|
|
|
ABSTRACT |
A combined intervention of strength and endurance training is common practice in elite swimming training, but the scientific evidence is scarce. The influences between strength and endurance training have been investigated in other sports but the findings are scattered. Some state the interventions are negative to each other, some state there is no negative relationship and some find bisected and supplementary benefits from the combination when training is applied appropriately. The aim of this study was to investigate the impact of a combined intervention among competitive swimmers. 20 subjects assigned to a training intervention group (n = 11) or a control group (n = 9) from two different teams completed the study. Anthropometrical data, tethered swimming force, land strength, performance in 50m, 100m and 400m, work economy, peak oxygen uptake, stroke length and stroke rate were investigated in all subjects at pre- and post-test. A combined intervention of maximal strength and high aerobic intensity interval endurance training 2 sessions per week over 11 weeks in addition to regular training were used, while the control group continued regular practice with their respective teams. The intervention group improved land strength, tethered swimming force and 400m freestyle performance more than the control group. The improvement of the 400m was correlated with the improvement of tethered swimming force in the female part of the intervention group. No change occurred in stroke length, stroke rate, performance in 50m or 100m, swimming economy or peak oxygen uptake during swimming. Two weekly dry-land strength training sessions for 11 weeks increase tethered swimming force in competitive swimmers. This increment further improves middle distance swimming performance. 2 weekly sessions of high- intensity interval training does not improve peak oxygen uptake compared with other competitive swimmers. |
Key words:
Oxygen consumption, muscle strength, metabolic efficiency
|
Key
Points
- Two weekly sessions of dry land strength training improves the swimming force.
- Two weekly sessions of high-intensity endurance training did not cause improved endurance capacity.
- It may seem that dry land strength training can improve middle distance performance.
|
Exercises to improve maximal muscle strength and maximal aerobic endurance capacity are essential elements for enhancing competitive swimming performance. Several studies have investigated the effects of maximal strength or strength- related training (Girold et al., 2006; 2007; Miyashita and Kanehisa, 1983; Petersen et al., 1984; Sharp et al., 1982; Tanaka et al., 1993; Toussaint and Vervoorn, 1990; Trappe and Pearson, 1994) or aerobic endurance capacity training (Barzdukas et al., 1992; Costill et al., 1988; 1991, D’Acquisto et al., 1992; Faude et al., 2008; Gergley et al., 1984; Houston et al., 1981; Kirwan et al., 1988; Konstantaki et al., 1999; Magel et al., 1975), but none have investigated a combined intervention even though it is common in modern competitive swimming. Former studies have found positive effects of interventions combining strength and endurance training in both basketball (Balabinis et al., 2003) and soccer athletes (Hoff et al., 2002b), along with runners (Tanaka et al., 1998; Yamamoto et al., 2008) and cyclists (Tanaka et al., 1998). The strength training approach is neural adaptations, simultaneously limiting muscular hypertrophy and increasing body weight (Sale, 2003). Endurance training improving maximal oxygen uptake (VO2max) is a response from enhanced cardiac stroke volume (O’Toole, 2000) prior to improving the oxidative capacity of the peripheral muscles (Helgerud et al., 2007). Both abilities have the potential to be improved without one influencing the other. Strength training in swimming improves swimming performance (Girold et al., 2006; 2007, Toussaint and Vervoorn, 1990; Trappe and Pearson, 1994) and performance-related parameters such as increased stroke length (Toussaint and Vervoorn, 1990), reduced stroke rate (Girold et al., 2006; 2007) and increased tethered swimming force (Girold et al., 2006; 2007, Toussaint and Vervoorn, 1990; Trappe and Pearson, 1994). Swimming velocity is the product of stroke length and stroke rate. However, of the three studies investigating the effects of dry-land strength training on swimming (Girold et al., 2007; Tanaka et al., 1993; Trappe and Pearson, 1994) only one found benefits between a combined strength and swim training group versus a swim-training only group (Girold et al., 2007). Neural adaptations may cause beneficial effects on work economy (Hoff et al., 2002a) through different mechanisms such as improved reflex potentiation, alterations of the synergists, alterations of the co-contraction of antagonist muscles and increases in electromyographic activity, but this approach has not been investigated in swimming although Rouard et al (Rouard et al., 1992) showed that muscular recruitment was higher for maximal swimming speed than mediocre speeds. Silva et al., 2007 used a feed forward neural network models method to predict 400m freestyle performance. They did not find any influence of dry land strength on performance, but swim velocity at lactate threshold was highly correlated with performance in both genders. The work economy at a given velocity can be improved by altering stroke length or stroke rate (Barbosa et al., 2008), and is considered one of the three major factors accounting for inter-individual variance in aerobic endurance performance along with VO2max and lactate threshold (Pate and Kriska, 1984), where VO2max is considered the most important of these (Helgerud et al., 2007; Åstrand et al., 2003). Thus, the improvement of VO2max is of major interest. A traditional pragmatic approach in swimming training has been to develop endurance capacity through high training volumes (Costill et al., 1988; 1991). This is supported by D’Acquisto et al (D’Acquisto et al., 1992) who found that VO2max improved in a 60 versus 20 minute training session intervention of the same intensity (76 ± 2% of VO2max) over 5 weeks in moderate level swimmers. However, 20 or 60 minute sessions 5 times a week are not representative training volumes for modern competitive swimming. Hence these findings must be further evaluated. In fact, five studies (Costill et al., 1988; 1991, Faude et al., 2008; Houston et al., 1981; Kirwan et al., 1988) have investigated traditional high training volumes versus high intensity training of lower volume. They all concluded that there seem to be no benefits of high volume compared to high intensity. However, the studies are detained with lack of control groups (Costill et al., 1988; Kirwan et al., 1988) or lack of subject-matching (Faude et al., 2008), expert, but merely regional level swimmers (Costill et al., 1991, Houston et al., 1981) or small intervention groups (Faude et al., 2008; Houston et al., 1981). Recently, Helgerud et al., 2007 investigated the same in treadmill running. They found that high intensity training is more efficient than medium- or low-intensity training of high volumes in improving VO2max. Although VO2max in swimming has been of major interest to researchers since the 1960’s (Magel and Faulkner, 1967), no studies have concluded which methods are more efficient in improving VO2max for competitive swimmers. This may partly rely on the methodological challenges in measuring VO2max in swimming (Rinehardt et al., 1991). The aim of this study was to investigate the effect of a combined intervention of maximal strength training and high-intensity interval training on swimming performance and performance related parameters such as swimming force, maximal velocity, swimming economy and maximal oxygen uptake.
