|
|
|
ABSTRACT |
The aim of the present study was to evaluate the effects of 3 weeks altitude training according to the HiHiLo (live high-base train high-interval train low) procedure as described by Chapman et al. (1998), on erythropoiesis, maximal oxygen uptake and energy cost of exercise under normoxia in elite biathletes. Fifteen male elite biathletes randomly divided into an experimental (H) group (n = 7; age 27.1 ± 4.6 years; maximal oxygen uptake (VO2max) 66.9 ± 3.3 ml·kg–1·min–1; body height (BH) 1.81 ± 0.06 m; body mass (BM) 73.1 ± 5.4kg), and a control (C) group (n = 8; age 23.2 ± 0.9 years; VO2max 68.2 ± 4.1 ml·kg–1·min–1; BH 1.75 ± 0.03 m; BM 63.1 ± 1.5 kg) took part in the study. The H group stayed for 3 weeks at an altitude of 2015 m and performed endurance training on skis four times per week at 3000 m. Additionally, the training protocol included three high-intensity interval sessions at an altitude of 1000 m. The C group followed the same training protocol with skirollers in normoxia at an altitude of 600 m. The HiHiLo protocol applied in our study did not change VO2max or maximal workload (WRmax) significantly during the incremental treadmill test in group H. However, the energy cost for selected submaximal workloads in group H was significantly (p < 0.01) reduced compared to group C (-5.7%, -4.4%, -6% vs. -3.5%, -2.1%, -2.4%). Also a significant (p < 0.001) increase in serum EPO levels during the first two weeks of HiHiLo training at 2015 m was observed, associated with a significant (p < 0.05) increase in hemoglobin mass, number of erythrocytes, hematocrit value and percent of reticulocytes compared with initial values (by 6.4%, 5%, 4.6% and 16,6%, respectively). In group C, changes in these variables were not observed. These positive changes observed in our study led to a conclusion that the HiHiLo training method could improve endurance in normoxia, since most of the biathlon competitions are performed at submaximal intensities. |
Key words:
Altitude training, erythropoiesis, aerobic capacity, biathlon
|
Key
Points
- The observed results suggests that the 3-weeks HiHiLo protocol is an effective training means for improving energy cost during submaximal exercise at sea level.
- The 3-weeks HiHiLo protocol increased the rate of erythropoiesis and improved most haematological variables.
- However, the positive changes in the athletes haematological variables after the HiHiLo protocol did not contribute to the improvement of VO2max values.
|
For the first time altitude training was applied in the training process almost fifty years ago. To make it more effective, its methodology has been constantly modified. As a result three concepts of high-altitude training have been accepted: live high-train high (LH-TH), live high-train low (LH-TL) and intermittent hypoxic training (IHT). Each of them combines the effects of acclimatization and different training procedures, what influences adaptation and performance (Levine and Stray-Gundersen, 1997; Wilber et al., 2007; Czuba et al., 2011). For example, the main factor limiting the effectiveness of the LH-TH concept is that many athletes cannot maintain high training intensity while staying at an altitude for a longer period of time and consequently decrease their level of endurance (Wilber et al., 2007). To overcome this problem Levine and Stray-Gundersen (1997) introduced the LH-TL method. This approach assumes that athletes should live at medium altitudes (2000-3000 m) in order to improve blood oxygen capacity, whereas training itself should be performed at an altitude not greater than 1200 m. This method allowed athletes to improve their blood oxygen capacity, while maintaining high training intensity (Wilber et al., 2007). Recently, significant attention in sport sciences has been given to IHT, which theoretically, may cause more pronounced adaptive changes in muscle tissues in comparison to traditional endurance training under normoxic conditions (Czuba et al., 2013). Improvement in sea-level performance and increased maximal oxygen uptake (VO2max) after IHT cannot be explained by changes in blood variables alone, but are also associated with non-hematological adaptive mechanisms (Czuba et al., 2011). Recently, Millet et al. (2010) introduced the possibility of combining different hypoxic training methods including intermittent hypoxic exposure at rest (IHE) during interval –training (IHIT) or LH-TL associated with IHT (LHTLH). Whereas reports on the impact of IHT on enchased glycolysis and buffering capacity (Gore at al., 2007), scientists have also discussed the potential benefits of this method for anaerobic performance. Therefore more recently, Millet at al. (2013) based predominantly on different mechanisms subdivided the IHT methods to: continuous low intensity (<70% VO2max) and long duration (>30 minut) exercise in hypoxia – CHT, interval training in hypoxia – IHT and repeated sprint training in hypoxia – RSH. The basic mechanism improving VO2max and thereby exercise capacity during both LH-TH and LH-TL training concepts is the stimulation of erythropoiesis, which results in increased blood oxygen capacity (Levine and Stray-Gundersen, 1997; Melissa et al., 1997). Another major factor improving exercise capacity after LH-TL training has been attributed to LL-TH-induced greater buffering capacity of muscle tissue (Gore et al., 2001) and/or to a decrease in exercise energy cost (Saunders et al., 2004a). The two mechanisms were also used to explain why exercise capacity increases after either LL-TH or IHT training, when its application did not lead to improved blood oxygen capacity (Czuba et al., 2011; Katayama et al., 2004; Rodriguez et al., 2004; Roels et al., 2005; 2007). However, there is a consensus in the literature that IHT training can improve exercise capacity by reducing exercise energy cost (Bonnetti et al., 2009; Czuba et al., 2011), increasing muscle tissue buffering capacity (Gore et al., 2001) and stimulating the activity of glycolytic enzymes (Katayama et al., 2004). Available data with regard to effects of altitude training on the energy cost of working muscles are unequivocal (Green et al., 2000; Gore et al., 2001; Levine and Stray-Gundersen, 1997; Svedenhag et al., 1991; Saltin et al. 1995). The LH-TL training has been found not to reduce the energy cost in normoxia (Levine and Stray-Gundersen, 1997; Telford et al., 1996), but in several studies (Gore et al., 2001; Katayama et al., 2003; Marconi et al., 2005; Neya et al., 2007; Saunders et al., 2004b; Schmidt et al. 2006) significant reductions in exercise energy cost after various procedures of altitude training were registered. Recently, Lundby et al. (2007) observed that submaximal VO2 is unchanged during and 2-3 days after chronic exposure from moderate altitude (2500-3000 m) up to altitudes of 4300 m. However, in the study