positive effect was observed. These results are probably due to lack of stimulation of interval training on a bicycle performed in our study. In other words, we consider that interval training or RSH with higher intensity and shorter duration (<30 s) should be performed (Faiss et al., 2013a; McLean et al., 2014). Historically, altitude/hypoxic training was shown to improve aerobic exercise capacity. More recently, however, interest has grown in the effects of altitude/hypoxic training on anaerobic power (Galvin et al., 2013; Hamlin et al., 2010). As commonly known, enhancement of the anaerobic energy supply system requires high-intensity repeated or interval training regimens at hypoxic condition (Bonetti et al., 2006; Brocherie et al., 2017). Some authors suggest that high intensity of IHT or RSH may also improve anaerobic exercise performance (Bonetti et al., 2006; Brocherie et al., 2017; Faiss et al., 2013a; Hendriksen and Meeuwsen, 2003), possibly via increases in muscle buffering capacity and glycolytic enzyme activity (Faiss et al., 2013b; Galvin et al., 2013; Hamlin et al., 2017). However, anaerobic exercise capacity or power improvements are not likely to occur when the duration, time, and intensity of repeated or interval training sessions under altitude/hypoxic conditions are insufficient (Hendriksen and Meeuwsen, 2003; Ponsot et al., 2006). Brocherie et al. (2017) performed systemic review and meta-analysis of the effects of RSH versus repeated-sprint training in normoxia (RSN) on key components of sea-level physical performance and reported that high intensity of RSH induces greater improvement for mean repeated-sprint performance during sea-level repeated sprinting than RSN. The additional benefit observed for best repeated-sprint performance and VO2max for RSH versus RSN was not significantly different. Therefore, we hypothesized that in addition to hypoxic continuous training, a specific high intensity of interval training under altitude/hypoxic conditions or RSH would provide greater stimulus for improvement in anaerobic power. However, there was no significant interaction effect in peak and mean anaerobic power, and significant main effect within time was observed in peak anaerobic power; it was significantly increased only in hypoxic training group. In our study, the reason for not showing a remarkable improvement in anaerobic power is that the interval exercise intensity is relatively low as the exercise load (watt) with 90% HRmax measured before training. Like aerobic exercise capacity, we consider that anaerobic interval or repeated training with higher intensity and shorter duration (<30 s) will probably have a significant improvement in anaerobic power (Bonetti et al., 2006; Brocherie et al., 2017; Faiss et al., 2013a; Galvin et al., 2013). Most recently, altitude/hypoxic training has been proposed to enhance some of the adaptations associated with resistance training. RTH is known to improve muscular strength, power production, and muscular endurance through a number of adaptations, including hypertrophy and various biochemical changes (Kon et al., 2014; Manimmanakorn et al., 2013; Nishimura et al., 2010). Kon et al. (2014) investigated how hypoxia affects resistance exercise-induced muscle adaptations. Their results suggest that, in addition to increases in muscle size and strength, RTH may also lead to increased muscular endurance and the promotion of angiogenesis in skeletal muscle. Manimmanakorn et al. (2013) has found that hypoxic training in conjunction with low-load resistant exercise improved the peak maximum voluntary contraction in 3 s and the number of repetitions able to be performed at 20% 1 RM. In addition, hypoxic training substantially increased muscle cross-sectional area compared to exercise training alone. Nishimura et al. (2010) reported resistance training (70% 1 RM, 4 sets of 10 repetitions, exercised twice weekly for 6 weeks) under hypoxic conditions improves muscle strength and induces muscle hypertrophy faster than under normoxic conditions. Based on this rationale, we applied elastic resistance training to strengthen the muscular function (strength and endurance) of moderately trained competitive swimmers, and the strengthening of the muscular function was expected to have a high correlation with the circulating hormonal response such as GH, IGF-1, and VEGF. As a result, hypoxic training group showed greater increases in muscular strength and endurance and greater hormonal response of GH, IGF-1, and VEGF, which has anabolic effects, when compared to normoxic training group. However, as mentioned above, there was no significant correlation between muscular strength or endurance and circulating hormones such as GH, IGF-1, and VEGF. A few previous studies reported no significant correlation between changes in anaerobic hormonal