SubjectsParticipants were included if they were above 14 years of age, free from injury and train regularly for at least 6 times a week. They were excluded if they failed to comply with more than 60% of the intervention. 26 subjects were included after written informed consent was signed by the subjects or their guardian for those under 18 years of age. The intervention group included 13 subjects from two teams and the control group included 13 subjects from three different teams. Efforts were made to recruit subjects for making comparable groups. Four of the control group subjects withdrew from the study during the intervention period because of injury not related to the investigation (one), illness (two) and personal reasons (one). One intervention group subject withdrew because of illness and another was excluded because of low compliance in strength training. There was an intention to recruit at least five subjects of each gender in each group, but the withdrawals left only two male subjects in the control group. The swimmers in the intervention group consisted of stroke specialists in freestyle (five persons), butterfly (three), breaststroke (two) and individual medley (one). Two of them were sprinters, the rest were middle or long distance swimmers. The control group consisted of 6 freestyle, one breaststroke, one backstroke and one butterfly swimmer. One of these was a sprinter. Lying body length was measured in a supine position with heels against the wall, and the distance from the wall to the top of the scull was measured. Reaching height was measured from heels to the tip of the middle finger in a supine position with 180º bilateral shoulder flexion, and palm of one hand on the back of the other. Body mass was measured on a balance scale (Type 304, Stathmos, Sweden). Physical characteristics for all groups are reported in Table 1.
Training InterventionsThe intervention group underwent a combined strength and endurance training regimen similar to Hoff et al (Hoff et al., 2002b). On average two combined training sessions were brought out per week, but due to vacations, competitions and a training camp, between one and three training sessions were carried out. A combined intervention was chosen as few swimmers were available and this approach best represents regular competitive swimming practice. Further, the mechanisms of the interventions have been described earlier and do not negatively influence each other. The endurance training was carried out in front crawl swimming in a 25m pool. Before each interval training the swimmers carried out a warm up of 20-40 minutes. A training regimen of 4 × 4 minute high intensity intervals separated by three minute moderate intensity periods was used (Helgerud et al., 2007). The four minute intervals were carried out at 90-95% of individual HRmax. The three minute separation periods and three minute cool down period consisted of moderate swimming at 60-75% of individual HRmax. The subject’s exercise heart rate was monitored using short range radio telemetry units (Polar Electro Oy, Kempele, Finland) during the interval training. Feedback was given immediately after each interval based on the heart rate at the end. Those swimmers who did not use a short range radio telemetry unit were instructed to increase velocity as steady as possible up to 100m, and then keep velocity at a maximal performance pace for the rest of the four minutes. They were instructed to measure heart rate by the pulse of the carotid artery and a pace watch over 10 seconds immediately after every interval. The velocity during the intervals was also monitored using a stop watch. The strength training consisted of a 5-10 minute cardiovascular warm up on an ergometer bike, a treadmill, or by swimming. In addition, a specific warm up procedure using 10-15 repetitions of 50-80% of 1RM in the strength training apparatus were performed before the intervention training. The strength training consisted of five maximal repetitions for three series with initial maximal mobilization of force in the concentric action and a slow eccentric phase at one side of a cable cross over apparatus. As the subjects managed to carry out a set of five repetitions, the load was increased by 1 kg. A 2-5 minutes rest interval was allowed between sets. The strength training was designed to imitate the butterfly stroke starting at approximately 170º shoulder extension with parallel hands on a bar and pulling the bar down to 10º shoulder extension by bilateral shoulder flexion in one movement. Thereby the primarily engaged muscles were the latissimus dorsi, triceps brachii and the rotator cuff which are all important in freestyle swimming. All strength training was supervised, and effort was put on all subjects to exercise with the heaviest load possible at each session. Swimmers were allowed to drop the elbow below the line between the hand and the shoulder to prevent from impingement-problems and possibly shoulder pain. The intervention group was under close surveillance during both strength training and high intensity intervals. Both groups kept an individual training diary (Østerås et al., 2002) which recorded how many meters the swimmer covered in total, in three intensity zones, and how much time was spent on dry land exercise. The zones were as follows: zone one (60-85% of HRmax), zone two (85-95% of HRmax) and zone three (>95% of HRmax). We included swimmers from 5 different teams and had an intention to register as individually correct training as possible. Therefore we felt the need to collect training diaries from the swimmers as some might stay out of training or not conform with the training prescribed from the coach. The swimmers were told that they were supposed to write what they had done and not what they were supposed to do, and that the diary was subject to professional secrecy.