of Schmitt et al. (2006) energy cost of exercise did not change immediately after LH-TL protocol but 15 days later was reduced in the non-specific activity. In specific activity this improvement was observed in both groups (LH-TL and control). The main reason of these discrepancies may be ascribed to different methodological approaches adopted by researchers, different sports level and genetic predispositions of subjects, as well as the level of hypoxia (altitude) and time of exposure (Chapman et al., 2014; Gore et al., 2013) Another significant factor to consider is the type of hypoxia used, because physiological adaptation to hypobaric vs. normobaric hypoxia is different, particularly with regard to lung fluid regulation (Millet et al., 2012). Bonetti and Hopkins (2009) reported in their meta-analysis that the LHTL protocol in natural conditions (hypobaric hypoxia) currently provides the best stimulus for enhancing endurance performance in elite and sub elite athletes, while some artificial protocols (normobaric hypoxia) are effective only in sub elite athletes. This may also explain why attempts at making the above methods more effective are being constantly made. One of the more interesting modifications in the LH-TL protocol was proposed by Chapman et al. (1998). According to the new solution, athletes should stay and perform low-intensity training at an altitude of 2500 m for four weeks, while high-intensity interval training should be performed at 1250 m. This method is known as HiHiLo (live high-base train high-interval train low). Stray-Gundersen et al. (2001) found a significant 3 % increase in VO2max accompanied by improvement of several hematological variables (hemoglobin mass and hematocrit) that resulted in significant improvement of running time (5.8 s, 3 km race) after the HiHiLo protocol. Therefore, one can assume that the HiHiLo protocol may be an effective training method, particularly applicable to cross-country skiers and biathletes during the preparatory period. Many winter sports athletes, in order to perform specific training on ice or snow during the preparatory period travel to the mountains and stay at high altitude. These procedures have been adopted by biathletes and cross-country skiers. The described above version of HiHiLo training is still not used frequently and reports on its effectiveness are limited. In particular, there are no data regarding the effects of HiHiLo training and a reduced energy cost of exercise in normoxia. Thus, we investigated if the HiHiLo procedure performed in hypobaric hypoxia conditions affects biathletes’ aerobic capacity and exercise energy cost in normoxia. Additionally, erythropoietin and selected hematological variables were evaluated, as potential factors influencing aerobic capacity and endurance performance.
ParticipantsFifteen highly-trained biathletes participated in the study. The basic anthropometric data of the athletes (body height- BH, body mass-BM, % of fat - FAT, fat-free mass-FFM) and their training experience are presented in Table 1. All subjects held valid medical examination certificates and had no contraindications for participating in the experiment. The study design was approved by the Bioethics Committee for Scientific Research at the Academy of Physical Education in Katowice.
Experimental designThe selected biathletes were randomly divided into experimental (H) and control (C) groups. The experiment was carried out in the middle of the preparatory period of the annual training cycle (September-October). The H group trained in hypobaric hypoxia for three weeks (3 microcycles) in natural settings (Glaitner Hochjoch, Italy) according to the HiHiLo procedure as described by Chapman et al. (1998), whereas their control counterparts trained in normoxic conditions (~600 m, Duszniki Zdroj, Poland). The athletes from the H group beside the LH-TL procedure performed specific aerobic endurance training in hypoxia. Both groups of athletes performed the same training protocol with respect to relative training workloads. The participants from the H group stayed at an altitude of 2015 m and performed aerobic endurance training on skis (70-85 % of threshold heart rate - HRLT; ~130-150 bpm) four times in each microcycle at 3000 m (12 altogether). The total training volume in hypoxia in the group H was 30 hours. Each microcycle included three sessions of high-intensity interval training (95-105% HRLT; ~170-180 bpm) intended to increase the athletes’ strength endurance, during which they skirolled at an altitude of 1000 m (19 h) and then returned to 2015 m. The total training volume in normoxia in the H group equaled 18 hours. The subjects in the group C followed the same training protocol with skirollers in normoxia. The total training volume in normoxia in the group C was 49 h.
Exercise protocolBefore the altitude training began all subjects underwent the first series of examinations (S1). The experimental training protocol consisted of three microcycles involving incremental exercise and a one-week regeneration period, followed by the second series of laboratory evaluations (S2). The exercise test started with a speed of 6 km·h–1 that was increased by 2 km·h–1 every 3 minutes until the speed reached 14 km·h–1. Exercise intensity was then increased by adjusting treadmill incline by 2.5% every 3 minutes until subject’s volitional exhaustion. To make the interpretation of the results easier, exercise intensity was also expressed in watts (W), as calculated by the MetaSoft software (Cortex, Germany). If a subject terminated the test before completing a given workload, then the maximum workload was calculated from the formula WRmax = WRk + (t/T x WRp) (Kuipers et al. 1985), where WRk – previous workload, t – exercise duration with the workload until premature failure, T – the duration of each workload, WRp – the amount of workload by which exercise intensity increased during the test. The heart rate (HR), minute ventilation (VE), breathing frequency (BF), oxygen uptake (VO2) and carbon dioxide content in expired air (VCO2) were recorded in the subjects at rest and during the exercise protocol with the MetaMax 3B gas analyser (Cortex, Germany). Fingertip capillary blood samples for the assessment of lactate (LA) concentration (Biosen C-line Clinic, EKF-diagnostic GmbH, Germany) were drawn at rest and at the end of each step of the test, as well as during the 3rd, 6th, 9th, and 12th min of recovery. The lactate threshold was determined by the D-max method (Cheng et al., 1992). Our earlier study (Czuba et al. 2009) demonstrated that LT determined by the D-max method corresponded to the maximal lactate steady state (MLSS). Additionally, capillary rest and post-exercise blood samples were used to determine acid-base equilibrium and oxygen saturation of hemoglobin (RapidLab 248, Bayer Diagnostics, Germany).
