|
|
|
ABSTRACT |
This study determined the effects of heavy resistance training and daily overfeeding with carbohydrate and/or protein on blood and skeletal muscle markers of protein synthesis (MPS), myogenesis, body composition, and muscle performance. Twenty one resistance-trained males were randomly assigned to either a protein + carbohydrate [HPC (n = 11)] or a carbohydrate [HC (n = 10)] supplement group in a double-blind fashion. Body composition and muscle performance were assessed, and venous blood samples and muscle biopsies were obtained before and after eight weeks of resistance training and supplementation. Data were analyzed by two-way ANOVA (p ≤ 0.05). Total body mass, body water, and fat mass were significantly increased in both groups in response to resistance training, but not supplementation (p < 0.05); however, lean mass was not significantly increased in either group (p = 0.068). Upper- (p = 0.024) and lower-body (p = 0.001) muscle strength and myosin heavy chain (MHC) 1 (p = 0.039) and MHC 2A (p = 0.027) were also significantly increased with resistance training. Serum IGF-1, GH, and HGF were not significantly affected (p > 0.05). Muscle total DNA, total protein, and c-Met were not significantly affected (p > 0.05). In conjunction with resistance training, the peri-exercise and daily overfeeding of protein and/or carbohydrate did not preferentially improve body composition, muscle performance, and markers indicative of MPS and myogenic activation. |
Key words:
Protein, carbohydrate, muscle strength, hypertrophy, muscle protein synthesis, myogenesis
|
Key
Points
- In response to 56 days of heavy resistance training and HC or HPC supplementation, similar increases in muscle mass and strength in both groups occurred; however, the increases were not different between supplement groups.
- The supplementation of HPC had no preferential effect on augmenting serum IGF-1 GH, or HGF.
- The supplementation of HPC had no preferential effect on augmenting increases in total muscle protein content or the myogenic markers, total DNA and muscle cMet content.
- In response to 56 days of a daily supplemental dose of 94 g of protein and 196 g of carbohydrate, the HPC group was no more effective than 312 g of carbohydrate in the HC group in increasing muscle strength and mass due to its ability to elevate serum anabolic hormones and growth factors and markers of myogenic activation of satellite cells.
|
Resistance exercise instigates changes in protein turnover in skeletal muscle that occur up to 48 hours into recovery (Biolo et al., 1995). Simultaneously, the rate of muscle protein degradation is also increased after exercise (Phillips et al., 1997). Whey protein (Ferguson-Stegall et al., 2011) and carbohydrate (Bird et al., 2006; Ferguson-Stegall et al., 2011; Thyfault et al., 2004) have been shown to augment muscle protein synthesis (MPS) with resistance exercise. For example, the stimulatory effect of protein supplementation on MPS may be attributed in part to an increase in amino acid supply for the muscle, thereby augmenting substrate availability for protein synthesis (Bohe et al., 2001; 2003). Conversely, the effectiveness of carbohydrate supplementation on MPS appears to reside with insulin’s ability to bind its respective receptor and subsequently up-regulate the Akt/mTOR signaling pathway. As such, it has been shown that 10 weeks (Willoughby et al., 2007) and 12 weeks (Rahbek et al., 2014) of resistance training in conjunction with daily whey protein and/or carbohydrate supplementation resulted in enhanced activation of protein signaling intermediates indicative of MPS and muscle protein content, along with subsequent improvements in muscle performance. In both studies, whey protein was more effective than carbohydrate in inducing an anabolic response; however, the effectiveness of carbohydrate was also demonstrated. It is common practice in those aspiring to significantly increase their muscle mass, such as bodybuilders, to employ a hyper-caloric, overfeeding diet during their so-called “off-season” between competitions, a practice referred to as “bulking.” In regard to energy balance, this mismatch between macronutrient intake and oxidation often leads to an increase in body composition even if energy balance remains unchanged (Hill et al., 1991). In fact, this practice typically results in macronutrient intakes that far exceed total daily energy expenditure (TDEE), thereby contributing to significant gains in fat mass. Oftentimes, the gain in fat mass far outweighs any gain in muscle mass. This bulking process from overfeeding involving high fat (Akiyama et al., 1996), and high carbohydrate (Coughlan et al., 2015; Samocha-Bonet et al., 2012) diets can cause metabolic dysregulation, which is associated with systemic inflammation and insulin resistance. In general, the condition (just as with Type 2 diabetes) results in a decrease in skeletal muscle glucose uptake and also in insulin’s ability to stimulate mTOR activity and subsequent MPS. Concurrently, this process of systemic inflammation accompanying insulin resistance results in chronic increases in cortisol which plays a role in inducing de novo lipogenesis (Vienberg and Bjornholm, 2014). There are a number of recent scientific studies showing that large amounts of protein ingested are no more effective at increasing MPS than a less amount of protein (Areta et al., 2013; Moore et al., 2012; Rennie et al., 2002). This generates the notion of the “muscle full effect” (Atherton et al., 2010) and is based on the premise that an upper limit of amino acid delivery must be achieved before muscle can no longer use amino acids as substrate for muscle protein synthesis, thereby resulting in the amino acids being diverted toward catabolic processes instead. So, in essence “bulking up” may result in two primary metabolic outcomes which can be associated with: 1) blunting the ability to increase muscle mass and 2) enhancing the ability to increase fat mass. The rationale for the use of oral, anabolic nutritional supplements is based on the assumption that they will improve net muscle protein synthesis above and beyond that afforded by regular food ingestion alone. However, from the research available at this time it appears that protein and/or carbohydrate ingestion associated with overfeeding/bulking does not preferentially augment muscle mass in conjunction with resistance training. Therefore, the purpose of this study was to determine the effects of eight weeks (56 days) of heavy resistance training combined with the oral ingestion of Stealth® supplementation. The specific aims of the study were to determine to what extent daily overfeeding from protein and/or carbohydrate supplementation for eight weeks in conjunction with resistance training affected resistance training adaptations (body composition, muscle strength, and total muscle protein content), serum growth factors (IGF-1 and GH), and myogenic markers of satellite cell activation (serum HGF and muscle DNA and c-Met protein content) compared to iso-energetic carbohydrate in resistance-trained men.