response (e.g. GH, IGF-1, and testosterone) after resistance training and muscular strength, and that anaerobic hormone response does not have an additive effect on muscular function (Lange et al., 2002; Mitchell et al., 2013). Lange et al. (2002) reported that our results do not support a role for GH as a means of increasing muscle strength or mass, either alone or combined with RT, in healthy elderly men; although GH administration alone may induce changes in MHC composition. Mitchell et al. (2013) investigated to determine relationships between post-exercise changes in hormonal response factors (testosterone, GH, and IGF-1) in a moderately-sized cohort of young men exhibiting divergent resistance training-mediated muscle hypertrophy. As a result, they concluded post-exercise increases in circulating hormones are not related to hypertrophy following training. However, Kon et al. (2014) and Vogt et al. (2001) reported that RTH increased angiogenesis in skeletal muscle via the altitude/hypoxic resistance training-induced increase in VEGF level, and muscular endurance and VEGF levels have been reported to be highly correlated. These observations suggested that enhancement of muscular function by RTH is influenced by not only circulating hormonal response but also many other factors (e.g., neuromuscular function, acid-base equilibrium, and biochemical pathway). Also, it means that enhancement of muscular function is influenced by increases in skeletal muscle oxidative fiber types, activities of metabolic enzymes, improvement in muscle-buffering capacity, and capillarization (Kon et al., 2014). We believe that future research should elucidate the correlation between muscular function and other variables (e.g. concentration of metabolites, hormonal response, intramuscular signaling pathways), and the mechanism by which the improvement of the muscular function is manifested. Generally, swimmers perform swimming approximately 5,000 to 6,500 m per practice and accumulate a large volume of distance training with high-intensity practices to gain aerobic exercise capacity, anaerobic power, and muscular strength and power (Hibberd et al. 2016). In addition, swimmers participate in almost all events (e.g., sprint, middle distance, and long distance) that require aerobic exercise capacity, anaerobic power, pull and push muscular strength and endurance of the upper limbs. So, we applied additional normoxic training consisted of 2.6 km swimming exercise and resistance exercise and hypoxic training composed of warm-up, continuous training, interval training, elastic resistance training, and cool-down with proper methodology, including training type, volume, and intensity under altitude/hypoxic conditions for exercise performance in moderately trained competitive swimmers. However, there was no significant interaction effect in 50 m and 400 m swimming performance, and significant main effect within time was observed; it was significantly increased only in IHT group. Similar to our study results, many previous studies reported that hypoxic training has no additional benefits on exercise performance when compared to the same training performed in normoxia. The results of Roels et al. (2005) research are in accordance with those of Truijens et al. (2003), who found those 5 weeks of high-intensity training in a flume improved normoxic athletic performance in competitive swimmers, however, there was no effect of hypoxic training (15.3% O2). Hendriksen and Meeuwsen (2003) conducted a study in that they examined the effect of IHT. The participants trained for 10 days, 2 h daily on a cycle ergometer placed in a hypobaric chamber at a simulated altitude of 2,500 m. Training intensity was at 60–70% of the heart rate reserve. The results indicated no significant increase in normoxic exercise performance. These results are consistent with Hamlin et al. (2010). In this study, sixteen well-trained athletes completed 90 min of endurance training (60–70% of heart rate reserve), followed by two 30-s all-out sprints (Wingate test), daily, for 10 consecutive days. Nine subjects trained with an FIO2 set to produce arterial oxygen saturations of 88–82%, while seven subjects (placebo group) trained while breathing a normal gas mixture (FIO2=0.21). As a results, they reported that training in a hypoxic environment for 91 min/day for 10 consecutive days resulted in a clear 3.0% improvement in the mean 30 s power 2 days’ post-intervention, and beneficial but unclear changes in 30 s peak power, 20 km mean power and 20 km oxygen cost 2 and 9 days’ post-intervention. Based on these prior studies, we consider that higher intensity interval training or RSG with shorter duration should be applied to improve swimming performance by hypoxic training (Brocherie et al., 2017; Faiss et al., 2013a; McLean et al., 2014).