Blood collectionThe antecubital venous and capillary blood samples were collected to estimate the levels of selected physiological variables before and after treadmill exercise tests to voluntary exhaustion. Fasting blood samples were collected in heparin-treated tubes and used for hormonal (erythropoietin) analyses. Blood plasma and serum were separated using routine procedures, then processed immediately and kept frozen at -80° C until analyses. Both S1 and S2 started before breakfast after an overnight fast to collect resting blood samples (5ml). The samples were tested immediately afterwards to assess blood morphology with concentration of reticulocytes (Advia 2120i, Siemens, Germany) and blood ferritin levels (IMMULITE 1000, Siemens, Germany). Serum EPO levels were determined by means of in vitro chemiluminescence with the IMMULITE 1000 analyzer (Siemens, Germany). After blood samples collection, body height, mass and composition were evaluated in all subjects. Body composition was analyzed by bioelectrical impedance (InBody 220, Biospace, Korea). Three hours after a light mixed meal (50% CHO, 30% fat, 20% Pro), the subjects performed an incremental exercise test on a mechanical treadmill (Pulsar HP, Cosmos, Germany), during which their VO2max and lactate thresholds were measured.
Blood testing during the HiHiLo protocolIn the course of the HiHiLo protocol, venous blood samples (5 ml) were taken several times from the antecubital vein of the subjects from the experimental group (H) to determine their blood morphology and serum EPO levels. Blood was sampled in the first days of the subjects’ exposure to hypobaric hypoxia (after 24 and 72 h), as well as after each week of altitude training. Blood sampling days in successive weeks were always preceded by a day of active rest. The only exception was blood sampling on the first and third day of exposure to altitude (after 24 and 72 h), during which the subjects performed light aerobic training.
Statistical analysisThe obtained data were analyzed statistically with the use of Statistica 10.0 (StatSoft). Basic descriptive statistics were calculated, and all variables were examined for normal distribution. To determine the influence of the HiHiLo protocol on chosen physiological and biochemical variables during two series of examinations in laboratory (S1 and S2), the two-way ANOVA (group & training) with repeated measures was applied. Additionally, to examine the influence of hypobaric hypoxia during the HiHiLo protocol on serum EPO levels and saturation of hemoglobin values (SpO2) in the experimental group (H), the one-way ANOVA with repeated measures was used. When significant differences in the F ratio were found, the post hoc Tukey’s test was used. The level of statistical significance was set at p < 0.05.
Table 2 contains the mean values, standard deviations and statistically significant differences in body mass and body composition of subjects in both groups participating in the two series of examinations (S1 and S2). Table 3 provides the mean values of maximal workloads (WRmax) and threshold workloads (WRLT), as well as selected cardiorespiratory variables obtained during the incremental tests performed before and after the experiment, and the significance of differences registered between successive examinations. Table 4 shows oxygen uptake values (VO2) for submaximal workloads recorded in S1 and S2 incremental tests and the statistical significance of differences between them. Finally, Table 5 shows the values of selected haematological indicators and serum ferritin levels recorded during the experiment along with the significance levels of the differences. A two-factor analysis of variance in the group × training interaction showed significant differences in the subjects’ body mass (F = 28.195, p < 0.001) and BMI (F = 14.032, p < 0.01) values, as well as in the percentage of fat tissue (%FAT) (F = 6.321, p < 0.05). Other significant differences were found in the absolute (F = 24.84; p < 0.001) and relative workloads at lactate threshold (WRLT; F = 21.43, p < 0.001), in the maximum respiratory exchange ratio (RERmax; F = 32.50, p < 0.001), and in the relative oxygen uptake at lactate threshold (VO2LT; F = 5.764, p < 0.05). The analysis of the group × training interaction also revealed significantly different oxygen uptake levels (VO2) recorded in the first series of examinations (S1) for selected submaximal workloads in the incremental test (VO2 -1LT – VO2 at one workload under LT, F = 5.14, p < 0.05; VO2 LT – VO2 at LT workload, F = 7.59, p < 0.01; VO2 +1LT – VO2 at one workload above LT, F = 8.85; p < 0.01), as well as significant differences in the increases in blood lactate concentration (∆LA; F = 6.411; p < 0.05), in blood pH changes (∆pH, F = 8.402; p < 0.05), and in blood base deficit values (∆BE; F = 5.131; p < 0.05) during S1 and S2 incremental tests. The number of erythrocytes (RBC; F = 37.302, p < 0.001), haemoglobin concentration (HGB; F = 51.992, p < 0.001), haematocrit values (HCT; F = 22.154, p < 0.001) and percent of reticulocytes (F = 5.587, p < 0.05) were also significantly different. A one-factor analysis of variance in the training interaction in the H group showed significant differences in serum EPO levels (F = 22.097, p < 0.001) and SpO2 values (F = 75.010, p < 0.001) during the HiHiLo protocol. The results of ANOVA indicate significant differences between groups, yet they do not indentify between which groups, thus post-hoc test were used to determine these differences. In the next stage of data analysis, the post-hoc Tukey’s test was applied. According to the results, in the group H, the subjects fat mass (FM) and fat content (FAT%) decreased significantly (p < 0.05) by 7.7% and 7.6% respectively compared to baseline measurements (Table 