Experimental approachIn a randomized, double-blind, parallel design, resistance-trained males participated in a four-day/week heavy resistance training program for eight weeks in conjunction with daily overfeeding of either a protein and/or carbohydrate supplement. Body composition and muscle performance were assessed, along with venous blood samples and muscle biopsies being obtained before and after the eight-week intervention.
ParticipantsApparently healthy, resistance-trained [regular, consistent resistance training (i.e. thrice weekly) for at least one year prior to the onset of the study], males between the ages of 18-35 and a body mass index between 18.5-30 kg·m-2 volunteered to participate in the study. Enrollment was open to men of all ethnicities. Only participants considered as low risk for cardiovascular disease and with no contraindications to exercise as outlined by the American College of Sports Medicine (ACSM), and who had not consumed any nutritional supplements (excluding multi-vitamins) three months prior to the study, were allowed to participate. All participants signed university-approved informed consent documents and were cleared for participation by passing a mandatory medical screening. Approval to conduct the study was granted by the Institutional Review Board for the Protection of Human Subjects in Research of Baylor University. Additionally, all experimental procedures involved in the study conformed to the ethical consideration of the Declaration of Helsinki.
Assessment of body compositionBody composition was determined on Day 0 and 57 based on our previous guidelines (Shelmadine et al., 2009; Spillane et al., 2009; 2011; 2012; Willoughby et al., 2007). Total body mass (kg) was determined on a standard dual beam balance scale (Detecto Bridgeview, IL, USA). Total body water was determined with bioelectrical spectroscopy [(BIS) ImpediMed Ltd., Australia]. Fat mass and lean mass were determined using DEXA (Hologic Discovery Series W, Waltham, MA, USA). Quality control calibration procedures were performed on a spine phantom prior to each participant. Previous studies in our lab have shown the accuracy of DEXA for body composition to be ±2.3% as assessed by direct comparison with hydrodensitometry and scale weight.
Assessment of muscle performanceAt Day 0 and 57, the determination of the one-repetition maximum (1-RM) for the angled leg press and knee extension exercises was based upon our previous procedures (Shelmadine et al., 2009; Spillane et al., 2009; 2011; 2012; Willoughby et al., 2007). As a warm-up, an estimated 50% 1-RM was utilized to complete 10 repetitions. After a two minute rest period, a load of 70% of estimated 1-RM was utilized to perform five repetitions. At this point, the weight was gradually increased until a 1-RM was reached, with a two minute rest period in between each successful lift. Test-retest reliability of performing these strength assessments on subjects within our laboratory has demonstrated low mean coefficients of variation and high reliability for the angled leg press (2.1%, intra-class r = 0.95) and knee extension (0.79%, intra-class r = 0.94), respectively.
Dietary analysis and energy expenditureFor the four consecutive days immediately prior to reporting to the lab for testing at Day 0 and 57, participants were instructed to record their dietary intake. During each of these four-day periods, as well as the duration of the study, participants were instructed to not change their usual dietary habits. The dietary data were analyzed with the Food Processor dietary assessment software (ESHA Research, Salem, OR, USA) for determination of the average intake of total kilocalories, protein, carbohydrate, and fat. Total daily energy expenditure (TDEE) was assessed by calculating resting energy expenditure using the Cunningham equation, which is based more specifically on recreational athletes, and then multiplied by a physical activity factor commensurate with each participant’s respective levels of physical activity to estimate TDEE needs to maintain their present body mass (Haaf and Weijs, 2014).
Venous blood sampling and muscle biopsiesAt Days 0 and 57, venous blood samples and muscle biopsies were obtained during the testing sessions. Blood was collected from the antecubital vein into a 10 ml serum sample tube. Blood samples were allowed to stand at room temperature for 10 minutes and then centrifuged. The serum was removed and frozen at -80°C for later analysis. Using a 5 mm Bergstrom needle, percutaneous muscle biopsies (~50 mg) were obtained from the middle portion of the vastus lateralis muscle of the dominant leg at the midpoint between the patella and the greater trochanter of the femur at a depth of one cm based on our previous procedures (Spillane et al., 2009; 2014; Willoughby et al., 2007). For the biopsy obtained at Day 57, attempts were made to extract tissue from approximately the same location as the initial biopsy by using the pre-biopsy scar, depth markings on the needle, and a successive incision that was made approximately one cm to the former from medial to lateral. After removal, muscle samples were trimmed of adipose tissue and immediately frozen in liquid nitrogen, and then stored at -808C for later analysis.