Swimming PerformanceTime trials of 50m, 100m and 400m front crawl maximal swimming were conducted one to four days before VO2-tests in 25m pools. One subject from the control group was ill over several days just before the pre test, and three subjects from the intervention group were ill over several days just before the post test. For these subjects their best times were retrieved from their most recent personal records.
Bioenergetical ParametersThe swimming economy test consisted of four submaximal swims at increasing velocity, from 0.7 to 1.2 m·s-1, with increments of 0.05 or 0.1 m·s-1 depending on the development of the respiratory exchange ratio of the previous velocity. After the fourth workload, a swim test of four to six minutes with increasing intensity ending with a maximal effort was conducted to measure peak oxygen uptake in swimming (VO2peak). Maximal heart rate was monitored and recorded continuously using short range radio telemetry (Polar S610i, Polar Electro Oy, Kempele, Finland) and a telemetry antenna connected to the MetaMax II. Five strokes of the heart were added to the heart rate at the end of the VO2peak-test and considered their maximal swimming heart rate (HRmax). Both swimming economy and VO2peak was measured in a 25m pool. The term VO2peak was used as pilot investigations showed that common criteria (Åstrand et al., 2003) for a VO2max measurement were difficult to obtain in swimming. Especially, to reach a respiratory exchange ratio equal to or above 1.15. A specially designed breathing valve for swimming (Toussaint et al., 1987), modified to ensure a tighter fit of the apparatus to the head of the swimmer, was attached to the subjects (Kjendlie et al., 2003). Direct measurements of VO2 were made throughout the tests using a portable mixing chamber gas-analyzer system (Cortex MetaMax II, Cortex, Leipzig, Germany), which has formerly been found valid (Medbø et al., 2002). Respiratory parameters were averaged in sequences of 10 seconds, and expressed in the MetaSoft version 1.11.5 software (Cortex Biophysik GmbH). The valve with hoses (volume of 3.4 L each) was always placed in a vertical manner to ensure minimal water resistance. Working time for each submaximal trial was four to six minutes, the rest period between each trial was passive and a minimum of 30 seconds as the valve was very uncomfortable to wear and thus for them to have a break and stay focused and motivated. Swimming economy was defined as the average VO2 ·m-1 of the fourth and fifth 50m of the submaximal level, but if the VO2-values fluctuated with more than 2 mL·kg-1 · min-1 an additional 50m was included until stable values were obtained. A set of pace-lights controlled the swimming velocity. These lights consisted of 23 clusters of LED-lights attached to an A/D board (Arcom PCO24, Arcom Control Systems, Kansas City, USA) and a computer. Turn time and increased kick-off velocity was incorporated into the pattern of the moving lights. All work economy bouts were of aerobic intensity to assess a general work economy measure at a between-subject comparable velocity. The period from push-off at the beginning of each lap to hand touch at the end of each lap was also manually measured. To ensure that the measured velocity corresponded to the velocity of the center of mass, the reaching height of the swimmer was subtracted from the length of each lap. Swimming velocity was calculated as the product of pool length minus reaching height divided on time in seconds (m·s-1). During the VO2peak-measurement the pace-lights regulated the swimming velocity stepwise for the first 200m to 350m of the measurement, and the subject then further increased swimming velocity stepwise per 50m on their own initiative. The swimmers were instructed to push themselves as hard as they could for as long as they could towards the end of the VO2peak-measurement. All parameters related to intensity of swimming are proportional to velocity in the power of three (v3) as described by Kjendlie et al., 2004b. The v3 values were calculated and a regression line between VO2 - v3, R - v3 and VE - v3 were established for each subject. The values were entered into a scatter plot and the linear best fit line and appurtenant formula was calculated with Microsoft®Office Excel 2003 for every participant. These formulas were used to calculate the energy cost of swimming at 1.1 m·s-1 for every subject as this represents a feasible training velocity to all participants. R2-values were calculated for describing the gathering of the values around the regression line. To ensure true HRmax was found during the VO2peak-test, an additional protocol of 2 × 300m maximal front crawl swimming after 20-40 minutes of warm up was conducted measuring the heart rate at the end of each 300m. HRmax was considered the highest heart rate after the 300 meters. The variables further analysed were swimming economy, VO2peak with appurtenant ventilation, respiratory quotient and velocity, and the highest measured HRmax.