1). The reduction of fat mass significantly (p < 0.05) decreased body mass of the tested subjects (Table 1). Furthermore, this group of athletes significantly (p < 0.01) increased absolute and relative values of WRLT (by 4% and 5.4%, respectively) and relative values of VO2LT (by 3.6%; p < 0.05), and registered a statistically significant lower RERmax (by 3.6%; p < 0.01). In the group C, changes in these variables were not observed. In the group H, the ∆pH, ∆BE, and ∆LA values decreased significantly (by 16.6%, 9.6%, respectively; p < 0.05), but in the group C ∆ pH rose significantly (by 2.16%; p < 0.05) (Figure 1 and Figure 2). The athletes from the group H had significantly higher levels of HCT, HGB, RBC and percent of reticulocytes (by 4.6%, 6.4%, 5%, and 16.6%, respectively, p < 0.05). The post-hoc Tukey’s test showed a significant increase in serum EPO levels during the first two weeks of the HiHiLo protocol in the group H. However, the highest significant increase (by 146.8%; p < 0.001) in EPO levels was observed after the first 24 hours of the HiHiLo protocol. Further continuation of the HiHiLo procedure caused smaller significant (p < 0.001) increases in concentration of this hormone (72h – 125%, 1 week – 106.25%, 2 week – 75% compared to the baseline). Opposing changes were observed in values of SpO2 during the HiHiLo protocol in the group H, where analysis showed a significant decrease in values of SpO2 (Figure 3). The statistical analysis also revealed significantly (p < 0.01) lower VO2 values for selected submaximal workloads in the group H (VO2 -1LT by -5.7%, VO2 LT by -4.4%, VO2 +1LT by -6%) and in the group C (VO2 -1LT by -3.5%, VO2 LT by -2.1%, and VO2 +1LT by -2.4% -).
The main finding of our study is that the 3-weeks HiHiLo protocol did not improve aerobic capacity estimated by VO2max in spite of significantly higher values of haematological variables. Moreover, the energy cost during submaximal exercise performed by the biathletes from the group H was almost twice as low as that recorded for their counterparts training in normoxia. Thus, the results of this study only partially confirm earlier reports on the effectiveness of the HiHiLo protocol, e.g. in terms of both a higher hemoglobin level and a hematocric value. The main mechanism which improves VO2max and exercise capacity after extended exposure to hypoxia is a faster rate of erythropoiesis. Our observation of a significant increase in serum EPO levels during the first two weeks of HiHiLo training at 2015 m is in accordance with earlier studies (Ashenden et al., 2000; Chapman et al., 1998; Friedmann et al., 1999; Rusko et al., 1999; Stray-Gundersen et al., 2001, Wehrlin et al., 2006). In case of LH-TH and LH-TL training, two factors are considered to be responsible for improvement of hematological variables, e.g. absolute altitude (hypoxia) and the length of exposure to hypoxia (see: Ashenden et al., 1999a; Gore and Hopkins, 2005; Fudge et al., 2012). Chapman et al. (2014) observed a significant improvement in sport performance in groups living at the middle two altitudes (2,085 and 2,454 m) for four weeks, but not in groups living at 1,780 and 2,800 m. Moreover, EPO levels were significantly higher in all groups at 24 and 48 h, but returned to baseline levels after 72 h in the 1,780-m group. These results suggest that, altitude between 2,000 and 2,500 m during the LH-TL protocol allows for an optimal acclimatization response for sea level performance. In most experiments in which the subjects trained at altitude for 100-200 hours over a period of 3-4 weeks neither haemoglobin mass nor the volume of erythrocytes increased significantly (Ashenden et al., 1999a; 1999b; Saunders et al., 2004b). Contrary, in several experiments with the LH-TL protocol where the subjects’ exposure to hypoxia was extended to 250 hours or longer (300-550 h) haemoglobin mass increased significantly by 8-10% (Brugniaux et al., 2006; Schmidt et al., 2002), and 4-7% (Clark et al., 2009; Friedmann et al., 2005; Gough et al., 2012; Rusko et al., 1999; Wehrlin et al., 2006), respectively. In contrast, in several experiments where the subjects were exposed to altitude for around 750 hours the haematological indicators did not change significantly, showing only an upward trend (Gore et al., 1998). Farther, Gore et al. (2013) reported in their meta-analysis that hemoglobin mass (Hbmass) increases at approximately 1.1%/100 h of altitude exposure, regardless of whether the exposure is classic altitude (>2100 m) or LHTL (~3000 m), and that after a typical exposure of 300–400 h the increase above pre-altitude values persists for ~3 weeks. The results of this study confirm the aforementioned findings. Group H staying at altitude for 470-480 hours had significantly higher concentrations of HGB, RBC, HCT and percent of reticulocytes compared with their initial values (by 6.4%, 5%, 4.6% and 16,6%, respectively). However, these positive changes in the subjects’ haematological parameters did not contribute to higher levels of their VO2max or maximal workload (WRmax) during the incremental treadmill test in group H. However, during the progressive exercise protocol, in all presented submaximal exercise loads VO2 was significantly lower in comparison to pre-training values (S1 trial). These changes and significantly lower levels of the maximal respiratory exchange ratio (RERmax), as well as a downward trend in VEmax, BFmax and HRmax indicate that subjects in group H ended the incremental treadmill test before reaching maximal exercise capacity. This interpretation is supported by the absence of similar changes in the group C and significant changes in the acid-base balance indicators in the group H, i.e. lower values of ∆LA, ∆pH and ∆BE (S1 vs S2). No improvement in VO2max may be explained, by