ConclusionsOur study demonstrates that hypoxic training composed of warm-up, continuous training, interval training, elastic resistance training, and cool-down with proper methodology had a positive effect on the muscular strength and endurance of moderately trained competitive swimmers. However, our hypoxic training method resulted in unclear changes in aerobic exercise capacity (VO2max), anaerobic power, and swimming performance of 50 m and 400m.
ACKNOWLEDGEMENTS |
The authors report no conflicts of interest with this manuscript. The experiments comply with the current laws of the country. This study was supported by a grant (NRF-2015M3C1B1019479) from the National Research Foundation funded by the Korean Government. |
|
AUTHOR BIOGRAPHY |
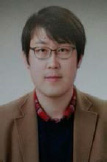 |
Hun-Young Park |
Employment: Research Scientist at Physical Activity and Performance Institute |
Degree: PhD |
Research interests: Training effect in hypoxic condition for sports performance, Clinical effects related to obesity and cardiovascular disease by exposure or training in hypoxic condition. |
E-mail: parkhy1980@konkuk.ac.kr |
|
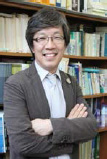 |
Kiwon Lim |
Employment: Professor of Exercise Nutrition and Director at Physical Activity and Performance Institute |
Degree: PhD |
Research interests: Effect of long-term high fat diet and/or exercise on energy metabolism at rest and during exercise. Clinical effects related to obesity and cardiovascular disease by exposure or training in hypoxic condition. |
E-mail: exercise@konkuk.ac.kr |
|
|
|
REFERENCES |
 Beidleman B.A., Muza S.R., Fulco C.S., Jones J.E., Lammi E., Staab J.E., Cymerman A. (2009) Intermittent hypoxic exposure does not improve endurance performance at altitude. Medicine and Science in Sports and Exercise 41, 1317-1325.
|
 Bonetti D.L., Hopkins W.G., Kilding A.E. (2006) High-intensity kayak performance after adaptation to intermittent hypoxia. International Journal of Sports Physiology and Performance 1, 246-260.
|
 Brocherie F., Girard O., Faiss R., Millet G.P. (2017) Effects of repeated-sprint training in hypoxia on sea-level performance: A meta-analysis. Sports Medicine 47, 1651-1660.
|
 Colado J.C., Garcia-Masso X., Triplett T.N., Flandez J., Borreani S., Tella V. (2012) Concurrent validation of the OMNI-resistance exercise scale of perceived exertion with Thera-band resistance bands. Journal of Strength and Conditioning Research 26, 3018-3024.
|
 Czuba M., Waskiewicz Z., Zajac A., Poprzecki S., Cholewa J., Roczniok R. (2011) The effects of intermittent hypoxic training on aerobic capacity and endurance performance in cyclists. Journal of Sports Science & Medicine 10, 175-183.
|
 Dufour S.P., Ponsot E., Zoll J., Doutreleau S., Lonsdorfer-Wolf E., Geny B., Lampert E., Flück M., Hoppeler H., Billat V., Mettauer B., Richard R., Lonsdorfer J. (2006) Exercise training in normobaric hypoxia in endurance runners. I. Improvements in aerobic performance capacity. Journal of Applied Physiology 100, 1238-1248.
|
 Faiss R., Girard O., Millet G.P. (2013a) Advancing hypoxic training in team sports: from intermittent hypoxic training to repeated sprint training in hypoxia. British Journal of Sports Medicine 47, i45-i50.