Kinematical ParametersStroke length, stroke rate and maximal velocity (vmax) were measured during 25 meter in water sprints. At least three maximal swims were recorded and the values of stroke length, stroke rate and vmax were extracted from the fastest of them. The rest period was minimum 2 minutes and the next bout started at the subject’s free will. The vmax value was calculated from the mean velocity of the stroke cycles from 12.5m to 25m except the last cycle in a 25m of maximal front crawl swimming. Variables were measured by a calibrated 100 Hz speedometer with a coefficient of variation of less than 2% (Pedersen and Kjendlie, 2006). The speedometer was connected to a computer and attached to the swimmer with a line spun around a wheel (circumference was 0.09m) specially turned to prevent toss. The wheel was connected to an incremental encoder (nr. IS630, Leine & Linde, Strängnes, Sweden), and connected to a digital encoding unit (DAQ 6024E data card, National Instruments, USA). The system was programmed by digital acquisition software (LabVIEW 7), and the signal was treated in MatLab (The MathWorks Inc., USA). At each stroke of the right hand a mark was registered for the software to calculate stroke length and stroke rate.
Force and Strength ParametersMaximal swimming force was attained from tethered maximal front crawl swimming until fatigue. Swimmers were allowed three attempts and the highest value was used. The rest period was minimum 2 minutes and the next bout started at the subject’s free will. A belt was attached to the waist of the swimmer and a rubber tube at the other end. The rubber tube was attached to a 100 Hz load cell (model TS C2, AEP, Modena, Italy) connected to a Digital Force Indicator display (AEP, Modena, Italy) which was also fastened to a pole on the pool deck in a horizontal position. A peak-hold function of the load cell was used to find the maximal peak force in front crawl swimming. A high reliability of this system has been reported (Kjendlie and Thorsvald, 2006). Maximal strength in bilateral shoulder extension (FL) was measured in a Technogym cable cross over apparatus (Technogym, Gambettola, Italy) from a starting position of 170 ± 10º shoulder flexion. During testing, subjects were not allowed to decrease the angle of the elbow joint below 90º, jerk the body backwards before the shoulder extension, reduce the angle in the hip joint below 170º or drop the elbow below the line between the shoulder joint and the wrist of the hand. All precautions were made to make the strength test as swim like as possible. Before the test the subjects were allowed a whole body warm up of 10-15 minutes on a treadmill or an ergometer cycle and a specific warm up with easier resistance in the apparatus with technical supervision. The test started at a resistance the subjects could easily carry out using a proper technique, and the load was increased by 2.5 kg at each attempt until failure. A maximal FL measurement was considered when subject could not fulfil an increased resistance in three attempts under given technical demands.
Statistical ProceduresMean and standard deviation were used for presentation of the findings. Since the control group included only two male subjects, data are presented and compared between whole groups and female part of the groups. All differences between the groups were calculated by a Mann-Whitney U test, and within group differences between pre and post test were calculated by a Wilcoxon matched-pairs signed-ranks test. Correlations were calculated by a Spearman rank-difference correlation. All calculations were performed using SPSS 13.0 for Windows. The level of significance was p < 0.05.
Swimming PerformanceThe 400m performance improved significantly (p < 0.05) in the intervention group, with no changes for the control group. The 50m (p = 0.11) and 100m (p = 0.12) performances did not significantly improve. Results are in Table 2. There was no significant difference between the swimming performance and the personal best records of the different groups.
Bioenergetical ParametersThere were no significant changes in swimming economy (p = 0.13 for the intervention group and 0.74 for the control group) or swimming economy scaled for body weight (p = 0.11 for intervention group and 0.68 for control group). There were no significant changes in VO2peak or VO2peak scaled for body weight for any group and the p > 0.60 in all groups. See Table 3 for further results. R2 from the slope in the VO2 - v3 used for calculating swimming economy showed a mean of 0.95 and 0.97 for the whole intervention group at pre and post test, respectively. Mean R2 for the whole control group was 0.94 and 0.88 at pre and post test, respectively.
Kinematical ParametersThere was no change of any kinematical parameter (Table 2).
Force and Strength ParametersA significant improvement of tethered swimming force in both the female intervention group (p < 0.05) and the whole intervention group (p < 0.05) was found (Table 2). No change was observed in the control group. In addition, tethered swimming force was significantly higher in the intervention group than in control group at post test (p < 0.05). FL improved in all groups, but was at post test significantly higher in the whole intervention group.
Relationships Between ParametersThere was a strong correlation between the change in the 400m performance and the change in tethered swimming force (rs = -0.975, p < 0.01) for the female intervention group. There were no differences between groups with regard to swimming distance covered in the training period (Table 4). 86% of the endurance intervention and 76% of the strength intervention was accomplished in the intervention group. This equals an average of 2.0 interval trainings and 1.8 strength trainings each week. There were no correlations between training volume or accomplishment of intervention and improved performances.