Supplementation protocolIn double-blind fashion, participants were assigned an eight-week (56-day) supplementation protocol consisting of the total oral ingestion of either a 312 g·day-1 of a carbohydrate supplement [HC (n =10), Vital Pharmaceuticals, Inc., Weston, FL, USA] or a protein and carbohydrate supplement [HPC (n = 11), Stealth®, Vital Pharmaceuticals, Inc., Weston, FL, USA]. The total daily caloric load of carbohydrate received as maltodextrose in the HC group was 1,248 kcals (312 g). In the HPC group, the total daily caloric load was also 1,248 kcals (312 g), but consisted of 94 g, 196 g, and 22 g of protein, carbohydrate/maltodextrose, and fat, respectively. Both supplements were iso-energetic in dose and identical in color and texture. For both supplements, half of the total daily dosage (156 g) was mixed with 15 oz of water and ingested 30 minutes prior to each exercise session and half (156 g) mixed with 15 oz of water and ingested within 30 minutes following each exercise session. For days where no exercise occurred, the full dosage of supplement was ingested in the morning upon waking. Supplementation compliance was monitored by having participants complete daily supplementation questionnaires and by returning empty containers of their respective supplement on day 57.
Resistance training protocolBased on our previous protocol (Spillane et al., 2011; 2012), participants engaged in a supervised, four-day/week resistance training program that was periodized and split into two upper- and two lower-body workouts per week for a total of eight weeks (56 days). Prior to each exercise session, participants performed a standardized series of calisthenics and stretching exercises. The participants then performed an upper body resistance-training program consisting of such exercises as bench press, lat pulldown, shoulder press, seated row, shoulder shrug, chest fly, biceps curl, triceps press down, and abdominal curl twice per week, and a lower-body program consisting of such exercise as leg press, back extension, step up, leg curl, leg extension, heel raise, and abdominal curl, also performed twice per week. Participants performed three sets of 8-10 repetitions with as much weight as they could lift per set (typically 70 – 80% of 1RM). Rest periods between exercises and sets were two minutes in duration. Exercise sessions were supervised by study personnel, monitoring the number of sets, repetitions, and weight performed for each exercise.
Resistance training volume loadVolume load (weight x sets x reps) was determined for the workout sessions throughout the resistance-training program. Volume load was recorded for each exercise in both upper- and lower-body workouts in both groups.
Assessment of serum IGF-1, GH, and HGFFrom the two blood samples obtained at day 0 and day 57, serum samples were analyzed for IGF-1, GH, (Enzo Life Sciences, Plymouth Meeting, PA, USA), and HGF (Ray Biotech, Norcross, GA, USA) using commercially-available enzyme-linked immunoabsorbent assay (ELISA) kits. The sensitivity of the IGF-1 kit is 48.5 pg·ml-1, and does not cross-react with IGF-2, and IGFBPs 2-4, insulin, or GH. For GH, this kit has a sensitivity of 0.2 ng/ml and no cross-reactivity with human chorionic gonadotrophin (HCG) or prolactin. The HGF kit has a sensitivity of 2 pg·ml-1 and has no cross-reactivity with angiogenin, BDNF, BLC, ENA-78, FGF-4, IL-1 alpha, IL-1 beta, IL-2, IL-3, IL-4, IL-5, IL-7, IL-8, IL-9, IL-10, IL-11, IL-12 p70, IL-12 p40, IL-13, IL-15, I-309, IP-10, G-CSF, GM-CSF, IFN-gamma, leptin, MCP-1, MCP-2, MCP-3, MDC, MIP-1 alpha, MIP-1 beta, MIP-1 delta, PARC, PDGF, RANTES, SCF, TARC, TGF-beta, TIMP-1, TIMP-2, TNF-alpha, TNF-beta, TPO, and VEGF. Absorbances, which were directly proportional to the concentration of each hormone in the sample, were measured in duplicate at a wavelength of 450 nm using a microplate reader (iMark, Bio-Rad, Hercules, CA, USA). A set of standards of known concentrations of each hormone was utilized to construct a standard curve by plotting the net absorbance values of the standards against the respective protein concentrations. By applying a linear curve using data reduction software (Microplate Manager, Bio-Rad, Hercules, CA, USA), the serum concentrations of each hormone were calculated. The overall intra-assay percent coefficients of variation were 8.2%, 7.1%, and 6.8% for IGF-1, GH, and HGF, respectively.
Skeletal muscle cellular extractionApproximately 20 mg of each muscle sample was weighed and subsequently homogenized using a commercial cell extraction buffer (Biosource, Camarillo, CA, USA) and a tissue homogenizer based on our previous approach (Shelmadine et al., 2009; Spillane et al., 2009; 2014). The cell extraction buffer was supplemented with 1mM phenylmethanesulphonylfluoride [(PMSF), Sigma Aldrich, St. Louis, MO, USA] and a protease inhibitor cocktail (Sigma Aldrich, St. Louis, MO, USA) which possesses broad specificity for the inhibition of serine, cysteine, and metallo-proteases.