a recent investigation of Robach et al. (2012). The results of that study are similar to ours, showing no significant increases in VO2max values, irrespective of treatment (LHTL: 1.5%; placebo: 2.0%). However Hbmass was slightly increased (4.6%) in 5 (of 10) LHTL participants, yet this was not accompanied by an increase in VO2max. The authors observed that in the subjects demonstrating an increase in Hbmass, isovolumic haemodilution elicited a 5.8% decrease in VO2max. Neither maximal capacity of oxidative phosphorylation nor mitochondrial efficiency was modified by time or LH-TL procedure. The authors suggested that LH-TL has no positive effect on VO2max in endurance-trained athletes because maximal muscle oxidative capacity is not improved following the LH-TL protocol, and erythrocyte volume expansion after LH-TL, if any, is too small to alter O2 transport. Some data indicate a gap between the completion of the protocol and the repetition of the incremental test as a possible factor influencing the results of VO2max. This may be caused by the different volume or/and intensity of applied training loads, what implies a different recovery period. This hypothesis is in accordance with the results obtained after various altitude training protocols (Levine and Stray-Gundersen, 1997; Mattila and Rusko, 1996, Rusko et al., 1999). Endurance capacity depends not only on maximal aerobic capacity expressed as VO2max, but also on two other factors. One of them is the capability of the organism to maintain exercise intensity during extended physical effort at a level corresponding to a high percentage of VO2max, usually expressed as lactate threshold whereas the other one is the energy cost of exercise. The available reports indicate that hypoxic training can decrease the energy cost of exercise by reducing oxygen demand by 3-10% (Neya et al., 2007, Saunders et al., 2004b). If one wished to induce similar changes in elite athletes using only endurance training in normoxia, then in many cases the process might extend over a period of several years. Coyle (2005) observed that, six years of systematic endurance training reduced the energy cost of exercise by 8%. This results are confirmed by the outcomes of this experiment, where the absolute values of WRLT were significantly higher (by 4%) in subjects following the HiHiLo protocol while their absolute VO2LT values remained unchanged, and where a significant increase in the relative values of VO2LT was also accompanied by reduced fat content. In the group C similar changes were not noted. Another difference pointing to an improved energy cost of exercise consisted in lower VO2 values (almost twice as high as in the group C) recorded after HiHiLo training in the group H during submaximal workloads during the incremental test. The improvement might be attributed to two factors: 1) a better technique of movement directly reducing the energy cost, and 2) more efficient cellular metabolism (Saunders et al., 2004a). Since the biathletes participating in the experiment had several years of training experience, it does not seem probable that they could improve the technique of movement required by the incremental test. Therefore, reduction in the energy cost noted in this experiment may be linked with adaptive changes in the aerobic metabolism in muscle cells. Our results are consistent with the outcomes of other studies with subjects exposed to hypoxia, where a lower energy cost by 3-10% was registered as well as lower oxygen uptake (VO2) during submaximal efforts (Gore et al., 2001; Green et al., 2000; Katayama et al., 2003; 2004; Marconi et al., 2005; Neya et al., 2007; Saunders et al., 2004b; Ponsot et al., 2006; Schmidt et al., 2006). Interestingly, Hochoachka et al. (1991) and Marconi et al. (2005) showed a similar effect in highlanders who had a lower energy cost of exercise by 15% compared to lowlanders during exercise performed in normoxia at the same relative intensity. However, Lundby et al. (2007) in several independent investigations observed that exercise economy remains unchanged after acclimatization to moderate and high altitude. They reported that submaximal VO2 is unchanged 2-3 days after chronic exposure at moderate altitude (2500-3000 m) as well as after acute and chronic exposure to high altitudes (4100 – 5260 m). Moreover Schmitt et al. (2006) also did not register improvements in energy cost of exercise during the LH-TL protocol (between pre- and post-treatment- 1 day) but they observed improvement in this range during post-altitude period of sea-level training (between post- 1 day and post 15 days) in the non-specific activity that is similar to our findings. This changes in energy cost were explained by the combination of positive effects as a decrease in ventilation at the same workload and a greater oxidation of carbohydrates (Saunders et al., 2004b), or negative ones as the fatigue induced by the additional hypoxic stress, as energy cost was shown to increase under the influence of fatigue (Morgan et al., 1989).
The probable reasons for conflicting results in studies related to the influence of altitude training on aerobic capacity and endurance performance in normoxia are due to different methodological assumptions adopted by researchers, as well as variations in the subjects’ training experience and thereby responsiveness to hypoxic stimuli. The findings of our study indicate that 3 weeks of HiHiLo training can improve the energy exercise cost and some hematological variables without improvement in VO2max of highly endurance trained biathletes. The positive changes observed in our participants led to a conclusion that this training method could effectively improve endurance in normoxia, since most of the biathlon competition is performed at submaximal intensities.