We hypothesized that a combined intervention of strength and endurance training would improve swimming force, maximal velocity, swimming economy, maximal oxygen uptake and swimming performance. The results show that both the whole and the female intervention group improved significantly in tethered swimming force (6.9% and 7.3% in whole and female, respectively), while no change occurred in the control group. All groups improved FL, but the whole intervention group gained statistically higher values than the control group at post test (p < 0.05). No significant change occurred in swimming economy or VO2peak in any group. As an improvement of strength due to strength training is consolidated, the improvement of dry-land strength occurred as expected in the intervention group (20.3% and 24.5% in whole and female, respectively). It is however surprising that the control group also improved land force significantly (11.8% and 9.3% in whole and female, respectively). There seem to be two liable explanations for this. The control group subjects might have been technically familiarized with the method from pre- to post-test, and/or the improvement is a response to the swimming or dry-land training they have brought out in the intervention period. The whole control group increased weight (Table 1) but not height (data not presented) which might have been a response to increased muscle mass and thus increased strength. A change of body composition was however not measured. The significantly higher post-test of the intervention group showed that an improvement from the strength intervention had occurred. The improvement of tethered swimming force was as expected, and in line with the study by Girold et al., 2007. The strong correlation between the improvement of the 400m freestyle performance time and the improvement of tethered swimming force in the female intervention group (rs = -0.975, p < 0.01) indicate that strength training seems important also for endurance performance in swimming. This indication is pointed out although the female intervention group did not significantly improve the 400m freestyle performance (p = 0.20) and the indication is thus built on incomplete evidence. However, leaning upon the results of Balabinis et al (Balabinis et al., 2003) and Hoff et al., 2002b our findings seem legitimate and the lack of consistency could probably be due to a low number of participants. Glancing at the findings in the light of clinical significance, any coach would appreciate an average improvement of 3.74 seconds in 400m freestyle. A possible explanation for the lack of correlation between strength and performance improvements is that the whole intervention group might have experienced a clustering when both genders were included. The males improved relatively little in land strength but much in swimming and the females vice versa. However, our findings should be investigated further to develop a clearer picture of the correlation between strength and swimming performance. Previous studies have shown that muscle force capabilities such as stroke force during swimming (Hawley and Williams, 1991), biokinetic stroke power on a swim bench (Sharp et al., 1982) and Wingate anaerobic arm test (Toussaint and Vervoorn, 1990) correlates more strongly with sprint performances than 400m performance and that strength training improves swimming performance (Girold et al., 2006; 2007). Improvements of 50m (p = 0.11) or 100m (p = 0.13) performance or both might have been expected. Although the groups were small and the p-values were low, our findings were not statistically significant. The lack of improvement in vmax, 50m or 100m performance might be observed in relation to the lack of improvements in stroke length and stroke rate which have previously been reported to improve simultaneously (Toussaint and Vervoorn, 1990). It may therefore be suggested that strength training should be followed up in parallel with technical training to improve technical performance, and to facilitate transfer of land strength gains. It should be mentioned that one subject from the intervention group performed a 0.76 seconds faster 50m within the intervention period and another 0.51 second faster five days after the post tests than their post test performances. If these best times were used in the statistics, the improvement would also be significant (p < 0.05) for the 50m. As the 400m is of a lower velocity, the impact of a missed turn, a slow start, etc. will not affect the final time to such an extent as in the shorter events. Thus, it can be hypothesized that an increased number of participants might have improved our chance of finding significant improvements. And again, the importance of looking at the clinical significance is underlined. It could be questioned whether the swimming performance tests were representative for their optimal performance level when mainly choosing to measure it outside competition. As there was no statistical difference between performance level and personal best times, we believe the performance times are reliable and valid for the groups. The lack of improvement in swimming economy might also be seen in relationship with the lack of change in stroke rate and stroke length, although the p-value in the whole intervention group might show a slight tendency toward significance for the swimming economy (p = 0.13 and p = 0.11 scaled for body weight). It has been shown that manipulation of stroke length or stroke rate may improve swimming economy (Barbosa et al., 2008). Previous studies have observed improvements of work economy in cross-country skiing (Hoff et al., 2002a; Østerås et al., 2002) as a consequence of maximal strength training, and these improvements have been explained by neural adaptation. Although a favourable neural adaptation might have occurred in the actual muscles and muscle groups in this study as subjects were stronger without increasing body weight, there would subsequently be a need for the subject to transfer these capacities into improved stroke length or stroke rate or both (Wakayoshi et al., 1995), and no changes of these parameters were observed. It would be interesting to investigate the nature of these improvements. An electromyographic investigation has successfully been applied to swimming before (Rouard et al., 1990), and a similar method could possibly unravel some of the improvements we have observed. Despite the somewhat large improvements of VO2max found in other studies employing a similar endurance interval training intervention (Helgerud et al., 2007; Hoff et al., 2002b) no improvement of VO2peak was observed. One of the plausible explanations for this is regarding the method of the VO2peak-measurements. There are different methods for measuring oxygen uptake kinetics during swimming but when comparing these it still remains unclear which is the better (Rinehardt et al., 1991). This challenge may be one of the reasons why very few studies have been published on the development of VO2max in swimming. The setup used to measure VO2peak has been described earlier (Kjendlie et al., 2004a; 2003), but instead of Douglas bags for sampling of gas, a Cortex MetaMaxII-analyzer was used. Although the Douglas-bag-method remains the gold standard for testing of oxygen uptake kinetics, the