Assessment of total DNA, muscle protein, and c-Met contentBased on our previous guidelines (Shelmadine et al., 2009; Spillane et al., 2011; Wilborn et al., 2009), DNA was isolated from muscle samples with 100% ethanol, 0.1 M sodium citrate, and 8 mM sodium hydroxide. The total DNA concentration was determined spectrophotometrically (Smart Spec Plus, Bio-Rad, Hercules, CA, USA) by optical density (OD) at 260 nm using an OD260 equivalent to 50 g//l [Ausubel et al., 1998], and the final concentration expressed relative to muscle wet-weight. In addition, total muscle protein content was determined based on the Bradford method [Bradford, 1976], using a spectrophotometer (xMark, Bio-Rad, Hercules, CA, USA) at a wavelength of 595 nm. Bovine serum albumin was used to generate a standard curve (R2 = 0.991), and total protein content was expressed relative to muscle wet-weight (Shelmadine et al., 2009; Spillane et al., 2011; Wilborn et al., 2009). Muscle homogenate samples were analyzed for total c-Met using a commercially-available ELISA kit (Life Technologies, Grand Island, NY, USA). The sensitivity of this assay is reported to be 0.4 ng·ml-1. Absorbances were measured in duplicate at 450 nm with a microplate reader (xMark, Bio-Rad, Hercules, CA, USA). A set of standards of known concentrations for c-Met were utilized to construct a linear standard curve by plotting the net absorbance values of the standards against their respective protein concentrations (R2 = 0.962). By applying a linear curve using data reduction software (Microplate Manager, Bio-Rad, Hercules, CA, USA), the concentration of muscle c-Met was calculated and expressed relative to total protein content. The overall intra-assay percent coefficient of variation was 7.7%.
Statistical analysisData were analyzed with separate 2 (group) x 2 (time) analysis of variance (ANOVA) using SPSS for Windows Version 20.0 software (SPSS, Chicago, IL). Significant differences among groups were identified by a Tukey HSD post-hoc test. However, to protect against Type I error, the conservative Hunyh-Feldt Epsilon correction factor was used to evaluate observed within-group F-ratios. Resistance volume load was analyzed with separate independent t-tests for upper- and lower-body. For TDEE from Day 0 to 57 within groups with and without ingestion of the supplement, paired t-tests were employed. For TDEE between groups, an independent t-test was utilized. An a-priori power calculation showed that 10 participants per group was adequate to detect a significant difference between groups in the dependent variable of muscle strength and the independent variable of resistance training, given a type I error rate of 0.05 and a power of 0.80. The index of effect size utilized was partial Eta squared (η2), which estimates the proportion of variance in the dependent variable that can be explained by the independent variable. Partial Eta squared effect sizes were determined to be: weak = 0.17, medium = 0.24, strong = 0.51, very strong = 0.70 (O’Connor et al., 2007). For statistical procedures, a probability level of ≤ 0.05 was adopted throughout the study.
Subject demographicsTwenty-four participants began the study; however, two in the HC group and one in the HPC group dropped out due to reasons unrelated to the study. As a result, 21 participants completed the study. The HC group (n = 10) had a mean (±SD) age of 19.38 ± 1.18 yr, height of 1.79 ± 0.06 m, percent fat of 21.86 ± 7.87 %, and total body mass of 86.09 ± 13.50 kg. The HPC group (n = 11) had an age of 21.38 ± 4.07 yr, height of 1.79 ± 0.06 m, percent fat of 17.65 ± 6.43 %, and total body mass of 84.28 ± 12.04 kg.
Dietary analyses and supplement complianceThe completed dietary intake forms were used to analyze the average daily caloric and macronutrient consumption, not including the additional daily calories ingested from the respective supplements, along with calculating TDEE (Table 1). In this case, neither group significantly changed their caloric intake during the course of the study (p > 0.05). In addition, there were no significant group x test interactions indicating there to be no differences between groups for total calories (p = 0.450, η2 = 0.029) or for the intake of protein (p = 0.182, η2 = 0.008), carbohydrate (p = 0.737, η2 = 0.036), fat (p = 0.989, η2 = 0.003), and TDEE (p = 0.341, η2 = 0.022). For HPC and HC without the supplement, there were no significant differences in the percent increase in total caloric intake at Day 57 compared to the TDEE (t = 0.035). However, for total caloric intake including the supplement at Day 57, HPC and HC were significantly different compared to the corresponding TDEE (t = 0.021). In regard to compliance, HC and HPC were 92.32 ± 9.89 % and 94.37 ± 8.17 % compliant to the resistance training program, respectively. For supplementation compliance, HC and HPC were 95.43 ± 4.12 % and 97.52 ± 3.84 % compliant to the supplementation protocol, respectively.
Resistance training compliance and volume load of training studyFour participants were unable to complete every workout session for the eight-week training period. Two participants were only able to complete 93.75% of the total lower-body workouts due to temporary muscular injury. Another participant was also only able to complete 93.75% for both the upper and lower body workout sessions due to temporary illness. In addition, one other participant suffered a minor muscular injury and was only able to complete 93.75% of the total lower-body sessions during the course of training. In regard to training volume load, no significant differences between groups for volume load (defined as reps x sets x weight) in both upper- (p = 0.823) and lower-body (p = 0.774) cumulative exercise sessions. Respective data for the overall volume load for the upper- and lower-body training sessions between groups are presented in Table 2.