|
AUTHOR BIOGRAPHY |
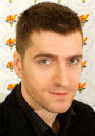 |
Milosz Czuba |
Employment: |
Degree: PhD |
Research interests: Endurance training, hypoxia, sports nutrition, exercise physiology |
E-mail: m.czuba@awf.katowice.pl |
|
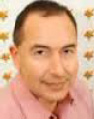 |
Adam Maszczyk |
Employment: Department of Statistics and Methodology, The Jerzy Kukuczka Academy of Physical Education in Katowice, Poland. |
Degree: PhD |
Research interests: Artificial neural networks, multidimensional analysis |
E-mail: a.maszczyk@awf.katowice.pl |
|
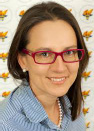 |
Dagmara Gerasimuk |
Employment: The Jerzy Kukuczka Academy of Physical Education in Katowice, Poland, Department of Sports Training. |
Degree: PhD |
Research interests: Endurance training, youth sports, biathlon |
E-mail: d.gerasimuk@awf.katowice.pl |
|
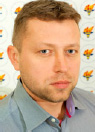 |
Robert Roczniok |
Employment: The Jerzy Kukuczka Academy of Physical Education in Katowice, Department of Statistics and Methodology, Poland. |
Degree: PhD |
Research interests: Artificial neural networks, multidimensional analysis, taxonomic analysis, control of sport training |
E-mail: r.roczniok@awf.katowice.pl |
|
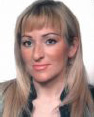 |
Olga Fidos-Czuba |
Employment: The Jerzy Kukuczka Academy of Physical Education in Katowice, Department of Statistics and Methodology, Poland. |
Degree: PhD student |
Research interests: Piano science, coordination skills, hypoxia |
E-mail: |
|
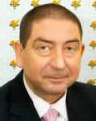 |
Adam Zając |
Employment: The Professor, Jerzy Kukuczka Academy of Physical Education in Katowice, Department of Sports Training, Poland. |
Degree: PhD |
Research interests: Strength training, sports nutrition, exercise physiology |
E-mail: a.zajac@awf.katowice.pl |
|
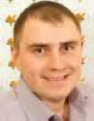 |
Artur Gołaś |
Employment: The Jerzy Kukuczka Academy of Physical Education in Katowice, Department of Biochemistry, Poland. |
Degree: PhD |
Research interests: Strength training, biomechanics, sports nutrition |
E-mail: a.golas@awf.katowice.pl |
|
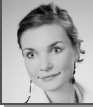 |
Aleksandra Mostowik |
Employment: Department of Statistics and Methodology, The Jerzy Kukuczka Academy of Physical Education in Katowice, Poland. |
Degree: PhD student |
Research interests: Artificial neural networks, multidimensional analysis |
E-mail: |
|
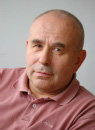 |
Jozef Langfort |
Employment: The Professor, Jerzy Kukuczka Academy of Physical Education in Katowice, Department of Nutrition, Poland. |
Degree: PhD |
Research interests: Physiology, hypoxia, sports nutrition |
E-mail: j.langort@awf.katowice.pl |
|
|
|
REFERENCES |
 Ashenden M.J., Gore C.J., Dobson G.P., Hahn A.G. (1999a) ‘Live high, train low’ does not change the total haemoglobin mass of male endurance athletes sleeping at a simulated altitude of 3000 m for 23 nights. European Journal of Applied Physiology 80, 479-484.
|
 Ashenden M.J., Gore C.J., Dobson G.P., Hahn A.G. (2000) Simulated moderate altitude elevates serum erythropoietin but does not increase reticulocyte production in well-trained runners. European Journal of Applied Physiology 81, 428-435.
|
 Ashenden M.J., Gore C.J., Martin D.T., Dobson G.P., Hahn A.G. (1999b) Effects of a 12-day ‘live high, train low’ camp on reticulocyte production and haemoglobin mass in elite female road cyclists. European Journal of Applied Physiology 80, 472-478.
|
 Bonetti D.L., Hopkins W.G. (2009) Sea-level exercise performance following adaptation to hypoxia, a meta-analysis. Sports Medicine 39, 107-127.
|
 Brugniaux J.V., Schmitt L., Robach P., Nicolet G., Fouillot J.P., Moutereau S., Lasne F., Pialoux V., Saas P., Chorvot M.C., Cornolo J., Olsen N.V., Richalet J.P. (2006) Eighteen days of “Living High - Training Low” stimulate erythropoiesis and enhance aerobic performance in elite middle-distance runners. Journal of Applied Physiology 96, 66-77.
|
 Chapman R.F., Karlsen T., Resaland G.K., Ge R.L., Harber M.P., Witkowski S., Stray-Gundersen J., Levine B.D. (2014) Defining the "dose" of altitude training, how high to live for optimal sea level performance enhancement. Journal of Applied Physiology 116, 595-603.
|
 Chapman R.F., Stray-Gundersen J., Levine B.D. (1998) Individual variation in response to altitude training. Journal of Applied Physiology 85, 1448-1456.
|
 Cheng B., Kuipers H., Snyder A.C., Keizer H.A., Jeukendrup A., Hesselink M. (1992) A new approach for the determination of ventilator and lactate thresholds. International Journal of Sport Medicine 13, 518-522.
|
 Clark A.S., Quod M.J., Clark M.A., Martin D.T., Saunders P.U., Gore C.J. (2009) Time course of haemoglobin mass during 21 days live high,train low simulated altitude. European Journal of Applied Physiology 106, 399-406.
|
 Coyle F. (2005) Improved muscular efficiency displayed as Tour de France champion matures. Journal of Applied Physiology 98, 2191-2196.
|
 Czuba M., Waskiewicz Z., Zajac A., Poprzecki S., Cholewa J., Roczniok R. (2011) The effects of intermittent hypoxic training on aerobic capacity and endurance performance in cyclists. Journal of Sports Science and Medicine 10, 175-183.
|
 Czuba M., Zając A., Cholewa J., Poprzęcki S., Waśkiewicz Z. (2009) Lactate threshold (D-max method) and maximal lactate steady-state in cyclists. Journal of Human Kinetics 21, 49-56.