|
 Faiss R., Léger B., Vesin J.M., Fournier P.E., Eggel Y., Dériaz O., Millet G.P. (2013b) Significant molecular and systemic adaptations after repeated sprint training in hypoxia. PloS one 8, e56522.
|
 Friedmann B., Kinscherf R., Borisch S., Richter G., Bärtsch P., Billeter R. (2003) Effects of low-resistance/high-repetition strength training in hypoxia on muscle structure and gene expression. Pflügers Archive European Journal of Physiology 446, 742-751.
|
 Galvin H.M., Cooke K., Sumners D.P., Mileva K.N., Bowtell J.L. (2013) Repeated sprint training in normobaric hypoxia. British Journal of Sports Medicine 47, i74-i79.
|
 Geiser J., Vogt M., Billeter R., Zuleger C., Belforti F., Hoppeler H. (2001) Training high-living low: changes of aerobic performance and muscle structure with training at simulated altitude. International Journal of Sports Medicine 22, 579-585.
|
 Girard, O., Brocherie, F., and Millet, G.P. (2017) Effects of altitude/hypoxia
on single-and multiple-sprint performance: a
comprehensive review. Sports Medicine. Epub ahead of print.
|
 Hamlin M.J., Marshall H.C., Hellemans J., Ainslie P.N., Anglem N. (2010) Effect of intermittent hypoxic training on 20 km time trial and 30 s anaerobic performance. Scandinavian Journal of Medicine & Science in Sports 20, 651-661.
|
 Hamlin M.J., Olsen P.D., Marshall H.C., Lizamore C.A., Elliot C.A. (2017) Hypoxic repeat sprint training improves rugby player’s repeated sprint but not endurance performance. Frontiers in Physiology 8, 24.
|
 Hendriksen I.J., Meeuwsen T. (2003) The effect of intermittent training in hypobaric hypoxia on sea-level exercise: a cross-over study in humans. European Journal of Applied Physiology 88, 396-403.
|
 Hibberd E.E., Laudner K.G., Kucera K.L., Berkoff D.J., Yu B., Myers J.B. (2016) Effect of swim training on the physical characteristics of competitive adolescent swimmers. The American Journal of Sports Medicine 44, 2813-2819.
|
 Katayama K., Sato K., Matsuo H., Ishida K., Iwasaki K.I., Miyamura M. (2004) Effect of intermittent hypoxia on oxygen uptake during submaximal exercise in endurance athletes. European Journal of Applied Physiology 92, 75-83.
|
 Kime R., Karlsen T., Nioka S., Lech G., Madsen Ø., Sæterdal R., Im J., Chance B., Stray-Gundersen J. (2003) Discrepancy between cardiorespiratory system and skeletal muscle in elite cyclists after hypoxic training. Dynamic Medicine 2, 4.
|
 Kon M., Ikeda T., Homma T., Akimoto T., Suzuki Y., Kawahara T. (2010) Effects of acute hypoxia on metabolic and hormonal responses to resistance exercise. Medicine and Science in Sports and Exercise 42, 1279-1285.
|
 Kon M., Ikeda T., Homma T., Suzuki Y. (2012) Effects of low-intensity resistance exercise under acute systemic hypoxia on hormonal responses. The Journal of Strength & Conditioning Research 26, 611-617.
|
 Kon M., Ohiwa N., Honda A., Matsubayashi T., Ikeda T., Akimoto T., Suzuki Y., Hirano Y., Russell A.P. (2014) Effects of systemic hypoxia on human muscular adaptations to resistance exercise training. Physiological reports 2, e12033.
|
 Lange K.H.W., Andersen J.L., Beyer N., Isaksson F., Larsson B., Rasmussen M.H., Juul A., Bülow J., Kjær M. (2002) GH administration changes myosin heavy chain isoforms in skeletal muscle but does not augment muscle strength or hypertrophy, either alone or combined with resistance exercise training in healthy elderly men. The Journal of Clinical Endocrinology & Metabolism 87, 513-523.