Body compositionThere were no significant group x test interactions for total body mass (p = 0.076, η2 = 0.003), fat mass (p = 0.975, η2 = 0.001), or lean mass (p = 0.761, η2 = 0.004). A significant interaction for total body water (p = 0.030, η2 = 0.024) was shown with HPC compared to the HC group. There were significant main effects for time for total body mass (p = 0.001 η2 = 0.153), total body water (p = 0.001, η2 = 0.227) and fat mass (p = 0.001, η2 = 0.160), but not for lean mass (p = 0.068, η2 = 0.007) as a result of resistance training (Table 3).
Muscle performanceThere were no significant group x test interactions for upper-body strength (p = 0.989, η2 = 0.001) and lower-body strength (p = 0.097, η2 = 0.012). However, there were significant main effects for time for both upper- (p = 0.024, η2 = 0.293) and lower-body strength (p = 0.001, η2 = 0.152) as a result of resistance training (Table 4).
Serum IGF-1, GH, and HGFThere were no significant group x test interactions observed for serum IGF-1 (p = 0.060, η2 = 0.139), GH (p = 0.375, η2 = 0.032), or HGF (p = 0.370, η2 = 0.022), indicating there to be no significant differences due to supplementation. In addition, there were no significant main effects for time regard to IGF-1 (p = 0.270, η2 = 0.026), GH (p = 0.397, η2 = 0.029), and HGF (p = 0.070, η2 = 0.094), indicating there to be no significant differences due to resistance training (Table 5).
Total muscle protein and MHC protein isoform contentFor total muscle protein content, there was no significant group x time interaction (p = 0.157, η2 = 0.082) or significant main effect for time (p = 0.101, η2 = 0.108), indicating no beneficial effect from supplementation or resistance training. There were no significant group x time interactions for MHC 1 (p = 0.178, η2 = 0.094 = 0.085), MHC 2A (p = 0.142, η2 = 0.091), and MHC 2X (p = 0.318, η2 = 0.089). However, a significant main effect for time was observed for MHC 1 (p = 0.039, η2 = 0.246) and MHC 2A (p = 0.027, η2 = 0.242), but there was no significant change for MHC 2X (p = 0.351, η2 = 0.094) (Table 6).
Total muscle DNA and cMet contentThere were no significant interactions for total muscle DNA (p = 0.782, η2 = 0.004) or cMet protein concentration (0.477, η2 = 0.108), indicating no beneficial effect from supplementation. For total DNA (p = 0.217, η2 = 0.081) and cMet (p = 0.231, η2 = 0.045) content, there were also no significant main effects for time, indicating no beneficial effect from resistance training (Table 7).
The present study investigated the effect of daily overfeeding with whey protein and/or carbohydrate in conjunction with eight weeks of resistance training on body composition, muscle strength, and markers indicative of MPS and myogenesis in resistance-trained males. While we observed significant increases in muscle strength and MHC 1 and 2A content with resistance training in both groups, we failed to observe any supplement-induced improvements in any of the variables assessed in either group. We also observed no significant differences in total caloric intake, as well as the intake of carbohydrate, fat, and protein in either group during the course of the study. Furthermore, our data provide insight into the issue that, in regard to total daily calories, a greater macronutrient intake relative to one’s TDEE with protein and/or carbohydrate supplementation does not appear to preferentially increase muscle strength and mass. Regarding the fact that the increased protein intake failed to elicit an anabolic/ergogenic effect in response to resistance training, this is most likely due to the “muscle full effect” in which an excessive protein/amino acid load simply overwhelms the muscle in a manner that does not augment increases in muscle mass. In our study, we had two groups of resistance-trained men undergo daily overfeeding with either carbohydrate (HC) or carbohydrate and protein (HPC) while participating in eight weeks of heavy resistance training. Both groups supplemented their daily caloric intake with an additional 312 grams (1,248 kcals of carbohydrate) of maltodextrose in the HC group and 312 grams [1,248 kcals (22 grams of fat, 158 grams of carbohydrate, 94 grams of protein) in the HPC group. For both supplements, half of the total daily dosage (156 g) was ingested 30 minutes prior to each exercise session and half (156 g) was ingested within 30 minutes following each exercise session. As can be seen in Table 1, without either supplement both groups were already ingesting approximately 2,500-2,700 kcals·day-1 at the onset of the study and 2,400-2,500 kcals·day-1 at the end of the study, which was approximately 440-600 kcals above their TDEE. However, the supplementation protocol increased the daily caloric intake of both groups to approximately 3,700-3,800 kcals·day-1, and represented an increase of 1,394 and 1,101 kcals above the total daily caloric intake, respectively, for HPC and HC. In addition, it also represents respective increases in macronutrient intakes of 1,650 and 1,520 kcals beyond the TDEE for HPC and HC. In regard to the fact that we failed to see any superior increases in muscle strength and muscle mass and serum (IGF-1, GH, HGF) and muscle (total muscle protein and MHC isoforms) biochemical indicators of MPS and myogenesis (total DNA and c-Met) in the HPC group compared to HC as a result of the greater daily protein intake, this can likely be explained by two recent studies utilizing egg protein (Moore et al., 2009) and whey protein (Witard et al., 2014). These studies provided the respective protein supplement following a bout of resistance exercise and found 20 grams of protein to be optimal for stimulating MPS, and that ingesting 40 grams of protein was no more effective than 20 grams. Interestingly, both studies found that protein doses greater than 20 grams resulted in an overabundance of available amino acids that subsequently resulted in an increased rate of amino acid oxidation and