Cortex MetaMaxII-analyzer has been found valid although it is reported to overestimate VO2 by 4% (Medbø et al., 2002), and allows for continuous surveillance of the oxygen uptake kinetics which necessarily requires a breathing valve. The arguments against the use of methods involving a breathing valve are that it is uncomfortable, time-consuming ant that it may affect VO2max (Montpetit et al., 1981). However, the breathing valve has been found not to cause any alterations in swimming technique (Kjendlie et al., 2003). Thus, every effort was made to ensure a reliable measurement, although we recognize that there still are challenges within the measuring of oxygen uptake kinetics in swimming. In support of our method, the same procedure was performed at pre- and post-test, and neither group changed in VO2peak or velocity at VO2peak. Another more likely explanation is that an average of two interval-sessions a week is an insufficient stimulus to produce detectable training adaptations in competitive swimmers. The already mentioned former intervention studies of 8 weeks (Helgerud et al., 2007; Hoff et al., 2002b) gave 7.2% and 10.8% improvements of VO2max in male junior soccer-players at pre-season and young male adults of average training level. However, our intervention group was different from those of these studies. The groups consisted of competitive swimmers with already very high training volumes and in the middle of the competitive season. The composition regarding gender differences and age was a result of few adequately skilled swimmers in the area to recruit from, and subsequently withdrawals resulting in a control group with only two male participants. The tendency towards heterogeneity of the intervention and control group is one of the weaknesses of this study. However, there were no significant differences between groups at baseline regarding age or anthropometrics, freestyle is commonly the most applied stroke during swimming practice disregarding specialty and the only modification of regular practice was the training interventions. Further a larger part of the control group consisted of freestyle specialists and the seemingly (but unsignificant) homogenously younger control group will traditionally be more susceptible to exercise. Thus, the presented within and between group changes is considered to be strongly related to the intervention. A third explanation might lie in the participant’s conformity with the prescribed training intervention. Although the intention of the interval training sessions was thoroughly explained to the intervention subjects and heart rates surveillanced, there was a possibility that the participants did not keep within the desired heart rate zone at all times as subscribed. This might have reduced the potential effect of the interventions. Due to illness we could unfortunately not assess swimming time trials for all participants at the same times, but carried out the other tests. This is unlikely to have compromised the short-time measurements such as tethered swimming force, land force, vmax, stroke length or stroke rate rate as these do not stress the energy-demanding oxygen transport system, but might have blunted the oxygen uptake measurements, especially VO2peak. The high training volume of the included swimmers and the study being carried out in the middle of the season are both considered strengths of this study. As special attention was paid to the intervention group, a Hawthorne-effect might have occurred. The phenomenon is explained as a short-term improvement caused by observing worker performance (Wickström and Bendix, 2000). However, the study duration of 11 weeks is considered more than short-term, and the effects are thus considered unlikely to influence our findings. In summary, it is adjacent to believe that the lack of improvement in VO2peak is related to a combination of merely two weekly aerobic interval sessions, swimmers already being at a high aerobic level and training volume, and maybe the swimmer’s heart rate consistency as described. Few qualified and available subjects made it difficult to make more than two groups, and physiological and pragmatic considerations suggested it possible and desirable to combine the interventions and still being able to separate the hypothetical findings. In the light of hindsight, maybe we should have applied only one intervention for a cleaner result. But on the other side, our study supplies information on the combination of interventions not available before. Future studies should look further into the mechanisms of the improvements of strength training and try to find methods to impose the increased swimming force on improved swimming biomechanics. There seem to be benefits from maximal strength training, and two weekly sessions seems to be sufficient to increase strength and positively influence swimming performance. Although VO2peak did not change, there are no indications that strength training has influenced endurance performance negatively. Rather the opposite as an improvement of 400m freestyle could be explained by increased tethered swimming force. In support of this conclusion Gullstrand and Holmér, 1983 concluded that VO2max in high performance swimmers is of less importance than other physiological factors, for example swimming force. To illuminate this matter further, a study on the strengths of correlations of well-known parameters affecting swimming performance should be brought out.
Two weekly sessions of maximal strength training is sufficient to improve maximal tethered swimming force in front crawl swimming. Improved tethered swimming force was correlated with improved 400m freestyle and it is thus concluded that strength training might be important for improving middle distance swimming. Adding two weekly sessions of high-intensity interval training to a high volume training situation was not enough to improve VO2peak in swimming.
ACKNOWLEDGEMENTS |
We thank Sør-Trøndelag Swimming District for financial support, and to Heimdal High School and Rune Heen for providing time, access and patience during the intervention. We appreciate the help of Kristian Thorsvald in datacollection. |
|
AUTHOR BIOGRAPHY |
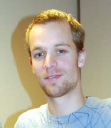 |
Stian Aspenes |
Employment: PhD student. |
Degree: Master of Science |
Research interests: Fitness in epidemiology, swimming performance, endurance training, female soccer performance. |
E-mail: stian.aspenes@ntnu.no |
|
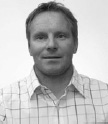 |
Per-Ludvik Kjendlie |
Employment: Associate Professor. |
Degree: PhD |
Research interests: Swimming research, work economy, swimming biomechanics. |
E-mail: per-ludvik.kjendlie@nih.no |
|
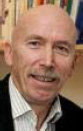 |
Jan Hoff |
Employment: Professor |
Degree: PhD |
Research interests: Strength training, endurance training, COPD, soccer physiology. |
E-mail: jan.hoff@ntnu.no |
|
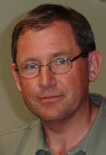 |
Jan Helgerud |
Employment: Professor |
Degree: PhD |
Research interests: Exercise physiology. Skeletal muscle blood flow and metabolism. Pulmonary gas exchange. Applied sport physiology. |
E-mail: jan.helgerud@ntnu.no |
|
|
|
REFERENCES |
 Åstrand P.O., Rodahl K., Dahl H.A., Strømme S.B. (2003) Textbook of work physiology. Champaign. Human Kinetics.