|
 Manimmanakorn A., Hamlin M.J., Ross J.J., Taylor R., Manimmanakorn N. (2013) Effects of low-load resistance training combined with blood flow restriction or hypoxia on muscle function and performance in netball athletes. Journal of Science and Medicine in Sport 16, 337-342.
|
 McLean B.D., Gore C.J., Kemp J. (2014) Application of ‘live low-train high’ for enhancing normoxic exercise performance in team sport athletes. Sports Medicine 44, 1275-1287.
|
 Millet G., Bentley D.J., Roels B., Mc Naughton L.R., Mercier J., Cameron-Smith D. (2014) Effects of intermittent training on anaerobic performance and MCT transporters in athletes. PloS one 9, e95092.
|
 Mitchell C.J., Churchward-Venne T.A., Bellamy L., Parise G., Baker S.K., Phillips S.M. (2013) Muscular and systemic correlates of resistance training-induced muscle hypertrophy. PloS one 8, e78636.
|
 Nishimura A., Sugita M., Kato K., Fukuda A., Sudo A., Uchida A. (2010) Hypoxia increases muscle hypertrophy induced by resistance training. International Journal of Sports Physiology and Performance 5, 497-508.
|
 Park H.Y., Sunoo S., Nam S.S. (2016) The effect of 4 weeks fixed and mixed intermittent hypoxic training (IHT) on respiratory metabolic and acid-base response of capillary blood during submaximal bicycle exercise in male elite taekwondo players. Journal of Exerise Nutrition and Biochemistry 20, 35-43.
|
 Ponsot E., Dufour S.P., Zoll J., Doutrelau S., N’Guessan B., Geny B., Hoppeler H., Lampert E., Mettauer B., Ventura-Clapier R., Richard R. (2006) Exercise training in normobaric hypoxia in endurance runners. II. Improvement of mitochondrial properties in skeletal muscle. Journal of Applied Physiology 100, 1249-1257.
|
 Puype J., Van Proeyen K., Raymackers J.M., Deldicque L., Hespel P. (2013) Sprint interval training in hypoxia stimulates glycolytic enzyme activity. Medicine and Science in Sports and Exercise 45, 2166-2174.
|
 Rodriguez F.A., Truijens M.J., Townsend N.E., Stray-Gundersen J., Gore C.J., Levine B.D. (2007) Performance of runners and swimmers after four weeks of intermittent hypobaric hypoxic exposure plus sea level training. Journal of Applied Physiology 103, 1523-1535.
|
 Roels B., Bentley D.J., Coste O., Mercier J., Millet G.P. (2007) Effects of intermittent hypoxic training on cycling performance in well-trained athletes. European Journal of Applied Physiology 101, 359-368.
|
 Roels B., Millet G.P., Marcoux C.J., Coste O., Bentley D.J., Candau R.B. (2005) Effects of hypoxic interval training on cycling performance. Medicine and Science in Sports and Exercise 37, 138-146.
|
 Scott B.R., Slattery K.M., Sculley D.V., Dascombe B.J. (2014) Hypoxia and resistance exercise: a comparison of localized and systemic methods. Sports Medicine 44, 1037-1054.
|
 Truijens M.J., Toussaint H.M., Dow J., Levine B.D. (2003) Effect of high-intensity hypoxic training on sea-level swimming performances. Journal of Applied Physiology 94, 733-743.
|
 Vallier J.M., Chateau P., Guezennec C.Y. (1996) Effects of physical training in a hypobaric chamber on the physical performance of competitive triathletes.. European Journal of Applied Physiology and Occupational Physiology 73, 471-478.
|
 Vogt M., Puntschart A., Geiser J., Zuleger C., Billeter R., Hoppeler H. (2001) Molecular adaptations in human skeletal muscle to endurance training under simulated hypoxic conditions. Journal of Applied Physiology 91, 173-182.
|
|
|
|
|
|
|