ureagenesis. Additionally, a recent study (Moore et al., 2012) showed that four, 20- gram doses of whey protein ingested every three hours were superior in increasing MPS than two, 40-gram doses ingested every six hours. In the present study, subjects were ingesting two, 47-gram doses of protein separated by approximately three hours. Based on previous results, the present study generates the notion of the “muscle full effect” originally proposed by Atherton et al. [2010]. In that study, an oral dose of 48 g of whey protein increased MPS between 45 and 90 minutes after ingestion despite increases in the blood level of leucine and EAA up to 240 minutes post ingestion. The authors concluded that an upper limit of amino acid delivery must be achieved before muscles can no longer use amino acids as substrate for MPS, thereby resulting in the amino acids being preferentially diverted toward catabolic processes. As a result, consuming excessive amount of protein and amino acids, which occurred in our present study, conceivably resulted in a diminished return in terms of stimulating MPS, rather favoring amino acid oxidation and ureagenesis. In regard to the fact that we failed to see any superior increases in muscle mass in the HC or HPC group as a result of the higher daily carbohydrate intake, this may be explained on the premise that it has been shown that carbohydrate does not augment exercise-induced protein accretion versus protein alone (Staples et al., 2011). This study specifically showed that insulin is not additive or synergistic to rates of MPS when carbohydrate (50 grams) was co-ingested with 25 grams of whey protein, a protein dose known to maximally stimulate MPS (Moore et al., 2012). It has also been shown that insulin is not an additive factor in stimulating protein/amino acid-induced increases in MPS (Staples et al., 2011). In regards to nutrient excess, this may involve the progressive overwhelming effect of elevated carbohydrate (and concomitant insulin levels) decreasing AMP-activated protein kinase (AMPK) activity in muscle (Coughlan et al., 2015). In the event that high levels of carbohydrate suppress muscle growth processes, it may be related to decreased AMPK activation (similar to that seen with insulin resistance). In our present study, participants ingested two 79-gram doses of carbohydrate separated by approximately three hours. In the Atherton study (2010), with a 48-gram dose of protein, they observed a 35 mU/L insulin peak at approximately 60 minutes post-exercise. In the present study, assuming our participants were not glycogen depleted prior to each resistance exercise session (and they should not have been based on their dietary intakes), the exercise alone likely would not have degraded enough muscle glycogen to predicate the need for all of the glucose being ingested being cleared by muscle and utilized; therefore, the activity of hexokinase would most likely be inhibited and AMPK activated, thereby minimizing glucose uptake (Gauthier et al., 2011). Chronic overfeeding typically leads to hypertrophy of adipocytes and the development of a chronic sub-clinical, pro-inflammatory environment (Glass and Olefshy, 2012). However, it has recently been shown that eight weeks of resistance training combined with consuming a high protein diet of 4.4 g·kg-1·day-1 did not increase total body mass, fat mass, or lean mass compared to a control protein intake of 1.8 g·kg-1·day-1 (Antonio et al., 2014). This study indicates that the daily caloric excess from consuming 5.5 times the recommended dietary allowance for protein had no apparent effect on de novo lipogenesis, nor did it have any preferential effect on increasing MPS. Although, we observed that daily overfeeding with protein and/or carbohydrate resulted in significant increases in total body mass and fat mass, without a corresponding increase in fat-free mass. It is not unreasonable to assume that the increased glycemic load from the supplementation protocol attributed to the accumulation of fat mass in both groups over the course of the study. With supplementation, the daily carbohydrate intake of the HC and HPC groups was 68% and 48%, respectively, above the reported daily carbohydrate intake. In light of this, our data indicate that chronic overfeeding appears to be associated with de novo lipogenesis and subsequent triglyceride synthesis since the increased influx of glucose provides substrate for triglyceride syn-thesis in adipocytes (Bijland et al., 2013).
LimitationsIn view of the results presented herein, our study does possess three possible limitations. One limitation may be the sample size. While a sample size of 21 is somewhat small, indeed it is notably larger than many other studies in the literature employing a very similar experimental design. We did perform an a-priori power analysis; therefore, our study should be adequately powered. The second limitation is the issue of only using four-day dietary recalls for determining nutritional intakes prior to each of the two testing sessions at Day 0 and 57. Second, it is possible that the information provided from the dietary intakes was not reflective of the nutritional intakes over the course of the study. Thirdly, supplement compliance is a potential limitation. Even though participants returned the empty containers and self- reported their compliance, it is possible that the information provided to study personnel was not reflective of the actual supplement compliance. Despite our confidence in the reliability and validity of our data, in lieu of these limitations, the results presented herein should be interpreted with some amount of caution.
In light of our results presented herein, we conclude that daily overfeeding with protein and/or carbohydrate in conjunction with resistance training for eight weeks does not improve body composition and muscle performance or increase the concentration of markers indicative of MPS and myogenic activation in resistance-trained men.