|
 Czuba M., Zając A., Maszczyk A., Roczniok R., Poprzęcki S., Garbaciak W., Zając T. (2013) The effects of high intensity interval training in normobaric hypoxia on aerobic capacity in basketball players. Journal of Human Kinetics 39, 103-114.
|
 Friedmann B., Frese F., Menold E., Bartsch P. (2005) Individual variation in the reduction of heart rate and performance at lactate thresholds in acute normobaric hypoxia. International Journal of Sport Medicine 26, 531-536.
|
 Friedmann B., Jost J., Rating T., Weller E., Werle E., Eckardt K-U, Bärtsch P., Mairbäurl H. (1999) Effects of iron supplementation on total body haemoglobin during endurance training at moderate altitude. International Journal of Sports Medicine 20, 78-85.
|
 Fudge B.W., Pringle J.S., Maxwell N.S., Turner G., Ingham S.A., Jones A.M. (2012) Altitude training for elite endurance performance, a 2012 update. Current Sports Medicine Reports 11, 148-54.
|
 Gore C.J., Clark S.A., Saunders P.U. (2007) Non-hematological mechanisms of improved sea-level performance after hypoxic exposure. Medicine and Science in Sports and Exercise 39, 1600-9.
|
 Gore C.J., Hahn A.G., Aughey R.J., Martin D.T., Ashenden M.J., Clark S.A., Garnham A.P., Roberts A.D., Slater G.J., McKenna M.J. (2001) Live high,train low increases muscle buffer capacity and submaximal cycling efficiency. Acta Physiologica Scandinavica 173, 275-286.
|
 Gore C.J., Hahn A.G., Aughey R.J., Martin D.T., Ashenden M.J., Clark S.A., Garnham A.P., Roberts A.D., Slater G.J., McKenna M.J. (2001) Live high,train low increases muscle buffer capacity and submaximal cycling efficiency. Acta Physiologica Scandinavica 173, 275-286.
|
 Gore C.J., Hahn A.G., Rice A., Bourdon P., Lawrence S., Walsh C., Stanef T., Barnes P., Parisotto R., Martin D., Pyne D., Gore C. (1998) Altitude training at 2690 m does not increase total haemoglobin mass or sea level VO in world champion track cyclists. Journal of Science and Medicine in Sport 1, 156-170.
|
 Gore C.J., Hopkins W.G. (2005) Counterpoint, positive effects of intermittent hypoxia (live high,train low) on exercise performance are not mediated primarily by augmented red cell volume. Journal of Applied Physiology 99, 2055-2058.
|
 Gore C.J., Sharpe K., Garvican-Lewis L.A., Saunders P.U., Humberstone C.E., Robertson E.Y., Wachsmuth N.B., Clark S.A., McLean B.D., Friedmann-Bette B., Neya M., Pottgiesser T., Schumacher Y.O., Schmidt W.F. (2013) Altitude training and haemoglobin mass from the optimised carbon monoxide rebreathing method determined by a meta-analysis. British Journal of Sports Medicine 47, i31-9.
|
 Gough C.E., Saunders P.U., Fowlie J., Savage B., Pyne D.B., Anson J.M., Wachsmuth N., Prommer N., Gore C.J. (2012) Influence of altitude training modality on performance and total haemoglobin mass in elite swimmers. European Journal of Applied Physiology 112, 3275-3285.
|
 Green H., MacDougall J., Tarnopolsky M., Melissa N.L. (1999) Downregulation of Na+-K+-ATPase pumps in skeletal muscle with training in normobaric hypoxia. Journal of Applied Physiology 86, 1745-1748.
|
 Green H.J., Roy B., Grant S. (2000) Increases in submaximal cycling efficiency mediated by altitude acclimatization. Journal of Applied Physiology 89, 1189-1197.
|
 Hochoachka P.W., Stanley C., Matheson G.O., McKenzie D.C., Allen P.S., Parkhouse W.S. (1991) Metabolic and work efficiencies during exercise in Andean natives. Journal of Applied Physiology 70, 1720-1730.
|
 Katayama K., Matsuo H., Ishida K., Mori S., Miyamura M. (2003) Intermittent hypoxia improves endurance performance and submaximal exercise efficiency. High Altitude Medicine & Biology 4, 291-304.
|
 Katayama K., Sato K., Matsuo H., Ishida K., Iwasaki K., Miyamura M. (2004) Effect of intermittent hypoxia on oxygen uptake during submaximal exercise in endurance athletes. European Journal of Applied Physiology 92, 75-83.
|
 Kuipers H., Verstappen F.T.J., Keizer H.A., Guerten P., van Kranenburg G. (1985) Variability of aerobic performance in the laboratory and its physiological correlates. International Journal of Sports Medicine 6, 197-201.
|
 Levine B.D., Stray-Gundersen J. (1997) "Living high-training low", effect of moderate-altitude acclimatization with low-altitude training on performance. Journal of Applied Physiology 83, 102-112.
|
 Lundby C., Calbet J.A., Sander M., van Hall G., Mazzeo R.S., Stray-Gundersen J., Stager J.M., Chapman R.F., Saltin B., Levine B.D. (2007) Exercise economy does not change after acclimatization to moderate to very high altitude. Scandinavian Journal of Medicine & Science in Sports 17, 281-291.
|
 Marconi C., Marzorati M., Sciuto D., Ferri A., Cerretelli P. (2005) Economy of locomotion in high-altitude Tibetan migrants exposed to normoxia. The Journal of Physiology 569, 667-675.
|
 Mattila V., Rusko H. (1996) Effect of living high and training low on sea level performance in cyclists. Medicine and Science in Sports and Exercise 28, 157-.