|
 Østerås H., Helgerud J., Hoff J. (2002) Maximal strength-training effects on force-velocity and force-power relationships explain increases in aerobic performance in humans. European Journal of Applied Physiology 88, 255-263.
|
 Balabinis C.P., Psarakis C.H., Moukas M., Vassiliou M.P., Behrakis P.K. (2003) Early phase changes by concurrent endurance and strength training. Journal of Strength and Conditioning Research 17, 393-401.
|
 Barbosa T.M., Fernandes R.J., Keskinen K.L., Vilas-Boas J.P. (2008) The influence of stroke mechanics into energy cost of elite swimmers. European Journal of Applied Physiology 103, 139-149.
|
 Barzdukas A., Franciosi P., Trappe S., Letner C., Troup J.P., Maclaren, D., Reilly T., Lees A. (1992) Biomechanics and Medicine in Swimming VI. Adaptations to interval training at common intensities and different work: Rest ratios. Cambridge. E & FN Spon.
|
 Costill D.L., Flynn M.G., Kirwan J.P., Houmard J.A., Mitchell J.B., Thomas R., Park S.H. (1988) Effects of repeated days of intensified training on muscle glycogen and swimming performance. Medicine and Science in Sports and Exercise 20, 249-254.
|
 Costill D.L., Thomas R., Robergs R.A., Pascoe D., Lambert C., Barr S., Fink W.J. (1991) Adaptations to swimming training: Influence of training volume. Medicine and Science in Sports and Exercise 23, 371-377.
|
 D’Acquisto L.J., Barzdukas A., Dursthoff P., Letner C., Troup J.P., Maclaren, D., Reilly T., Lees A. (1992) Biomechanics and Medicine in Swimming VI. Physiological adaptations to 60 vs 20 minutes of swim training at 76% VO2max. Cambridge. E & FN Spon.
|
 Faude O., Meyer T., Scharhag J., Weins F., Urhausen A., Kindermann W. (2008) Volume vs. intensity in the training of competitive swimmers. International Journal of Sports Medicine 29, 906-912.
|
 Gergley T.J., McArdle W.D., DeJesus P., Toner M.M., Jacobowitz S., Spina R.J. (1984) Specificity of arm training on aerobic power during swimming and running. Medicine and Science in Sports and Exercise 16, 349-354.
|
 Girold S., Calmels P., Maurin D., Milhau N., Chatard J.C. (2006) Assisted and resisted sprint training in swimming. Journal of Strength and Conditioning Research 20, 547-554.
|
 Girold S., Maurin D., Dugue B., Chatard J.C., Millet G. (2007) Effects of dry-land vs. Resisted- and assisted-sprint exercises on swimming sprint performances. Journal of Strength and Conditioning Research 21, 599-605.
|
 Gullstrand L., Holmér I., Hollander, A.P., Huijing, P.A., De Groot, G. (1983) Biomechanics and Medicine in Swimming IV. Physiological characteristics of champion swimmers during a five-year follow-up period. Champaign, Illinois. Human Kinetics Publishers.
|
 Hawley J.A., Williams M.M. (1991) Relationship between upper body anaerobic power and freestyle swimming performance. International Journal of Sports Medicine 12, 1-5.
|
 Helgerud J., Høydal K., Wang E., Karlsen T., Berg P., Bjerkaas M., Simonsen T., Helgesen C., Hjorth N., Bach R., Hoff J. (2007) Aerobic high-intensity intervals improve VO2max more than moderate training. Medicine and Science in Sports and Exercise 39, 665-671.
|
 Hoff J., Gran A., Helgerud J. (2002a) Maximal strength training improves aerobic endurance performance. Scandinavian Journal of Medicine and Science in Sports 12, 288-295.
|
 Hoff J., Wisløff U., Engen L.C., Kemi O.J., Helgerud J. (2002b) Soccer specific aerobic endurance training. British Journal of Sports Medicine 36, 218-221.
|
 Houston M.E., Wilson D.M., Green H.J., Thomson J.A., Ranney D.A. (1981) Physiological and muscle enzyme adaptations to two different intensities of swim training. European Journal of Applied Physiology and Occupational Physiology 46, 283-291.
|
 Kirwan J.P., Costill D.L., Flynn M.G., Mitchell J.B., Fink W.J., Neufer P.D., Houmard J.A. (1988) Physiological responses to successive days of intense training in competitive swimmers. Medicine and Science in Sports and Exercise 20, 255-259.
|
 Kjendlie P.L., Ingjer F., Madsen Ø., Stallman R.K., Stray-Gundersen J. (2004a) Differences in the energy cost between children and adults during front crawl swimming. European Journal of Applied Physiology 91, 473-480.
|
 Kjendlie P.L., Ingjer F., Stallman R.K., Stray-Gundersen J. (2004b) Factors affecting swimming economy in children and adults. European Journal of Applied Physiology 93, 65-74.
|
 Kjendlie P.L., Stallman R.K., Stray-Gundersen J., Chatard, J. C. (2003) Biomechanics and Medicine in Swimming IX. Influences of a breathing valve on swimming technique. Saint-Etienne. University of Saint-Etienne.
|
 Kjendlie P.L., Thorsvald K. (2006) A tethered swimming power test is highly reliable. Portuguese Journal of Sports Science 6, 91-92.