ACKNOWLEDGEMENTS |
Funding for this study was obtained from an independent research grant awarded to Baylor University from Vital Pharmaceuticals, Inc. (Weston, FL, USA). Researchers involved in collecting data in this study have no financial or personal interest in the outcome of results or the sponsor. |
|
AUTHOR BIOGRAPHY |
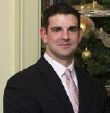 |
Mike Spillane |
Employment: Assistant Professor of Exercise Physiology in the Department of Health, Physical Education, and Leisure Studies at the University of South Alabama. |
Degree: PhD |
Research interests: muscle physiology and exercise nutrition. |
E-mail: micheilspillane@southalabma.edu |
|
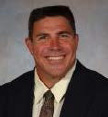 |
Darryn S. Willoughby |
Employment: Associate Professor of Exercise and Nutritional Biochemistry and Molecular Physiology in the Department of Health, Human Performance, & Recreation at Baylor University |
Degree: PhD |
Research interests: biochemical and molecular regulatory mechanisms regarding exercise performance and nutrition. |
E-mail: darryn_willoughby@baylor.edu |
|
|
|
REFERENCES |
 Akiyama T., Tachibana I., Shirohara H., Watanabe N., Otsuki M. (1996) High-fat hypercaloric diet induces obesity, glucose intolerance and hyperlipidemia in normal adult male Wistar rat. Diabetes Research and Clinical Practice 31, 27-35.
|
 Antonio J., Peacock C.A., Ellerbroek A., Fromhoff B., Silver T. (2014) The effects of consuming a high protein diet (4.4 g/kg/d) on body composition in resistance-trained individuals. Journal of the International Society of Sports Nutrition 11, 19-.
|
 Areta J.L., Burke L.M., Ross M.L., Camera D.M., West D.W., Broad E.M., Jeacocke N.A., Moore D.R., Stellingwerff T., Phillips S.M., Hawley J.A., Coffey V.G. (2013) Timing and distribution of protein ingestion during prolonged recovery from resistance exercise alters myofibrillar protein synthesis. Journal of Physiology 591, 2319-2331.
|
 Atherton P.J., Etheridge T., Watt P.W., Wilkinson D., Selby A., Rankin D., Smith K., Rennie M.J. (2010) Muscle full effect after oral protein: time-dependent concordance and discordance between human muscle protein synthesis and mTORC1 signaling. The American Journal of Clinical Nutrition 92, 1080-1088.
|
 Ausubel F.M. (1998) . Current Protocols in Molecular Biology. John Wiley & Sons.
|
 Bijland S., Mancini S.J., Salt I.P. (2013) Role of AMP-activated protein kinase in adipose tissue metabolism and inflammation. Clinical Science 124, 491-507.
|
 Biolo G., Maggi S.P., Williams B.D., Tipton K.D., Wolfe R.R. (1995) Increased rates of muscle protein turnover and amino acid transport after resistance exercise in humans. American Journal of Physiology 268, E514-520.
|
 Bird S.P., Tarpenning K.M., Marino F.E. (2006) Liquid carbohydrate/essential amino acid ingestion during a short-term bout of resistance exercise suppresses myofibrillar protein degradation. Metabolism 55, 570-577.
|
 Bohe J., Low J.F.A, Wolfe R.R., Rennie M.J. (2001) Latency and duration of stimulation of human muscle protein synthesis during continuous infusion of amino acids. Journal of Physiology 532, 575-579.
|
 Bohe J., Low J.F.A, Wolfe R.R., Rennie M.J. (2003) Human muscle protein synthesis is modulated by extracellular, not intramuscular amino acid availability: a dose-response study. Journal of Physiology 552, 315-324.
|
 Bradford M.M. (1976) A rapid and sensitive method for the quantitation of microgram quantities of protein utilizing the principle of protein-dye binding. Analytical Biochemistry 72, 248-254.
|
 Coughlan K.A., Balon T.W., Valentine R.J., Petrocelli R., Schultz V., Brandon A., Cooney G.F., Kraegen E.W., Ruderman N.B., Saha A.K. (2015) Nutrient excess and AMPK downregulation in incubated skeletal muscle and muscle of glucose infused rats. PLOS ONE 21, 10-.
|
 Ferguson-Stegall L., McCleave E.L., Ding Z., Doerner P.G., Wang B., Liao Y.H., Kammer L., Liu Y., Hwang J., Dessard B.M., Ivy J.L. (2011) Postexercise carbohydrate-protein supplementation improves subsequent exercise performance and intracellular signaling for protein synthesis. Journal of Strength and Conditioning Research 25, 1210-1224.
|
 Gauthier M.S., O’Brien E. L., Bigornia S., Mott M., Caciedo J.M., Xu X.J., Gokce N., Apovian C., Ruderman N. (2011) Decreased AMP-activated protein kinase activity is associated with increased inflammation in visceral adipose tissue and with whole-body insulin resistance in morbidly obese humans. Biochemical and Biophysical Research Communications 404, 382-387.
|
 Glass C.K., Olefsky J.M. (2012) Inflammation and lipid signaling in the etiology of insulin resistance. Cell Metabolism 15, 635-645.
|
 Haaf T.T., Weijs P.J.M. (2014) Resting energy expenditure prediction in recreational athletes of 18-35 years: confirmation of Cunningham equation and an improved weight-based alternative. PLOS ONE 9, 1-8.
|
 Hill J.O., Peters J.C., Reed G.W., Schlundt D.G., Sharp T., Greene H.L. (1991) Nutrient balance in humans: effects of diet composition. American Journal of Clinical Nutrition 54, 10-17.