|
 Melissa L., Macdougall J.D., Tarnopolsky M.A., Cipriano N., Green H.J. (1997) Skeletal muscle adaptations to training under normobaric hypoxic versus normoxic conditions. Medicine and Science in Sports and Exercise 29, 238-243.
|
 Millet G.P., Faiss R., Brocherie F., Girard O. (2013) Hypoxic training and team sports, a challenge to traditional methods?. British Journal of Sports Medicine 47, 16-7.
|
 Millet G.P., Faiss R., Pialoux V. (2012) Point, hypobaric hypoxia induces different physiological responses from normobaric hypoxia. Journal of Applied Physiology 112, 1783-4.
|
 Millet G.P., Roels B., Schmitt L., Woorons X., Richalet J.P. (2010) Combining hypoxic methods for peak performance. Sports Medicine 40, 1-25.
|
 Morgan D.W., Martin P.E., Krahenbuhl G.S. (1989) Factors affecting running economy. Sports Medicine 7, 310-330.
|
 Neya M., Enoki T., Kumai Y., Sugoh T., Kawahara T. (2007) The effects of nightly normobaric hypoxia and high intensity training under intermittent normobaric hypoxia on running economy and hemoglobin mass. Journal of Applied Physiology 103, 828-834.
|
 Ponsot E., Dufour S.P., Zoll J., Doutrelau S., N‘Guessan B., Geny B., Hoppeler H., Lampert E., Mettauer B., Ventura-Clapier R., Richard R. (2006) Exercise training in normobaric hypoxia in endurance runners. II. Improvement of mitochondrial properties in skeletal muscle. Journal of Applied Physiology 100, 1249-1257.
|
 Robach P., Siebenmann C., Jacobs R.A., Rasmussen P., Nordsborg N., Pesta D., Gnaiger E., Díaz V., Christ A., Fiedler J., Crivelli N., Secher N.H., Pichon A., Maggiorini M., Lundby C. (2012) The role of hemoglobin mass on VO(2)max following normobaric ‘live high-train low‘ in endurance-trained athletes. British Journal of Sports Medicine 46, 822-827.
|
 Rodriguez F.A., Truijens M.J., Townsend N.E., Martini E.R., Stray-Gundersen J., Gore C.J., Levine B.D. (2004) Effects of four weeks of intermittent hypobaric hypoxia on sea level running and swimming performance. Medicine and Science in Sports and Exercise 36, 338-.
|
 Roels B., Bentley D.J., Coste O., Mercier J., Millet G.P. (2007) Effects of intermittent hypoxic training on cycling performance in well-trained athletes. European Journal of Applied Physiology 101, 359-68.
|
 Roels B., Millet G.P., Marcoux C.J.L., Coste O., Bentley D.J., Candau R.B. (2005) Effects of hypoxic interval training on cycling performance. Medicine and Science in Sports and Exercise 37, 138-146.
|
 Rusko H., Tikkanen H., Hamalainen I., Kalliokoski K., Puranen A. (1999) Effect of living in hypoxia and training in normoxia on sea level VOmax and red cell mass. Medicine and Science in Sports and Exercise 31, 86-.
|
 Saltin B., Larsen H., Terrados N., Bangsbo J., Bak T., Kim C.K., Svedenhag J., Rolf C.J. (1995) Aerobic exercise capacity at sea level and at altitude in Kenyan boys, junior and senior runners compared with Scandinavian runners. Scandinavian Journal of Medicine and Science in Sports 5, 209-221.
|
 Saunders P.U., Pyne D.B., Telford R.D., Hawley J.A. (2004a) Factors Affecting Running Economy in Trained Distance Runners. Sports Medicine 34, 465-485.
|
 Saunders P.U., Telford R.D., Pyne D.B., Cunningham R.B., Gore C.J., Hahn A.G., Hawley J.A. (2004b) Improved running economy in elite runners after 20 days of simulated moderate-altitude exposure. Journal of Applied Physiology 96, 931-937.
|
 Schmidt W., Heinicke K., Rojas J., Manuel Gomez J., Serrato M., Mora M., Wolfarth B., Schmid A., Keul J. (2002) Blood volume and hemoglobin mass in endurance athletes from moderate altitude. Medicine and Science in Sports and Exercise 34, 934-1940.
|
 Schmitt L., Millet G., Robach P., Nicolet G., Brugniaux J.V., Fouillot J.P., Richalet J.P. (2006) Influence of living high training low on aerobic performance and economy of work in elite athletes. European Journal of Applied Physiology 97, 627-636.
|
 Stray-Gundersen J., Chapman R., Levine B.D. (2001) ‘Living high-training low’ altitude training improves sea level performance in male and female elite runners. Journal of Applied Physiology 91, 1113-1120.
|
 Svedenhag J., Saltin B., Johansson C., Kaijser L. (1991) Aerobic and anaerobic exercise capacities of elite middle-distance runners after two weeks of training at moderate altitude. Scandinavian Journal of Medicine and Science in Sports 1, 205-214.
|
 Telford R.D., Graham K.S., Sutton J.R., Hahn A.G., Campbell D.A., Creighton S.W. (1996) Medium altitude training and sea-level performance. Medicine and Science in Sports and Exercise 28, 124-.
|
 Wehrlin J.P., Zuest P., Hallen J., Marti B. (2006) Live High - Train Low for 24 days increases hemoglobin mass and red cell volume in elite endurance athletes. J. Appl. Physiol. 100, 1938-1945.
|
 Wilber R.L., Stray-Gunderson J., Levine B.D. (2007) Effect of Hypoxic “Dose” on Physiological Responses and Sea-Level Performance. Medicine and Science in Sports and Exercise 39, 1590-1599.
|
|
|
|
|
|
|