|
 Konstantaki M., Winter E.M., Swaine I.L., Keskinen, K. L., Komi, P. V., Hollander, A.P. (1999) Biomechanics and Medicine in Swimming VIII. The effects of arms- or legs-only training on indices of swimming performance and dry-land endurance in swimmers. Jyväskylä. University of Jyväskylä.
|
 Magel J.R., Faulkner J.A. (1967) Maximum oxygen uptakes of college swimmers. Journal of Applied Physiology 22, 929-933.
|
 Magel J.R., Foglia G.F., McArdle W.D., Gutin B., Pechar G.S., Katch F.I. (1975) Specificity of swim training on maximum oxygen uptake. Journal of Applied Physiology 38, 151-155.
|
 Medbø J.I., Mamen A., Welde B., von Heimburg E., Stokke R. (2002) Examination of the metamax i and ii oxygen analysers during exercise studies in the laboratory. Scandinavian Journal of Clinical and Laboratory Investigation 62, 585-598.
|
 Miyashita M., Kanehisa H., Hollander, A. P., Huijing P.A., De Groot, G. (1983) Biomechanics and Medicine in Swimming IV. Effects of isokinetic, isotonic and swim training on swimming performance. Champaign, Illinois. Human Kinetics Publishers.
|
 Montpetit R.R., Leger L.A., Lavoie J.M., Cazorla G. (1981) VO2 peak during free swimming using the backward extrapolation of the O2 recovery curve. European Journal of Applied Physiology and Occupational Physiology 47, 385-391.
|
 O’Toole M., Shephard, R.J., Åstrand, P.O. (2000) Endurance in sport. Endurance training for women. Cornwall. Blackwell Science.
|
 Pate R.R., Kriska A. (1984) Physiological basis of the sex difference in cardiorespiratory endurance. Sports Medicine 1, 87-98.
|
 Pedersen T., Kjendlie P.L. (2006) The effect of the breathing action on velocity in front crawl sprinting. Portuguese Journal of Sport Science 6, 75-77.
|
 Petersen S.R., Miller G.D., Wenger H.A., Quinney H.A. (1984) The acquisition of muscular strength: The influence of training velocity and initial vo2 max. Canadian Journal of Applied Sport Sciences 9, 176-180.
|
 Rinehardt K.F., Kraemer R.R., Gormely S., Colan S. (1991) Comparison of maximal oxygen uptakes from the tethered, the 183- and 457-meter unimpeded supramaximal freestyle swims. International Journal of Sports Medicine 12, 6-9.
|
 Rouard A.H., Quezel G., Billat R.P., Maclaren, D., Reilly, T., Lees, A. (1990) Biomechanics and Medicine in Swimming VI. Effects of speed on emg and kinematic parameters in freestyle. E … FN Spon.
|
 Rouard A.H., Quezel G., Billat R.P., Maclaren, D., Reilly, T., Lees, A. (1992) Biomechanics and Medicine in Swimming VI. Effects of speed on emg and kinematic parameters in freestyle. Cambridge. E … FN Spon.
|
 Sale D.G., Komi, P. V. (2003) Strength and power in sport. Neural adaptation to strength training. Oxford. Blackwell Publishing.
|
 Sharp R.L., Troup J.P., Costill D.L. (1982) Relationship between power and sprint freestyle swimming. Medicine and Science in Sports and Exercise 14, 53-56.
|
 Silva A.J., Costa A.M., Oliveira P.M., Reis V.M., Saavedra J., Perl J., Rouboa A., Marinho D.A. (2007) The use of network technology to model swimming performance. Journal of Sports Science and Medicine 6, 117-125.
|
 Tanaka H., Costill D., Thomas R., Fink W.J., Widrick J.J. (1998) Impact of resistance training on endurance performance. A new form of cross-training?. Sports Medicine 25, 191-200.
|
 Tanaka H., Costill D.L., Thomas R., Fink W.J., Widrick J.J. (1993) Dry-land resistance training for competitive swimming. Medicine and Science in Sports and Exercise 25, 952-959.
|
 Toussaint H.M., Meulemans A., de Groot G., Hollander A.P., Schreurs A.W., Vervoorn K. (1987) Respiratory valve for oxygen uptake measurements during swimming. European Journal of Applied Physiology and Occupational Physiology 56, 363-366.
|
 Toussaint H.M., Vervoorn K. (1990) Effects of specific high resistance training in the water on competitive swimmers. International Journal of Sports Medicine 11, 228-233.
|
 Trappe S., Pearson D.R. (1994) Effects of weigth assisted dry-land strength training on swimming performance. Journal of Strength and Conditioning Research 08, 209-213.
|
 Wakayoshi K., D’Acquisto L.J., Cappaert J.M., Troup J.P. (1995) Relationship between oxygen uptake, stroke rate and swimming velocity in competitive swimming. International Journal of Sports Medicine 16, 19-23.
|
 Wickström G., Bendix T. (2000) The "Hawthorne effect"-what did the original hawthorne studies actually show?. Scandinavian Journal of Work, Environment and Health 26, 363-367.
|
 Yamamoto L.M., Lopez R.M., Klau J.F., Casa D.J., Kraemer W.J., Maresh C.M. (2008) The effects of resistance training on endurance distance running performance among highly trained runners: A systematic review. Journal of Strength and Conditioning Research 22, 2036-2044.
|
|
|
|
|
|
|