|
 Moore D.R., Areta J., Coffey V.G., Stellingwerff T., Phillips S.M., Burke L.M., Cleroux M., Godin J.P., Hawley J. A. (2012) Daytime pattern of post-exercise protein intake affects whole-body protein turnover in resistance-trained males. Nutrition and Metabolism (Lond) 9, 91-.
|
 Moore D.R., Robinson M.J., Fry J.L., Tang J.E., Glover E.I., Wilkinson S.B., Prior T., Tarnopolsky M.A., Phillips S. M. (2009) Ingested protein dose response of muscle and albumin protein synthesis after resistance exercise in young men. The American Journal of Clinical Nutrition 89, 161-168.
|
 O’Connor K., Stip E., Pelissier M. C., Aardema F., Guay S., Gaudette G., Van Jaaster I., Robillard S., Grenier S., Careau Y, Doucet P., Leblanc V. (2007) Treating delusional disorder: a comparison of cognitive-behavioural therapy and attention placebo control. Canadian Journal of Psychiatry 52, 182-190.
|
 Phillips S. M., Tipton K. D., Aarsland A., Wolf S. E., Wolfe R. R. (1997) Mixed muscle protein synthesis and breakdown after resistance exercise in humans. American Journal of Physiology 273, E99-107.
|
 Rahbek S.K., Farup J., Moller A.B., Vendelbo M.H., Holm L., Jessen N., Vissing K. (2014) Effects of divergent resistance exercise contraction mode and dietary supplementation type on anabolic signalling, muscle protein synthesis and muscle hypertrophy. Amino Acids 46, 2377-2392.
|
 Rennie M. J., Bohé J., Wolfe R. R. (2002) Latency, duration and dose response relationships of amino acid effects on human muscle protein synthesis. The Journal of Nutrition 132, 3225S-3227S.
|
 Samocha-Bonet D., Campbell L. V., Mori T. A., Croft K. D., Greenfield J. R., Turner N., Heilbronn L. K. (2012) Overfeeding reduces insulin sensitivity and increases oxidative stress, without altering markers of mitochondrial content and function in humans. PLOS ONE 7, e36320-.
|
 Shelmadine B., Cooke M., Buford T., Hudson G., Redd L., Leutholtz B., Willoughby D.S. (2009) Effects of 28 days of resistance exercise and consuming a commercially available pre-workout supplement, NO-Shotgun(R), on body composition, muscle strength and mass, markers of satellite cell activation, and clinical safety markers in males. Journal of the International Society of Sports Nutrition 6, 16-.
|
 Spillane M., Emerson C., Willoughby D. S. (2012) The effects of 8 weeks of heavy resistance training and branched-chain amino acid supplementation on body composition and muscle performance. Nutrition and Health 21, 263-273.
|
 Spillane M., Schoch R., Cooke M., Harvey T., Greenwood M., Kreider R., Willoughby D. S. (2009) The effects of creatine ethyl ester supplementation combined with heavy resistance training on body composition, muscle performance, and serum and muscle creatine levels. Journal of the International Society of Sports Nutrition 6, 6-.
|
 Spillane M., Schwarz N., Leddy S., Correa T., Minter M., Longoria V., Willoughby D.S. (2011) Effects of 28 days of resistance exercise while consuming commercially available pre- and post-workout supplements, NO-Shotgun(R) and NO-Synthesize(R) on body composition, muscle strength and mass, markers of protein synthesis, and clinical safety markers in males. Nutrition and Metabolism (Lond) 8, 78-.
|
 Spillane M., Schwarz N., Willoughby D.S. (2014) Heavy resistance training and peri-exercise ingestion of a multi-ingredient ergogenic nutritional supplement in males: effects on body composition, muscle performance and markers of muscle protein synthesis. Journal of Sports Science and Medicine 13, 894-903.
|
 Staples A.W., Burd N.A., West D.W., Currie K.D., Atherton P.J., Moore D.R., Rennie M.J., Macdonald M.J., Baker S.K., Phillips S. M. (2011) Carbohydrate does not augment exercise-induced protein accretion versus protein alone. Medicine and Science in Sports and Exercise 43, 1154-1161.
|
 Thyfault J.P., Carper M.J., Richmond S.R., Hulver M.W., Potteiger J.A. (2004) Effects of liquid carbohydrate ingestion on markers of anabolism following high-intensity resistance exercise. Journal of Strength and Conditioning Research 18, 174-179.
|
 Vienberg S.G., Bjornholm M. (2014) Chronic glucocorticoid treatment increases de novo lipogenesis in visceral adipose tissue. Acta Physiologica (Oxford, England), 211, 257-259.
|
 Wilborn C.D., Taylor L.W., Greenwood M., Kreider R.B., Willoughby D.S. (2009) Effects of different intensities of resistance exercise on regulators of myogenesis. Journal of Strength and Conditioning Research 23, 2179-2187.
|
 Willoughby D., Stout J., Wilborn C. (2007) Effects of resistance training and protein plus amino acid supplementation on muscle anabolism, mass, and strength. Amino Acids 32, 467-477.
|
 Witard O.C., Jackman S.R., Breen L., Smith K., Selby A., Tipton K.D. (2014) Myofibrillar muscle protein synthesis rates subsequent to a meal in response to increasing doses of whey protein at rest and after resistance exercise. American Journal of Clinical Nutrition 99, 86-95.
|
|
|
|
|
|
|